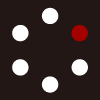
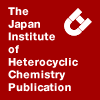
HETEROCYCLES
An International Journal for Reviews and Communications in Heterocyclic ChemistryWeb Edition ISSN: 1881-0942
Published online by The Japan Institute of Heterocyclic Chemistry
e-Journal
Full Text HTML
Received, 2nd July, 2009, Accepted, 18th August, 2009, Published online, 20th August, 2009.
DOI: 10.3987/COM-09-11789
■ Diels-Alder Reactions between Acrolein N,N-Dimethylhydrazone and N-Benzylated Benzotriazole-, Indazole- or Indole-4,7-diones
Christelle Marminon,* Bernard Fenet, Valeria Mignosi, Raphael Terreux, and Pascal Nebois
INSERM U 863, Laboratory of Organic Chemsitry , University of Lyon 1, ISPB, 8 avenue Rockefeller, 69373 Lyon cedex 08, France
Abstract
Diels-Alder reactions between acrolein N,N-dimethylhydrazone 4 and N-benzylated benzotriazole, indazole and indole-4,7-diones 1, 2 and 3 afforded new heterotricyclic quinones 5, 7 and 9 including from 2 to 4 intracyclic nitrogen atoms. A structural assignment of all new compounds was achieved using 2D NMR 1H-13C HMBC correlations. The single 1,8- regioisomer was isolated starting from benzotriazole or indazole quinones, while a mixture of the 1,5- and 1,8- regioisomers was obtained from an indole-4,7-dione derivative. In all cases, the observed regiochemistry was in agreement with FMO calculation.INTRODUCTION
Heterocyclic para-quinones containing a nitrogen atom have been reported to possess various pharmacological properties.1,2 Structure-activity relationship studies showed the importance of the number and the position of the nitrogen atom for cytotoxicity.2
In order to quantify these parameters, we recently3 reported the synthesis of heterobicyclic quinones 1-3 (Figure 1). In this paper, we describe a supplementary work which consisted in introducing an additional pyridine ring.
The most widely methodology used4 to build a pyridine ring in one step is the [4+2] cyloaddition reaction using α,β-unsaturated dialkylhydrazones as dienes. Among them, we chose acrolein N,N-dimethylhydrazone 45 in order to obtain an unsubstituted pyridine ring. This strategy led to tricyclic quinones, two of which were new original frameworks: the triazolo- and pyrazolo[4,5-g]quinoline-4,9-diones. To our knowledge, these skeletons have not been referenced so far.
RESULTS AND DISCUSSION
1. CHEMISTRY
There is no example of Diels-Alder reactions between α,β-unsaturated dialkylhydrazones and benzotriazole- or indazole-4,7-dione derivatives. The best conditions we found for the reactions described in Scheme 1 were a heating at 50-60 °C in acetonitrile for one or two days. Tricyclic quinones 5 or 7 were then regiospecifically obtained in 85 and 84% yield respectively, along with byproducts 6 or 8 (6% and 13% yield respectively). The latter ones result from the addition to the starting quinones of dimethylamine, which is released during the oxidative process of the primary cycloadduct. Attempts to minimize this addition pathway by adding acetic6 anhydride in the reaction mixture failed.
Pyrrolo[4,5-g]quinoline-4,9-dione derivatives7,8 are more described, due to their great biological activities.9 Only one synthesis of this skeleton was described using a hetero-Diels-Alder reaction8 although few examples10 of [4+2] cyloadditions of indolo-4,7-diones with α,β-unsaturated dialkylhydrazones are reported in the literature. In all cases, a mixture of 1,5- and 1,8- regioisomers was obtained in moderated overall yields. The 1,5-/1,8- ratio varies according to the nature of the substituent on the nitrogen atom of the starting quinone. The reaction described in Scheme 2 was carried out in acetonitrile at 50 °C for 36 hours and afforded a mixture of the regioisomers 9a and 9b (ratio 9a/9b: 58/42) with 55% overall yield.
2. STRUCTURAL ANALYSIS
Assignment of regiochemistry was established for each compound by 2D NMR HSQC and 1H-13C HMBC correlations, using the characteristic long-range 3J, 4J and 5J couplings. All of the cross couplings observed are reported in a table, and the decisive correlations for the structural assignment are summarized in the corresponding figure. For every compound, the carbonyls C-4 and C-9 (or C-7) were firstly attributed due to the 4J coupling between the methylene protons 1’-H and C-9 (or C-7). Then, couplings between the pyridine protons and the carbonyls were analyzed.
For compound 5 (Table 1), the 5-H proton showed couplings with C-4 (3JH,C) and C-9 (4JH,C), while the 7-H proton correlated only with C-4 (5JH,C). These data allowed us to determine the regiochemistry of 5.
Surprisingly, for quinone 7 (Table 2), no correlation was observed between the proton 3-H and the carbon of the carbonyl groups in spite of additional NMR experiments. NMR studies of the starting indazole-4,7-dione 2 showed the same phenomenon (Table 3), which is related for several quinones linked to a pyrrolo11 or pyrazolo12 ring. However, as for compound 5, the spectrum showed the same couplings between the 5-H proton with C-4 (3JH,C) and C-9 (4JH,C) and the 7-H proton with C-4 (5JH,C). On the other hand, the 6-H proton correlated with C-9 (5JH,C). So the comparative study with NMR data obtained from compound 5 led us to hypothesize a similar regiochemistry.
In order to confirm this hypothesis, a reductive acetylation of quinone 7 was carried out, following a known procedure,13 to afford the diacetate 10 in 27 % yield (scheme 3).
The cross couplings (Figure 2) found in the NOESY spectrum agreed with the proposed regiochemistry, confirming unambiguously the assignment of 7.
Finally, a comparative study of the spectra (Tables 4 and 5) of the pyrrolo derivatives 9a and 9b allowed us to determine the structure of each regioisomer. Indeed, they showed differences in the couplings between the proton opposite to the N atom of the pyridine ring (4JH,C-9 and 3JH,C-4 for the major isomer 9a, the reverse for the minor isomer 9b: 4JH,C-4 and 3JH,C-9) and the coupling between the proton next to the same N atom (5JH,C-4 for regioisomer 9a, not seen for 9b).
Assignment of regiochemistry was also established for by-products 6 and 8 by 2D NMR 1H-13C HMBC correlations (Tables 6 and 7).
For compound 6, the 1’-H protons correlated with C-7a (3JH,C), C-3a and C-7 (4JH,C). Moreover, the 6-H proton showed couplings with C-5, C-7 (2JH,C), C-4 and C-7a (3JH,C). These data allowed us to determine the structure of 6.
For derivative 8, correlations between the 6’-H protons and C-5 (3JH,C), C-4, C-6 (4JH,C), C-3a and C-7 (5JH,C) were observed. On the other hand, the 6-H proton showed couplings with C-5, C-7 (2JH,C), C-4, C-7a (3JH,C) and C-3a (4JH,C). So these facts are in favour of the proposed structure.
3. FRONTIER MOLECULAR ORBITAL CONSIDERATIONS
In order to confirm the above assignments, quantum chemistry calculations were performed using the SYBYL suite of programs (by Tripos, Inc.). The geometry of the molecule and the energy were computed using Gaussian 98 version A10 program (Gaussian Inc.). First, the geometry of each molecule was optimized using Density Functional Theory (DFT)14 with the B3LYP15 method for the molecule using 6-31G** for the atomic basis set. The partial charges were extracted. The coefficient of the atoms of the HOMO orbital for diene compound and LUMO orbital for dienophile compound were sums. These values give in absolute value information about the electron density of these atoms (Table 8).
The calculations (Table 8) indicate that for acrolein N,N-dimethylhydrazone 4 the largest coefficient is located at the carbon C-4, while for the quinones it is always situated at C-5. So the major regioisomers should result from the attack of the C-4 diene to the C-5 of the different quinones. Regioselectivities should be important, due to the large difference of the coefficient values, except perhaps for the indole-4,7-dione derivative 3. Finally, these predictions agree with the observed regiochemistry for each cycloaddition: the 1,8-regioisomer is the single or the major product formed.
Moreover, the theoretical calculations can also be used to estimate the regiochemistry of by-products 6 and 8. Even if partial charge calculations show no or only minor difference between C-5 and C-6 (Table 8), the electronic density, smaller in the largest HOMO, would promote the attack on this location. These predictions agree with the established regiochemistry too.
CONCLUSION
We synthesized from a short process new heterotricyclic derivatives including a para quinoline moiety. Assignment of all isolated regioisomers were achieved using 2D NMR 1H-13C HMBC correlations. Evaluation of antiparasitic and antitumoral potential of all compounds is currently underway.
EXPERIMENTAL
1. GENERAL PROCEDURES
Melting points were measured with a Büchi apparatus (capillary tube). The 1H- (300 MHz) and 13C-NMR (75 MHz) spectra were recorded on a Bruker DRX 300 spectrometer. The 2D NMR experiments were recorded on a Bruker DRX 500 spectrometer. 1H-13C HSQC and HMBC and NOESY experiments used gradients for better coherence selection. The chemical shifts are reported in ppm (δ) using tetramethylsilane (TMS) as an internal reference. J values are given in Hz. The infrared spectra were recorded with a Perkin-Elmer 1310 spectrometer. Mass spectra (EI) were recorded with a GC/MS Nermag R10-10 spectrometer. Elemental analyses were performed at the Centre de Microanalyse du CNRS at Solaize (France). Column chromatography were carried out with Matrex (60 Å, 35-70 µm) acidic silica gel. Acrolein N,N-dimethylhydrazone 4,5 benzotriazole-4,7-dione 1, indazole-4,7-dione 2 and indole-4,7-dione 33 were prepared according the procedures described in the literature.
2. [4+2] CYCLOADDITIONS
3-Benzyl-3H-[1,2,3]triazolo[4,5-g]quinoline-4,9-dione (5) 5-(Dimethylamino)benzotriazole-4,7-dione (6): To a solution of benzotriazole-4,7-dione 1 (150 mg, 0.63 mmol) in MeCN (5 mL), was added the acrolein N,N-dimethylhydrazone 4 (74 mg, 0.75 mmol) in MeCN (2 mL). After stirring for 24 h at 50 °C, the mixture was cooled and the solvent evaporated to dryness under vacuum. The crude residue was then crystallized with Et2O at 0 °C and the quinolinedione filtered 5 (140 mg, 0.47 mmol, 75%). The filtrate was evaporated under vacuum and the residue purified by column chromatography (AcOEt/petroleum ether, 1/1) to lead to 6 (10 mg, 0.035 mmol, 6%) and 5 (19 mg, 0.065 mmol, 10 %). (5): brown crystals; Rf = 0.18 (AcOEt/petroleum ether, 1/1); mp 133 °C; IR(KBr) 1686, 1574, 1483 cm-1; 1H NMR (DMSO-d6) δ 6.10 (2H, s, CH2-Ph), 7.37-7.48 (5H, m, H Ph), 7.95 (1H, dd, J1 = 7.9 Hz, J2 = 4.7 Hz, H-6), 8.60 (1H, dd, J1 = 7.9 Hz, J2 = 1.5 Hz, H-5), 9.00 (1H, dd, J1 = 4.7 Hz, J2 = 1.5 Hz, H-7); 13C NMR (DMSO-d6) δ 52.8, 128.7 (2C), 128.9, 129.2, 129.6 (2C), 131.4, 134.6, 135.3, 135.9, 145.5, 149.8, 159.8, 174.1, 177.1. HRMS calcd for C16H11N4O2: 291.0882 [M+H]+, found: 291.08816. (6): red powder; Rf = 0.55 (AcOEt/petroleum ether, 1.5/1); mp 189 °C; IR(KBr) 1702, 1618, 1541 cm-1; 1H RMN (CDCl3) δ 3.28 (6H, s, 2CH3), 5.54 (1H, s, H-5), 5.89 (2H, s, CH2-Ph), 7.30-7.38 (3H, m, H Ph), 7.44-7.53 (2H, m, H Ph); 13C RMN (CDCl3) δ 44.1 (2C), 53.3, 104.7, 129.1 (2C), 129.2, 129.3 (2C), 133.4, 134.7, 143.5, 153.2, 174.0, 176.6; HRMS calcd for C15H15N4O2: 283.1195 [M+H]+, found: 283.1198.
1-Benzyl-1H-pyrazolo[4,5-g]quinoline-4,9-dione (7) and 5-(Dimethylamino)indazole-4,7-dione (8): To a solution of indazole-4,7-dione 2 (150 mg, 0,66 mmol) in MeCN (5 mL), was added 4 (78 mg, 0.80 mmol) in MeCN (2 mL). After stirring for 48 h at 60 °C, the mixture was cooled and the solvent evaporated to dryness under vacuum. The crude residue was then crystallized with Et2O at 0 °C and the quinolinedione 7 filtered (115 mg, 0.40 mmol, 63%). The filtrate was evaporated under vacuum and the residue purified by column chromatography (AcOEt/petroleum ether, 1/1) led to 8 (red, 23 mg, 0.082 mmol, 13%) and 7 (38 mg, 0.013 mmol, 21%). (7): pink crystals; Rf = 0.14 (AcOEt/petroleum ether, 1/1); mp 177 °C; IR(KBr) 1687, 1673, 1532 cm-1; 1H NMR (DMSO-d6) δ 5.92 (2H, s, CH2-Ph), 7.25-7.43 (5H, m, H Ph), 7.91 (1H, dd, J1 = 7.9 Hz, J2 = 4.7 Hz, H-6), 8.34 (1H, s, H-3), 8.53 (1H, dd, J1 = 7.7 Hz, J2 = 1.5 Hz, H-5), 9.08 (1H, dd, J1 = 4.5 Hz, J2 = 1.5 Hz, H-7); 13C NMR (DMSO-d6) δ 55.3, 123.4, 128.6 (2C), 128.8 (2C), 128.9, 129.5, 131.4, 135.5, 136.8, 138.2, 138.5, 149.9, 154.7, 174.5, 179.0; Anal. Calcd for C17H11N3O2: C, 70.58; H, 3.83; N, 14.53; O, 11.06. Found: C, 70.27; H, 4.02; N, 14.17; O, 10.95. (8): Rf = 0.72 (AcOEt/petroleum ether, 1.5/1); mp 119 °C; IR(KBr) 1677, 1619, 1535 cm-1; 1H RMN (CDCl3) δ 3.24 (6H, s, 2CH3), 5.49 (1H, s, H-6), 5.77 (2H, s, CH3-Ph), 7.19-7.45 (5H, m, H Ph), 7.86 (1H, s, H-3); 13C RMN (CDCl3) δ 43.8 (2C), 54.7, 105.1, 121.1, 128.6, 128.9 (2C), 129.1 (2C), 136.2, 137.5, 137.9, 153.6, 176.0, 179.2; HRMS calcd for C16H16N3O2: 282.1243 [M+H]+, found: 282.1247.
1-Benzyl-1H-pyrrolo[4,5-g]quinoline-4,9-dione (9): To a solution of indole-4,7-dione 3 (140 mg, 0.59 mmol) in MeCN (13 mL), was added 4 (48 mg, 0.42 mmol) in MeCN (4 mL). After stirring for 36 h at 50 °C, the mixture was evaporated and the crude purified by column chromatography (AcOEt/petroleum ether, 2/1) then (CHCl3/acetone/NH4OH, 150/5/1) to lead to 9a (54 mg, 0.19 mmol, 32 %) and 9b (39 mg, 0.135 mmol, 23 %).
(9a): yellow crystals; Rf = 0.86 (CHCl3/acetone/NH4OH, 100/20/5); mp 158 °C; IR(KBr) 1673, 1666, 1496 cm-1; 1H NMR (CDCl3) δ 5.70 (2H, s, CH2-Ph), 7.04 (1H, s, H-2); 7.29-7.40 (6H, m, H-3 + H Ph), 7.63 (1H, dd, J1 = 7.9 Hz, J2 = 4.7 Hz, H-6), 8.50 (1H, dd, J1 = 7.9 Hz, J2 = 1.7 Hz, H-5), 8.97 (1H, dd, J1 = 4.7 Hz, J2 = 1.5 Hz, H-7); 13C NMR (CDCl3) δ 53.0, 113.6, 123.1, 127.2, 128.1, 128.3 (2C), 128.7, 129.1 (2C), 129.2, 130.4, 134.8, 135.2, 149.1, 153.8, 173.7, 178.3; HRMS calcd for C18H13N2O2: 289.0977 [M+H]+, found: 289.09769.
(9b): yellow crystals; Rf = 0.78 (CHCl3/acetone/NH4OH, 100/20/5); mp 178 °C; IR(KBr) 1687, 1661, 1491 cm-1; 1H NMR (CDCl3) δ 5.61 (2H, s, CH2-Ph), 6.97 (1H, s, H-2); 7.14-7.34 (6H, m, H-3 + H Ph), 7.56 (1H, dd, J1 = 7.7 Hz, J2 = 4.5 Hz, H-7), 8.40 (1H, dd, J1 = 7.7 Hz, J2 = 1.5 Hz, H-6), 8.94 (1H, dd, J1 = 4.5 Hz, J2 = 1.5 Hz, H-8); 13C NMR (CDCl3) δ 52.8, 114.3, 123.9, 126.9, 127.8 (2C), 128.7, 129.0, 129.1 (2C), 129.4, 130.3, 134.7, 135.2, 149.2, 153.9, 174.5, 177.5; HRMS calcd for C18H13N2O2: 289.0977 [M+H]+, found: 289.09769.
3. REDUCTIVE ACETYLATION
4-(Acetyloxy)-1-benzyl-1H-pyrazolo[4,5-g]quinolin-9-yl acetate (10): A mixture of 2 (50 mg, 0.17 mmol), zinc powder (255 mg, 3.91 mmol), sodium acetate (89 mg, 1.08 mmol) and acetic anhydride (24 mL) was stirred for 22 h. The mixture was then diluted with CH2Cl2 (100 mL) and filtered. The filtrate was evaporated and purified by column chromatography (AcOEt/petroleum ether, 1.5/1) then (CH2Cl2/AcOEt, 4/1) to give 10 (17 mg, 0.045 mmol, 27%). (10): pale yellow powder; Rf = 0.54 (CH2Cl2/ AcOEt, 2/1); mp degradation >143 °C; IR(KBr) 1755, 1642, 1196 cm-1; 1H NMR (CDCl3) δ 2.30 (3H, s, CH3), 2.50 (3H, s, CH3), 5.76 (2H, s, CH2-Ph), 7.06-7.30 (5H, m, H Ph), 8.10 (1H, s, H-3), 8.22 (1H, dd, J1 = 8.7 Hz, J2 = 1.5 Hz, H-5), 8.82 (1H, dd, J1 = 3.8 Hz, J2 = 1.5 Hz, H-7); 13C NMR (CDCl3) δ 54.8, 20.8, 20.9, 54.8, 116.7, 119.4, 120.4, 126.7 (2C), 127.2, 127.9, 128.8 (2C), 128.9, 130.9, 132.0, 132.9, 135.3, 137.4, 137.7, 151.6 168.6, 168.9; HRMS calcd for C21H18N3O4: 376.1297 [M+H]+, found: 376.1298.
ACKNOWLEDGEMENTS
We thank Jacques Gentili for supplying the starting material indole-4,7-dione.
References
1. V. K. Tandon, D. B. Yadav, A. K. Chaturvedi, and P. K. Shukla, Bioorg. Med. Chem. Lett., 2005, 15, 3288. CrossRef
2. J. S. Kim, H.-J. Lee, M.-E. Suh, H.-Y. P. Choo, S. K. Lee, H. J. Park, C. Kim, S. W. Park, and C. O. Lee, Bioorg. Med. Chem., 2004, 12, 3683; CrossRef I. A. Shaikh, F. Johnson, and A. P. Grollman, J. Med. Chem., 1986, 29, 1329. CrossRef
3. C. Marminon, J. Gentili, R. Barret, and P. Nebois, Tetrahedron, 2007, 63, 735. CrossRef
4. B. Serckx-poncin, A.-M. Hesbain-Frisque, and L. Ghosez, Tetrahedron Lett., 1982, 23, 3261; CrossRef F. Pautet, P. Nebois, Z. Bouaziz, and H. Fillion, Heterocycles, 2001, 54, 1095; CrossRef M. Behforouz and M. Ahmadian, Tetrahedron, 2000, 56, 5259; CrossRef Q. Fan, L. Lin, J. Liu, Y. Huang, X. Feng, B. Wang, and G. Zhang, Org. Lett., 2004, 6, 2185; CrossRef F. Alvarez, A. Taleb, J. Gentili, P. Nebois, R. Terreux, M. Domard, A. Thozet, D. Merle, H. Fillion, and N. Walchshofer, Eur. J. Org. Chem., 2005, 1903. CrossRef
5. E. Gomez-Bengoa and M. A. Echavarren, J. Org. Chem., 1991, 56, 3497. CrossRef
6. M. Chigr, H. Fillion, and A. Rougny, Tetrahedron Lett., 1988, 29, 5913; CrossRef P. Nebois, R. Barret, and H. Fillion, Tetrahedron Lett., 1990, 31, 2569. CrossRef
7. M.-E. Suh, S.-Y. Park, and C.-O. Lee, Bioorg. Med. Chem., 2001, 9, 2979; CrossRef M.-E. Suh, M.-J. Kang, and S.-Y. Park, Bioorg. Med. Chem., 2001, 9, 2987. CrossRef
8. L. Legentil, J. Bastide, and E. Delfourne, Tetrahedron Lett., 2003, 44, 2473. CrossRef
9. J.-M. Seo, T.-J. Kim, Y.-R. Jin, H.-J. Han, C.-K. Ryu, Y.Y. Sheen, D.-W. Kim, and Y.-P. Yun, Eur. J. Pharmacol., 2008, 586, 74; CrossRef M.-E. Suh, H.-K. Park, H.-W. Yoo, and C. O. Lee, Biol. Pharm. Bull., 2000, 23 (3), 354.
10. R. Barret, N. Roue, and H. Fillion, Chem. Pharm. Bull., 1998, 46, 548; J. Gentili and R. Barret, Tetrahedron Lett., 2005, 46, 1639. CrossRef
11. R. A. Tapia, Y. Prieto, F. Pautet, N. Walchshofer, H. Fillion, B. Fenet, and M.-E. Sarciron, Bioorg. Med. Chem., 2003, 11, 3407. CrossRef
12. L. Legentil, L. Benel, V. Bertrand, B. Lesur, and E. Delfourne, J. Med. Chem., 2006, 49, 2979. CrossRef
13. J. Lee and J. K. Snyder, J. Org. Chem., 1990, 56, 4995. CrossRef
14. P. Hohenberg and W. Kohn, Phys. Rev. Lett., 1964, 136, B864; CrossRef W. Kohn and L. J. Sham, Phys. Rev., 1965, 140 (4A), A1133; CrossRef R. Parr and W. Yang, 'Density-functional theory of atoms and molecules', vol. 16, Oxford University Press, New York, 1989; D. R. Salahub and M. C. Zerner, ACS Symp. Ser., 1989, 394, 1.
15. A. D. Becke, J. Chem. Phys., 1993, 98, 5648; CrossRef J. A. Pople, M. Head-Gordon, D. J. Fox, K. Raghavachari, and L. A. Curtiss, J. Chem. Phys., 1989, 90, 5622; CrossRef L. A. Curtiss, C. Jones, G. W. Trucks, K. Raghavachari, and J. A. Pople, J. Chem. Phys., 1990, 93, 2537 CrossRef