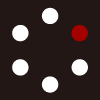
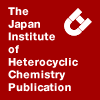
HETEROCYCLES
An International Journal for Reviews and Communications in Heterocyclic ChemistryWeb Edition ISSN: 1881-0942
Published online by The Japan Institute of Heterocyclic Chemistry
e-Journal
Full Text HTML
Received, 26th June, 2009, Accepted, 31st August, 2009, Published online, 31st August, 2009.
DOI: 10.3987/COM-09-S(S)42
■ Rhodium-Catalyzed 1,2-Addition of Sb-Phenyl-1,5-azastibocines to Functionalized Aldehydes
Naoki Kakusawa, Shuji Yasuike, and Jyoji Kurita*
Faculty of Pharmaceutical Sciences, Hokuriku University, 3-Ho, Kanagawa machi, Kanazawa 920-1181, Japan
Abstract
Simple and efficient addition of a phenyl group to aldehydes was accomplished by the rhodium-catalyzed reaction of Sb-phenyl-1,5-azastibocines. Because of the soft nucleophilic character of 1,5-azastibocines, arylation of functionalized aldehydes having ketone, ester, and halogen moieties can be achieved to afford aryl alcohols. The reaction can be carried out under aerobic conditions, in striking contrast to the reactions with hard nucleophiles such as organolithium and Grignard reagents.Chemoselective addition of aryl anion equivalent to mutifunctionalized carbonyl compounds is one of the most challenging reactions in organic synthesis, because the direct formation of aryl alcohols is expected by nucleophilic addition in a single step.1 For arylation of unstable carbonyl compounds with a complex structure, nucleophiles must be compatible with various functional groups, such as aldehydes, ketones, esters, nitriles, enones, and halogens. Application of hard organometallic reagents, including organolithiums and Grignard reagents, will be limited due to their strong nucleophilicity and basicity, which often bring about undesirable side reactions, such as metallation of other susceptible functional groups and decomposition of labile target molecules.2 Development of soft nucleophiles is desired, by which arylation of only specific carbonyl groups will become possible without a tedious protection-deprotection procedure.3
According to Pauling’s electron negativity,4 the carbon atom of the Sb-C bond is slightly negatively charged, so organoantimony compounds exhibit soft nucleophilic characteristics. For example, pentaarylstiboranes, one of the most reactive hypervalent organoantimony compounds, can react with various acyl chlorides and aldehydes which are activated with Lewis acids. In this reaction, aryl groups on pentaarylstiborane are considered to behave as aryl anion equivalents.5 Taking advantage of this soft nucleophilic property of organoantimony compounds, we have previously reported 1,4-addition of Sb-aryl-1,5-azastibocines to electron-deficient olefins under a rhodium catalyst.6 The result implies that not only the aryl group on the 1,5-azastibocine furnishes soft nucleophilic properties, but also the antimony atom bears highly reactive hypervalent characteristics. Indeed, the antimony atom in 1,5-azstibocine exhibits tetravalent psuedo-trigonal bipyramidal structure with intramolecular coordination between antimony and nitrogen, as shown compound 1 in Table 1 and 2.7 As a part of our continuous study on organoantimony compounds as useful organic reagents,8 we next planned the 1,2-addition of 1,5-azastibocines to carbonyl compounds.
Researches on 1,2-addition using main group compounds to aldehydes have been flourishing recently. 9 Organo-boron,10 -aluminum,11 -stannum,12 and -silicon13 compounds have been reacted with aldehyde in the presence of transition metal catalysts, such as rhodium and palladium, to afford aryl alcohols. These main group compounds are generally stable in air and water. The high stability and reactivity of main group compounds under ambient conditions could possibly provide a straightforward method for 1,2-addition of aldehydes and might be a promising alternative for organolithium and Grignard reagents which require strict anhydrous conditions along with an inert atmosphere.
The reaction of Sb-phenyl-1,5-azastibocine (1) with benzaldehyde (2a) was chosen as the model reaction to investigate suitable reaction conditions. The mixture was refluxed in 1,2-DCE (dichloroethane) under aerobic conditions without a transition metal catalyst, but no reaction occurred (Table 1, entry 1). However, we found that rhodium catalysts, especially [RhCl(cod)]2, promoted the 1,2-addition to give the expected benzhydrol (3a) in 70% yield in an atmospheric environment (entry 9) and that similar reactions using nickel and palladium catalysts gave no product (entries 2-6).
The effect of phosphine ligand was investigated to facilitate the arylation of 2a. The addition of 5 mol% of triphenylphosphine (TPP) improved the yield of 3a to 84% yield (entry 11). In contrast, the yield of 3a was decreased by the further addition of TPP (10 mol%) and the reaction was completely inhibited by excess addition of TPP (20 mol%) (entries 12, 13). As Ueda and Miyaura have reported, the electron-donating phosphine ligand is considered to enhance the nucleophilicity of the aryl group on a rhodium catalyst; however, excess phosphine ligand may block the aldehyde to coordinate with rhodium metal.14 Thus, addition of an appropriate amount of phosphine ligand (5 mol%) might keep the balance between the high nucleophilicity of the aryl group and effective coordination of aldehydes to the rhodium catalyst. Electron-rich monodentate phosphines, such as tris(methoxyphenyl)phosphines and tri(n-butyl)phosphine, were excellent ligands for the present reaction, but bulky phosphines and bidentate phosphines with a large bite angle were not effective (entries 14−21).
We also screened suitable solvents under similar reaction conditions using Ph3P (5 mol%) as the phosphine ligand. Polar solvents, such as NMP (N-methyl-2-pyrrolidinone) and DMF, were the preferred solvents in the 1,4-conjugate addition of 1,5-azastibocines with electron-deficient olefins;6 however, they were unsuitable for the present 1,2-addition, e.g., NMP (5 h, 33%) and DMF (9 h, 0%). In hydrocarbon solvents, such as benzene (7 h, 56%) and hexane (7 h, 39%), 3a was formed in moderate yield. The arylation proceeded smoothly in ethereal solvents such as 1,2-DME (7 h, 78%), 1,4-dioxane (4 h, 68%). Consequently, the best result was obtained when 1,2-DCE was employed (5 h, 84%) as a solvent. However, other halogen solvents, such as chloroform (5 h, 40%) and carbon tetrachloride (5 h, 2%), gave 3a in modest yield and the major product was Sb-chloro-1,5-azastibocine, probably derived from homolytic cleavage of the Sb-phenyl bond of 1,5-azastibocine, followed by chlorine abstraction from chloroform and carbon tetrachloride.
Under the optimized conditions examined here,15 we next examined the reaction of 1 with various aromatic (2a−h) and aliphatic aldehydes (2i, j) to reveal the generality and electronic effect of the present reaction. Electronically neutral and deficient aromatic aldehydes (2a−e) underwent 1,2-addition efficiently to afford benzhydrols (3a−e) in excellent yield (Table 2, entries 1−5). Even electron rich and sterically hindered o-substituted aldehydes (2f−h) gave the corresponding 1,2-adducts (3f−h) in satisfactory yield (entries 6−8). When the aryl bromide was treated with phenyllithium or phenylmagnesium bromide, a halogen-metal exchange reaction could occur; however, the reaction of 1,5-azastibocine with p-bromobenzaldehyde (2e) gave the 1,2-adduct (3e) with the halogen moiety unchanged (entry 5). Ketone and ester moieties tolerated the reaction conditions, so no care was needed to protect these carbonyl groups in the formal nucleophilic addition of aryl anion equivalent to aldehyde (entries 2, 3). Aliphatic aldehydes (2i, j) were moderately reactive substrates and the reaction required a prolonged reaction time; however, it is noteworthy that no aldol product was observed even in the presence of α-hydrogen in the present arylation.
These results suggest that Sb-aryl-1,5-azastibocine can behave as a soft nucleophile which reacts only with aldehyde with high functional compatibility. The catalytic cycle of arylation with 1,5-azastibocine might be similar to that with other main group compounds10, 16 and the details of the mechanism and compatibility with other functional groups will be discussed in due course.
ACKNOWLEDGEMENTS
This work was supported in part by a Grant-in-Aid for Scientific Research (C) from the Japan Society for the Promotion of Science (JSPS) (to J. K.); the “Academic Frontier” Project for Private Universities from the Ministry of Education, Culture, Sports, Sciences and Technology of Japan (to S. Y.). Financial support was also provided by the Specific Research Fund of Hokuriku University (to N. K.). All are gratefully acknowledged.
References
1. B. M. Trost and I. Fleming, ‘Comprehensive Organic Synthesis’ Vol. I, Pergamon Press, New York, 1991.
2. M. Schlosser, ‘Organometallics in Synthesis, A Manual’, John Wiley & Sons, Chichester, 2002; R. G. Jones and H. Gilman, ‘Organic Reactions’, Vol. VI ed. by R. Adams, John Wiley & Sons, New York, 1951, pp. 339-366; H. Gilmane and J. W. Molton Jr., ‘Organic Reactions’, Vol. VIII, ed. by R. Adams, John Wiley & Sons, New York, 1954, pp. 258-304.
3. T. W. Green and P. G. M. Wuts, ‘Protective Groups in Organic Synthesis’, 3rd ed., John Wiley & Sons, New York, 1999.
4. J. Emsley, ‘The Elements’, Clarendon Press, Oxford, 1998.
5. M. Fujiwara, M. Tanaka, A. Baba, H. Ando, and Y. Souma, J. Organomet. Chem., 1996, 508, 49. CrossRef
6. N. Kakusawa and J. Kurita, Chem. Pharm. Bull., 2008, 56, 1502; CrossRef N. Kakusawa, S. Yasuike, and J. Kurita, Heterocycles, 2009, 77, 1269. CrossRef
7. N. Kakusawa, Y. Tobiyasu, S. Yasuike, K. Yamaguchi, H. Seki, and J. Kurita, J. Organomet. Chem., 2006, 691, 2953; CrossRef N. Kakusawa and J. Kurita, Heterocycles, 2006, 68, 1335. CrossRef
8. W. Qin, N. Kakusawa, Y. Wu, S. Yasuike, and J. Kurita, Chem. Pharm. Bull., 2009, 57, 436 and references cited therein. CrossRef
9. H. Yamamoto and K. Oshima, ‘Main Group Metals in Organic Synthesis’, Wiley-VCH, Weinheim, 2004; CrossRef K.-y. Akiba, ‘Chemistry of Hypervalent Compounds’, Wiley-VHC, New York, 1999.
10. M. Sasaki, M. Ueda, and N. Miyaura, Angew. Chem. Int. Ed., 1998, 37, 3279; CrossRef M. Pucheault, S. Darses, and J.-P. Genet, Chem. Commun., 2005, 4714; CrossRef S. U. Son, S. B. Kim, J. A. Reingold, G. B. Carpenter, and D. A. Sweigart, J. Am. Chem. Soc., 2005, 127, 12238; CrossRef R. B. C. Jagt, P. Y. Toullec, J. G. de Vries, B. L. Feringa, and A. J. Minnaard, Org. Biomol. Chem., 2006, 4, 773; CrossRef C. Qin, H. Wu, J. Cheng, X. Chen, M. Liu, W. Zhang, W. Su, and J. Ding, J. Org. Chem., 2007, 72, 4102; CrossRef T. Yamamoto, T. Ohta, and Y. Ito, Org. Lett., 2005, 7, 4153. CrossRef
11. J. Siewert, R. Sandmann, and P. Zezschwitz, Angew. Chem. Int. Ed., 2007, 46, 7122. CrossRef
12. C.-J. Li and Y. Meng, J. Am. Chem. Soc., 2000, 122, 9538; CrossRef S. Oi, M. Moro, H. Fukuhara, T. Kawanishi, and Y. Inoue, Tetrahedron, 2003, 59, 4351. CrossRef
13. M. Murata, R. Shimazaki, M. Ishikura, S. Watanabe, and Y. Masuda, Synthesis, 2002, 6, 717; CrossRef S. Oi, M. Moro, and Y. Inoue, Organometallics, 2001, 20, 1036. CrossRef
14. M. Ueda and N. Miyaura, J. Org. Chem., 2000, 65, 4450. CrossRef
15. Typical experimental procedure: The reaction of Sb-phenyl-1,5-azastibocine (1) (1.0 mmol) with various aldehydes (2a−j) (1.5 mmol) was conducted in 1,2-DCE (3 mL) at 80 ºC using [RhCl(cod)]2 (5 mol%) and n-Bu3P (5 mol%) under aerobic conditions.
16. C. Krug and J. F. Hartwig, J. Am. Chem. Soc., 2002, 124, 1674. CrossRef