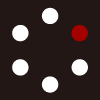
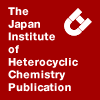
HETEROCYCLES
An International Journal for Reviews and Communications in Heterocyclic ChemistryWeb Edition ISSN: 1881-0942
Published online by The Japan Institute of Heterocyclic Chemistry
e-Journal
Full Text HTML
Received, 19th June, 2009, Accepted, 7th August, 2009, Published online, 10th August, 2009.
DOI: 10.3987/COM-09-11779
■ A Three-Dimensionally Oriented π-Conjugated System: Synthesis of the Porphyrins Bearing Terthiophene Pendant Strands
Toru Amaya, Koichi Mori, and Toshikazu Hirao*
Department of Applied Chemistry, Graduate School of Science, Osaka University, 2-1 Yamadaoka, Suita, Osaka 560-0871, Japan
Abstract
The porphyrins bearing three-dimensionally oriented terthiophene pendant strands were synthesized to elucidate the conformational behaviour.Architecturally ordered regulation of π-conjugated molecular chains on a functionalized scaffold is considered to provide efficient and functional nanostructured catalysts and materials. In this context, the introduction of π-conjugated chains into a porphyrin as a scaffold is expected to afford such a π-conjugated system. Covalently connected porphyrins through the π-pyrrole moieties or meso-positions have been designed for two-dimensional π-electron systems.1 Atropisomerism of meso-tetrakis(o-substituted aryl)porphyrins is considered to have an advantage of aligning pendant strands on the porphyrin plane under dimensional control. We have focused on the redox-active oligoaniline chains, which were demonstrated to be incorporated into a porphyrin2 as well as ruthenium bipyridyl3 and calix[4]arene scaffolds4 to construct the dimensionally oriented π-conjugated systems.5 In our porphyrin-oligoaniline systems, as represented by the porphyrin bearing the four aniline trimer pendant strands, the photo-induced electron transfer from the phenylenediamine pendant groups to the porphyrin scaffold is indicated under excitation of the porphyrin.2 In the present study, the oligothiophene-porphyrin compounds with one or two terthiophene pendant strands were designed. As is well known, oligothiophene is one of the most attractive π-conjugated molecules with redox activity, which are applicable for electrical materials such as organic field effect transistors and light emitting diodes.6 During our investigation,7 the dimensional controlled porphyrins bearing oligothiophene chains were synthesized.8 We herein describe the synthesis of the porphyrins 1cis, 1trans, and 2 bearing dimensionally aligned terthiophene chains for the construction of three-dimensionally oriented π-conjugated systems (Figure 1).
Scheme 1 shows the synthesis of the above-mentioned oligothiophene-porphyrins 1cis, 1trans, and 2. These were prepared by the nucleophilic ether formation reaction of the corresponding isolated atropisomeric phenols 3cis,9 3trans,10 and 411 with the bromide 5 bearing the terthiophene moiety under the basic conditions in 55, 56, and 50% yields, respectively.12 1H NMR studies suggest the structures of the porphyrins 1cis, 1trans, and 2. The chemical shifts of the pendant moiety of 1cis, 1trans, and 2, and the model terthiophene compound 6 are summarized in Table 1. The protons of the terthiophene units, especially the thiophene protons close to the porphyrin ring, were observed in a higher field. The Δδ(Ha) values between the porphyrins 1cis, 1trans, and 2, and the model terthiophene compound 6 reach more than 5 ppm, and even the Δδ(Hb) values show 2.3-2.4 ppm. The shift is explained by the ring-current effect of the porphyrin π-system. Thus, 1cis, 1trans, and 2 take a leaned conformation of the terthiophene pendant strands toward the porphyrin scaffold in solution. The terthiophene chains are stably positioned on the porphyrin ring.
The electronic environment of the π-conjugated systems 1cis, 1trans, and 2 was investigated by UV–vis spectroscopy. The spectra are shown in Figure 2a. Typical Soret and Q bands were observed in these porphyrin derivatives. The molar coefficient of the Soret band of 1cis was lower than those of the atropisomer 1trans and the single strand derivative 2, which might be accounted for by the interaction and/or the small distortion and fluctuation of the porphyrin ring due to the terthiophene moiety, whose absorption was observed around 350 to 450 nm as a broad peak. The fluorescence emission spectroscopy with excitation of the Qy(1,0) band was studied (Figure 2b). The significant quenching was observed with 1cis and 1trans bearing the two terthiophene pendant strands. The single strand derivative 2 showed the emission with ca. 30% intensity as compared with that of tetraphenylporphyrin (TPP). However, no quenching occurred with the mixture of TPP and 6, even in the presence of 10 equivalents of 6. These findings suggest the dimensionally oriented π-conjugated systems play an important role in the intramolecular photo-induced behaviour.
In conclusion, the porphyrins bearing the dimensionally oriented terthiophene pendant strands were synthesized. These π-conjugated systems are of potential use in a variety of applications such as redox-active receptors and photo-active catalysts or materials. Further investigation is now in progress.
ACKNOWLEDGEMENTS
The authors thank Mrs. Toshiko Muneishi at Osaka University for the measurement of the NMR spectra.
References
1. (a) V. S.-Y. Lin, S. G. DiMagno, and M. J. Therien, Science, 1994, 264, 1105; CrossRef (b) F. Würthner, M. S. Vollmer, F. Effenberger, P. Emele, D. U. Meyer, H. Port, and H. C. Wolf, J. Am. Chem. Soc., 1995, 117, 8090; CrossRef (c) S. Mikami, K. Sugiura, and Y. Sakata, Chem. Lett., 1997, 26, 833; CrossRef (d) N. Ono, H. Miyagawa, T. Ueta, T. Ogawa, and H. Tani, J. Chem. Soc., Perkin Trans. 1, 1998, 1595; CrossRef (e) A. Tsuda and A. Osuka, Scinece, 2001, 293, 79; CrossRef (f) G. J. Capitosti, C. D. Guerrero, D. E. Binkley, Jr., C. S. Rajesh, and D. A. Modarelli, J. Org. Chem., 2003, 68, 247; CrossRef (g) B. Li, J. Li, Y. Fu, and Z. Bo, J. Am. Chem. Soc., 2004, 126, 3430; CrossRef (h) T. Ishi-i, Y. Taguri, S. Kato, M. Shigeiwa, H. Gorohmaru, S. Maeda, and S. Mataka, J. Mater. Chem., 2007, 17, 3341; CrossRef (i) H. Yamada, D. Kuzuhara, T. Takahashi, Y. Shimizu, K. Uota, T. Okujima, H. Uno, and N. Ono, Org. Lett., 2008, 10, 2947; CrossRef (j) J. Song, S. Y. Jang, S. Yamaguchi, J. Sankar, S. Hiroto, N. Aratani, J.-Y. Shin, S. Easwaramoorthi, K. S. Kim, D. Kim, H. Shinokubo, and A. Osuka, Angew. Chem. Int. Ed., 2008, 47, 6004. CrossRef
2. (a) T. Hirao and K. Saito, Tetrahedron Lett., 2000, 41, 1413; CrossRef (b) K. Saito and T. Hirao, Tetrahedron, 2002, 58, 7491; CrossRef (c) T. Hirao and K. Saito, Synlett, 2002, 415. CrossRef
3. (a) T. Hirao and K. Iida, Chem. Commun., 2001, 431; CrossRef (b) X. Shen, T. Moriuchi, and T. Hirao, Tetrahedron Lett., 2003, 44, 7711. CrossRef
4. K. Saito and T. Hirao, Bull. Chem. Soc. Jpn., 2002, 75, 1845. CrossRef
5. (a) T. Hirao, Coord. Chem. Rev., 2002, 226, 81; CrossRef (b) T. Hirao and K. Saito, Macromol. Symp., 2003, 204,103; CrossRef (c) T. Moriuchi and T. Hirao, ‘Redox Systems Under Nano-Space Control,’ ed. by T. Hirao, Springer, Heidelberg, 2006, pp 3-27. CrossRef
6. For reviews, see: (a) T. Otsubo, Y. Aso, and K. Takimiya, J. Mater. Chem., 2002, 12, 2565; CrossRef (b) G. Barbarella, M. Melucci, G. Sotgiu, Adv. Mater., 2005, 17, 1581; CrossRef (c) A. R. Murphy and J. M. J. Frchet, Chem. Rev., 2007, 107, 1066. CrossRef
7. T. Hirao, K. Mori, H.-L. Wu, and T. Amaya, The Tenth International Kyoto Conference on New Aspects of Organic Chemistry (IKCOC-10), Kyoto, Japan, Nov. 14, 2006 (abstract: pp 87).
8. K. Sugiyasu and M. Takeuchi, Chem. Eur. J., 2009, 15, 6350. CrossRef
9. The 1H NMR spectrum of 3cis is identical to the reported one, see: T. Hayashi, T. Miyahara, N. Koide, Y. Kato, H. Matsuda, and H. Ogoshi, J. Am. Chem. Soc., 1997, 119, 7281. CrossRef
10. 3trans was separated from a mixture of cis and trans isomers according to the reference 9.
11. The porphyrin skeleton for 4 was constructed according to a related procedure described in the reference 9 based on a BF3·Et2O-catalyzed condensation of the corresponding benzaldehyde and 2-methoxy-1-naphthaldehyde with pyrrole, followed by oxidation with 2,3-dichloro-5,6-dicyano-1,4-benzoquinone. The deprotection of the methyl group with BBr3 gave 4.
12. Synthetic procedure for 1cis as a typical one, melting points, 1H and 13C NMR, and high resolution mass values for 1cis, 1trans, 2: To a DMF solution of 3cis (26 mg, 0.035 mmol), 5 (65 mg, 0.138 mmol), and K2CO3 (48 mg, 0.35 mmol) were added two portions of Bu4NI under argon at room temperature. After stirring for 24 h at room temperature, the reaction mixture was poured into a solution of Et2O and water. The aqueous layer was extracted with Et2O twice. The combined organic layer was washed with water, brine, dried over MgSO4, and evaporated in vacuo. The crude residue was purified by silica-gel column chromatography to give 1cis as a purple solid (28 mg, 0.018 mmol, 53% yield). 1cis : Mp 155 °C; 1H NMR (600 MHz, CD2Cl2) δ 8.81 (d, J = 4.2 Hz, 4H), 8.68 (d, J = 4.2 Hz, 4H), 8.45 (d, J = 8.4 Hz, 2H), 8.23 (d, J = 8.4 Hz, 2H), 8.19 (m, 2H), 7.83 (d, J = 7.2 Hz, 2H), 7.70 (m, 4H), 7.60 (d, J = 9.0 Hz, 4H), 7.55 (dd, J = 7.8, 7.8 Hz, 4H), 7.33 (dd, J = 7.8, 7.8 Hz, 2H), 6.83 (d, J = 3.0 Hz, 2H), 6.67 (d, J =3.6 Hz, 2H), 6.66 (d, J = 3.6 Hz, 2H), 5.94 (d, J = 3.6 Hz, 2H), 5.32 (bs, 2H), 4.52 (d, J = 3.6Hz, 2H), 4.41 (s, 4H), 2.79 (t, J = 7.2 Hz, 4H), 1.67 (tt, J = 7.2, 7.2 Hz, 4H), 1.48 (d, J = 3.6 Hz, 4H), 1.35-1.32 (m, 12H), 0.93-0.90 (m, 6H), -2.21 (bs, 2H); 13C NMR (150 MHz, CD2Cl2) 162.98, 155.74, 145.85, 141.68, 137.44, 135.70, 134.89, 134.72, 134.47, 132.81, 131.85, 129.85, 128.23, 127.89, 127.72, 127.22, 125.58, 125.17, 124.93, 123.55, 123.39, 122.89, 121.27, 118.56, 114.44, 113.30, 111.25, 68.03, 31.97, 30.48, 30.07, 29.14, 22.98, 14.27 ppm; HRMS(FAB): Calcd for C92H76N6O4S6 1520.4252; Found 1520.4249 (M)+. 1trans : Mp 148 °C; 1H NMR (600 MHz, CD2Cl2) δ 8.78 (d, J =4.8 Hz, 4H), 8.66 (d, 4.8 Hz, 4H), 8.47 (d, J = 9.0 Hz, 2H), 8.22 (d, J = 8.4 Hz, 2H,), 8.00 (d, J = 6.6 Hz, 4H,), 7.71 (d, J = 9.0 Hz, 2H), 7.69 (d, J = 7.8 Hz, 2H), 7.63 (dd, 7.2. 7.2 Hz, 4H), 7.52 (dd, J = 7.2, 7.2 Hz, 2H), 7.37 (d, J = 8.4 Hz, 2H), 7.27 (d, J = 7.8 Hz, 2H), 6.77 (d, J = 3.0 Hz, 2H), 6.72 (d, J = 3.6 Hz, 2H), 6.63 (d, J = 3.6 Hz, 2H), 6.29 (d, J = 3.6 Hz, 2H), 5.83 (bs, 2H), 4.68 (s, 4H), 4.63 (d, J = 4.2 Hz, 2H), 2.76 (t, J = 7.8 Hz, 4H), 1.67 (tt, J = 7.2. 7.2 Hz, 4H), 1.54 (m, 2H), 1.32-1.30 (m, 12H), 0.91-0.87 (m, 6H), -2.29 (bs, 2H); 13C NMR (150 MHz, CD2Cl2) 162.84, 155.58, 145.26, 140.99, 136.65, 135.39, 134.68, 134.23, 134.04, 133.87, 131.21, 129.32, 127.60, 127.56, 127.17, 127.12, 126.93, 126.52, 126.41, 125.49, 124.49, 124.36, 123.19, 122.99, 122.88, 122.78, 122.36, 120.86, 120.19, 117.88, 114.86, 112.41, 110.11, 103.43, 50.10, 31.27, 31.23, 29.76, 28.41, 22.26, 13.51 ppm; MS(MALDI-TOF): 1522 (M)+. 2 : Mp: 181 °C; 1H NMR (600 MHz, CD2Cl2) δ: 8.91 (d, J = 4.8 Hz, 2H), 8.85 (d, J = 4.2 Hz, 2H), 8.78 (d, J = 4.8 Hz, 2H), 8.59 (d, J = 4.2 Hz, 2H), 8.44 (d, J = 9.6 Hz, 1H), 8.29 (dd, J = 9.0, 9.0 Hz, 2H), 8.21 (d, J = 6.6 Hz, 2H), 8.19 (d, J = 8.4 Hz, 1H), 8.01 (d, J = 6.6 Hz, 2H), 7.84-7.80 (m, 3H), 7.76-7.72 (m, 4H), 7.70-7.68 (m, 3H), 7.50 (dd, J = 7.8, 7.8 Hz, 1H), 7.30 (d, J = 8.4 Hz, 1H), 7.22 (dd, J = 8.4 Hz, 1H), 6.86 (d, J = 4.2 Hz, 1H), 6.77 (d, J = 3.6 Hz, 1H), 6.66 (d, J = 3.6 Hz, 1H), 6.30 (d, J = 3.6 Hz, 1H), 5.74 (bs, 1H), 4.65 (s, 2H), 4.49 (d, J = 3.6 Hz, 1H), 2.78 (t, J = 8.4 Hz, 2H), 1.66 (tt, J = 7.2, 7.2 Hz, 2H), 1.36 (d, J = 3.6 Hz, 1H), 1.33-1.31 (m, 6H), 0.90 (t, J = 6.6 Hz, 3H), -2.58 (bs, 2H); 13C NMR (150 MHz, CD2Cl2) δ: 162.87, 141.37, 136.78, 134.32, 134.22, 134.04, 131.06, 129.30, 127.53, 127.42, 127.20, 126.85, 126.44, 126.35, 125.73, 124.46, 124.30, 122.84, 122.69, 122.33, 120.82, 120.16, 117.98, 114.73, 110.18, 68.34, 50.11, 45.91, 43.70, 31.25, 29.74, 28.39, 22.25, 19.42, 13.51 ppm; HRMS(FAB): Calcd for C68H53N5O2S3 1067.3361; Found 1067.3357 (M)+.