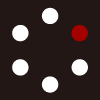
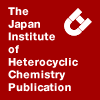
HETEROCYCLES
An International Journal for Reviews and Communications in Heterocyclic ChemistryWeb Edition ISSN: 1881-0942
Published online by The Japan Institute of Heterocyclic Chemistry
e-Journal
Full Text HTML
Received, 15th July, 2009, Accepted, 11th September, 2009, Published online, 15th September, 2009.
DOI: 10.3987/COM-09-11797
■ Stereoselective Synthesis of Isoquinuclidinones by Direct Imino-Diels-Alder Type Reaction Catalyzed by L-Proline
Noelia Quiñones, Fernándo Aznar,* and María-Paz Cabal
instituto Universitario de Química Organometálica "Enrique Moles", Facultad de Química, Universidad de Oviedo, Julián Clavería 8, 33071- Oviedo, Spain
Abstract
A general synthesis of isoquinuclidinones (2-azabicyclo[2.2.2]octan-5-ones), has been developed by one-pot three component imino-Diels-Alder type reaction catalyzed by L-proline. Simple and commercially available α,β-unsaturated cyclic ketones, aromatic aldehydes and either aliphatic or aromatic primary amines were used. The reaction proceeds with excellent exo-diastereoselectivity (>99%) and moderated enantioselectivity (30-64%).INTRODUCTION
Over the past few years organocatalysis has become very popular for a wide range of enantioselective transformations.1 In particular, L-proline and other chiral amines2 have been reported to catalyze asymmetric aldol,3 Michael,4 Mannich,5 Diels-Alder,6 hetero-Diels-Alder7 and related addition reactions. As part of our ongoing interests in the stereoselective synthesis of piperidin-4-ones, we have previously developed methodologies employing the imino-Diels-Alder reaction of 2-amino-1,3-butadienes with aldimines.8
Recently, we reported an efficient route to meso- and cis-2,6-diarylpiperidin-4-ones by imino-Diels-Alder reaction of an acyclic enone with an aldimine in the presence of L-proline as a catalyst (Scheme 1).9a Alternatively, this procedure can be carried out more conveniently by generating the aldimine in situ from an aryl aldehyde and a primary amine.9b In both cases, the proline-promoted cycloaddition occurs through in situ generation of a 2-amino-1,3-butadiene II, produced by tautomerization of iminium ion I (Scheme 2).
Using cyclic enones as substrates for these cycloadditions, we envisoned extending this methodology to the synthesis of more complex heterocyclic ring systems such as isoquinuclidinones (2-azabicyclo [2.2.2]octan-5-ones), which are common structures of alkaloids having important biological properties.10 Retrosynthetically, isoquinuclidinones can be obtained by an imino-Diels-Alder cycloaddition between an imine and a 2-amino-1,3-diene derived from cyclohexenone, as shown in Scheme 3.
Furthermore, the use of L-proline as a chiral catalyst (R3R4*NH) to generate the 2-amino-1,3-diene in situ provides an opportunity to control enantiofacial selectivity in the Diels-Alder cycloaddition. Isoquinuclidinones have previously been prepared by an imino-Diels-Alder reaction between a cyclohexenone and an imine in the presence of Lewis11 or a Brönsted acid.12 Córdova et al.13 reported the first enantioselective proline-catalyzed imino-Diels-Alder reaction between cyclohexenones, formaldehyde and 4-methoxyaniline in a single pot. Although high enantioselectivity was observed, the reaction was restricted to the use of formaldimines and ethyl N-(p-methoxyphenyl)-α-imino-glyoxalate as the imines. In this report, we demonstrate the application of this methodology to the enantioselective synthesis of isoquinuclidinones, by a proline-catalysed three-component imino-Diels-Alder coupling of cyclohexenone, an aldehyde, and a primary amine.
RESULTS AND DISCUSSION
We initially investigated as a model system the proline-catalyzed reaction between the commercially available 4,4-dimethylcyclohexenone 1a (2 mmol), benzaldehyde 2a (1 mmol), and allylamine 3a (1.1 mmol) in methanol (2 mL). After vigorously stirring the mixture for 24 hours, the reaction was quenched by extraction and the crude product was purified by columm chromatography on silica gel to furnish the desired isoquinuclidine 5a as a mayor diastereoisomer (97% diastereoselectivity), in 35% yield and 30% enantiomeric excess (Scheme 4). This product was shown to have the exo relative stereochemistry by NMR.
The exo product was confirmed by NOESY experiments that showed a strong nuclear Overhauser enhancement (NOE) between the aromatic protons at the ortho position and the ones of the methyl group in the bridge, suggesting that the phenyl group has to be on the same side of the bridge (Figure 1).
Encouraged by this experiment, we investigated different reaction conditions to optimize this process (Table 1). First, we studied the effect of the solvent. Neither anhydrous solvents nor use of inert atmosphere conditions were found to be necessary or beneficial. We conducted the reaction with different organic solvents and reaction times, and found that the highest enantioselectivity as well as efficiency was obtained in MeOH for seven days (Table 1, entry 2). When DMSO and CH2Cl2 were used, only 10% of compound 5a was observed. When the reaction was performed in THF, CH3CN, DMF, toluene or dioxane, none of the desired bicyclic product was obtained; only starting material was recovered from these reaction mixtures.
The concentration of the substrate also affects the yield of the reaction.14 Thus, increasing the number of equivalents of α,β-unsaturated ketone 1a from two to four and with a longer reaction time, increased the product yield to 77% and the enantioselectivity slightly, to 37% ee (Table 1, entry 2). Lower or higher molar ratios gave no beneficial results.
Another important factor was the effect of reaction temperature (Table 1, entries 3-6) on the reaction yield and enantioselectivity. When the reaction was carried out in methanol at lower temperatures (Table 1, entries 3-4), lower yields, diastereo- and enantioselectivities were obtained; and at higher temperatures (Table 1, entries 5-6) the yields increased but lower enantioselectivities were observed. We conclude that the best result was when the reaction was carry out at room temperature. Thus, the performance of the reaction at 25 ºC could give the desired product 5a in 77% yield with high diastereoselectivity (>99%) and 37% ee (Table 1, entry 2).
The addition of achiral alkali cations as additives has been reported in the literature to increase the enantioselectivity of catalyzed asymmetric transformations.15 Thus, we decided to investigate the effect of this positive additive effect on our reaction. Unfortunately, we found that in our case the addition of salts (Table 1, entries 7-10) increased the yield of the reaction but sacrified the enantioselectivity.
In addition to proline we also investigated other organocatalysts to mediate the model reaction (Table 2).
The catalyst screen revealed that L-proline 4a (Table 2, entries 1, 2), proline derivatives 4b-4e (Table 2, entries 3-7) and 4h (Table 2, entry 11) all catalyzed the asymmetric formation of the corresponding bicyclic compound 5a. However, L-proline afforded the highest stereoselectivity under the reaction conditions. When prolinol 4f-4g (Table 2, entries 8-10), imidazole 4i (Table 2, entries 12-14) and cinchone 4j (Table 2, entry 15) derivatives were used no enantioselectivity was found even when an acid co-catalyst (p-toluensulfonic acid or benzoic acid) was added.
Based on these results and the practical aspects of employing proline catalysis we decided to investigate the scope of the three-component aza-Diels-Alder reaction for the synthesis of isoquinuclidines 5, for an expanded set of different α,β-unsaturated cyclic ketones, aromatic aldehydes and aromatic or aliphatic amines (Table 3).
We chose as α,β-unsaturated cyclic ketone 4,4-dimethylcyclohexenone 1a (entries 1-11), cyclohexenone 1b (entries 12-13) and cycloheptenone 1c (entry 14). The best yield was obtained with cycloheptenone 1c (entry 14) and 4,4-dimethylcyclohexenone 1a (entry 1); the ee is moderate in both cases but the diastereoselectivity is higher with 1a. It is noteworthy that the two methyl groups at the C-4 position in the cyclohexenone seem to enhance the yield and ee of the reaction (entry 1 vs 13).
The structure of the aryl moiety does not affect the enantiomeric excess, regardless of the presence of electron-rich aryl substituents (MeO, Me2N, entries 2-3) or electron poor groups (CN, entry 4). When the aryl substituent is a halogen, we observed higher ee values having the halogen in the C-2 position (entries 7-8) versus C-4 (entries 3-6). The use of heterocyclic aldehydes did not improve the ee values (entry 9).
The influence of the N-substituent of the imine on the enantioselectivity is not clear. Thus, when we employed the PMP group as the N-substituent of the imine, the enantioselectivity of the cycloaddition range from 20% (entry 10) with 4,4-dimethylcyclohexenone to 64% with cyclohexenone (entry 12). As aliphatic amines, we used allylamine which have the additional and attractive advantage that the allyl group can be easily removed after the cycloaddition.9
In general we can conclude that the enantioselectivity for this imino Diels-Alder reaction between α,β-unsaturated cyclic ketones, aromatic aldehydes and N-allyl or N-p-methoxyphenylamines depends on each combination. The best results were obtained during the formation of (exo)-2-(4-methoxyphenyl)-3-phenyl-2-azabicyclo[2.2.2]octan-5-one 5l, and the N-allyl-isoquinuclidine-2-aryl-substituted products 5h and 5g (Figure 2).
MECHANISTIC ANALYSIS
Based on previous experience with reactions involving chiral amine-derived dienes,17 the following mechanism is proposed to account for the stereochemical outcome of the cycloaddition reaction (Scheme 5). Thus, L-proline initially condenses with the enone to create an iminium zwitterion I, which rapidly tautomerizes to the putative chiral 2-aminodiene II (a “Barbas dienamine”).18 Next, the imine adds to the chiral diene II stereoselectively (via transition state III, a key step which controls the diastereo- and the enantioselectivity of the process) to form the activated iminium species IV. This zwitterion intermediate IV then collapses via a 6-endo-trig cyclization to furnish the Diels-Alder adduct V, which is hydrolyzed by adventitious water to produce isoquinuclidinone 5 with release of the catalyst.
As indicated in Scheme 5, the key step governing enantioselectivity of the cycloaddition occurs when the chiral dienamine intermediate II adds to the imine. There are four possible transition states IIIA-IIID to consider to explain the diastereo- and enantioselectivity of this step (Scheme 6). In transition states IIIA and IIIB the aryl group of the approaching imine may л-stack with the diene, with the R group (R = allyl) of the approaching imine lying exo to the diene system. Conversely, for transition states IIIC and IIID, the R substituent may form a favourable л-interaction with the diene, in which case the endo product would be obtained. Since the observed product of the reaction is the exo isomer, there appears to be a strong bias for transition states IIIA and IIIB during the critical first bond-forming step of the cycloaddition.
The enantioselectivity can be accounted for by considering the degree of coordination between the proline ring substituent X (of the diene component) and the available electron pair of the imine nitrogen. If a strong hydrogen bond can form between these two entities (as for transition states IIIA and IIIC), the reaction would occur from the si-face of the chiral diene13,19 to afford just one of the enantiomeric products. However, if there is only weak coordination, the imine could also add via the re-face of the diene (via transition states IIIB and IIID) yielding the other enantiomeric adduct. In our case, stereofacial control favors formation of one stereoisomeric adduct but not with complete enantioselectivity. Based on reports of similar examples in the literature, it is likely that the exo-product coming from transition state IIIA is the one that predominates in the enantiomeric mixture.
CONCLUSION
In summary, we have described a general synthesis of isoquinuclidinones by a three-component imino-Diels-Alder reaction catalyzed by L-proline, using several α,β-unsaturated cyclic ketones, aromatic aldehydes, and aromatic amines or allyl amine as reactants. The cycloaddition reaction is catalyzed by proline and proceeds with excellent exo diastereoselectivity (>99%) and moderate enantioselectivity (30-64%). It is the first time, to the best of our knowledge, that an aliphatic amine (allylamine) has been used in this kind of transformation. Further exploration of this methodology and its application to problems in synthesis are ongoing in our laboratory.
EXPERIMENTAL
General: All comercially available reagents were purchased from Aldrich or Fluka, amines and aldehydes were distilled previously to their use. Thin Layered Chromatography analyses were performed on Merk silica gel plates 60 and visualized by UV light ( λ= 254 nm) or K2MnO4 solution. Flash column chromatography was performed with Merk silica gel (60, 230-400 mesh) eluting with hexane/ethyl acetate mixtures. 1H and 13C NMR spectra were recorded at 300 or 400 MHz and 75 or 100 MHz, respectively in CDCl3 solution. Tetramethylsilane was used as internal standard for 1H and the residual solvent signals as standard for 13C. Chemical shifts are given in ppm. The data is being reported as s = singlet, br s = broad singlet, d = doublet, dd = double doublet, t = triplet, q = quatriplet, qn = quintuplet and m = multiplet or unresolved, chemical shifts in ppm and coupling constant(s) in Hz. HPLC was carried out using a Waters LC Module I plus with V- UV photodiode array detector. Enantiomeric excess were determined by HPLC using Daicel Chiracel OD-H and Daicel Chiralpak IA columns. Mass spectra were obtained by EI (70 eV) with Finnigan-Mat 95 and VG Autospec M spectrometers or by ESI or APCI with Agilent Technologies G 1946B. The adduct (exo)-2-(4-methoxyphenyl)-3-phenyl-2-azabicyclo[2.2.2]octan-5-one (5l) is known compound.11 For all the new adducts, complete characterization has been reported below.
General procedure for the preparation of isoquinuclidines 5a-n: To a mixture of aldehyde 2 (1 mmol, 1 eq.) and amine 3 (1.1 mmol, 1.1 eq.) (in case of preformed aldimine, 1 mmol was used) in MeOH (4 mL) was added L-proline (30 mol%) and α,β-unsaturated ketone 1 (4 mmol, 4 eq.). The reaction mixture was allowed to stir at room temperature for 7 days. Then, the solvent (MeOH) was evaporated under reduced pressure. CH2Cl2 (10 mL) was added to the reaction crude and the organic layer was washed with brine (3 x 10 mL). The organic layer was dried (Na2SO4), concentrated, and purified by flash column chromatography (silica gel, mixtures of hexane/EtOAc) to afford the desired pure isoquinuclidine 5a-n. Partial decomposition of these products have been observed after prolonged storage.
(exo)-2-Allyl-7,7-dimethyl-3-phenyl-2-azabicyclo[2.2.2]octan-5-one (5a): Yield 207 mg (77%), yellow oil. Rf 0.46 (hexane/EtOAc 5:1). 1H-NMR (300 MHz, CDCl3): δ = 0.92 (s, 3H), 1.30 (dd, J = 13.7, 4 Hz, 1H), 1.33 (s, 3H), 1.65 (dd, J = 13.5, 1.86 Hz, 1H), 2.32 (m,1H), 2.40 (dd, J = 18.6, 2.8 Hz, 1H), 2.70 (dd, J = 18.6, 2.4 Hz, 1H) , 2.80 (m, 1H), 3.04 (dd, J = 14, 8.6 Hz, 1H), 3.17 (m, 1H), 3.87 (s, 1H), 5.15 (m, 2H) , 5.89 (m, 1H), 7.27 (m, 1H), 7.36 (dd, J = 9.6 Hz, 2H), 7.49 (d, J = 6 Hz, 2H) ppm. 13C-NMR (75 MHz, CDCl3) δ = 27.73 (CH3), 28.19 (CH3), 33.88 (CH2), 34.42 (CH2), 34.63 (C), 53.49 (CH), 55.62 (CH2), 59.59 (CH), 62.92 (CH), 117.40 (CH2), 127.07 (CH), 127.44 (2 x CH), 128.34 (2 x CH), 136.15 (CH), 140.35 (C), 216.08 (C) ppm. HPLC (37% ee) (Daicel Chiralpak IA, hexane/ EtOH 90:10, flow rate 0.8 mL/min, λ= 205.7): major isomer: tR = 5.30 min.; minor isomer: tR = 5.82 min. HRMS calcd for C18H23NO: 269.17742, found 269.177260.
(exo)-2-Allyl-3-(4-methoxyphenyl)-7,7-dimethyl-2-azabicyclo[2.2.2]octan-5-one (5b): Yield 129 mg (43%), yellow oil. Rf 0.52 (hexane/EtOAc 5:1). 1H-NMR (300 MHz, CDCl3): δ = 0.91 (s, 3H), 1.28 (dd, J = 13.5, 4.2 Hz, 1H), 1.32 (s, 3H), 1.64 (dd, J = 13.5, 1.8 Hz, 1H), 2.27 (m, 1H), 2.40 (dd, J = 18.6, 2.7 Hz, 1H), 2.71 (dd, J = 18.6, 2.4 Hz, 1H), 2.79 (t, J = 2.4 Hz, 1H), 3.04 (dd, J = 8.7, 13.8 Hz, 1H), 3.15 (m, 1H), 3.81 (s, 3H), 3.82 (s, 1H), 5.15 (m, 2H), 5.90 (m, 1H), 6.91 (d, J = 9 Hz, 2H), 7.41 (d, J= 9 Hz, 2H) ppm. 13C-NMR (75 MHz, CDCl3) δ = 27.11 (CH3), 27.58 (CH3), 33.22 (CH2), 33.77 (CH2), 34.01 (C), 53.05 (CH), 55.58 (CH3), 54.95 (CH2), 59.04 (CH), 61.79 (CH), 113.15 (2 x CH), 116.67 (CH2), 127.80 (2 x CH), 131.58 (C), 135.61 (CH), 158.46 (C), 215.64 (C) ppm. HPLC (25% ee) (Chiralpak IA, hexane/ EtOH 90:10, flow rate 0.8 mL/min, λ= 199.4): major isomer: tR = 7.16 min.; minor isomer: tR = 8.74 min.
HRMS calcd for C19H25NO2: 299.1885, found 299.1889.
(exo)-2-Allyl-3-(4-(dimethylamino)phenyl)-7,7-dimethyl-2-azabicyclo[2.2.2]octan-5-one (5c) : Yield 207 mg (65%), orange oil. Rf 0.42 (hexane/EtOAc 3:1). 1H-NMR (300 MHz, CDCl3): δ = 0.92 (s, 3H), 1.30 (m, 1H), 1.34 (s, 3H), 1.74 (dd, J = 13.5, 1.8 Hz, 1H), 2.29 (m, 1H), 2.40 (dd, J = 18.6, 2.7 Hz, 1H), 2.71 (dd, J = 18.9, 2.4 Hz, 1H), 2.75 (t, J = 2.7 Hz, 1H), 2.96 (s, 6H), 3.03 (dd, J = 13.8, 8.7 Hz, 1H), 3.20 (m, 1H), 3.80 (s, 1H), 5.15 (m, 2H), 5.89 (m, 1H), 6.76 (d, J = 9 Hz, 2H), 7.35 (d, J = 9 Hz, 2H) ppm. 13C-NMR (75 MHz,CDCl3) δ = 27.82 (CH3), 28.28 (CH3), 34.03 (CH2), 34.45 (CH2), 34.72 (C), 40.67 (2 x CH3), 53.90 (CH), 55.60 (CH2), 59.67 (CH), 62.50 (CH), 112.50 (2 x CH), 117.28 (CH2), 127.91 (C), 128.25 (2 x CH), 136.57 (CH), 149.90 (C), 216.86 (C) ppm. HPLC (12% ee) (Daicel Chiralpak IA, hexane/ 2-propanol 99:1, flow rate 0.5 mL/min, λ= 257.9): major isomer: tR = 9.88 min.; minor isomer: tR = 10.88 min. HRMS calcd for C20H28N2O: 312.2202, found 312.2206.
(exo)-4-(2-Allyl-7,7-dimethyl-5-oxo-2-azabicyclo[2.2.2]octan-3-yl)benzonitrile (5d): Yield 85 mg (29%), brown oil. Rf 0.42 (hexane/EtOAc 5:1). 1H-NMR (300 MHz, CDCl3): δ = 0.93 (s, 3H), 1.32 (m, 4H), 1.50 (d, J = 15 Hz; 1H), 2.31 (m, 1H), 2.46 (m, 1H), 2.70 (d, J = 18.6 Hz, 1H), 2.82 (m, 1H), 3.10 (d, J = 6 Hz, 2H), 3.90 (s, 1H), 5.17 (m, 2H), 5.86 (m, 1H), 7.61 (d, J = 6 Hz, 2H), 7.67 (d, J = 9 Hz, 2H) ppm. 13C-NMR (75 MHz, CDCl3) δ = 27.69 (CH3), 28.28 (CH3), 33.90 (C), 34.59 (CH2), 34.70 (CH2), 53.01 (CH), 55.95 (CH2), 59.86 (CH), 63.03 (CH), 111.10 (C), 118.11 (CH2), 118.82 (C), 128.27 (2 x CH), 132.34 (2 x CH), 135.45 (C), 146.42 (C), 214.79 (C) ppm. HPLC (36% ee) (Daicel Chiralpak IA, hexane/ EtOH 90:10, flow rate 0.8 mL/min, λ= 222.1): major isomer: tR = 10.42 min.; minor isomer: tR = 25.24 min. MS (APCI) calcd for C19H22N2O: 294.17, found 295.1
(exo)-2-Allyl-3-(4-bromophenyl)-7,7-dimethyl-2-azabicyclo[2.2.2]octan-5-one (5e): Yield 155 mg (45%), orange oil. Rf 0.5 (hexane/EtOAc 3:1). 1H-NMR (400 MHz, CDCl3): δ = 0.91 (s, 3H), 1.28 (m, 1H), 1.32 (s, 3H), 1.61 (dd, J = 13.8, 2.1 Hz, 1H), 2.26 (m, 1H), 2.40 (dd, J = 18.6, 2.7 Hz, 1H), 2.65 (dd, J = 18.6, 2.4 Hz, 1H), 2.78 (m, 1H), 3.05 (dd, J = 14.1, 8.4 Hz, 1H), 3.12 (m, 1H), 3.81 (s, 1H), 5.16 (m, 2H), 5.86 (m, 1H), 7.36 (d, J = 8 Hz, 2H), 7.48 (d, J = 8 Hz, 2H) ppm. 13C-NMR (100 MHz, CDCl3) δ = 27.81 (CH3), 28.27 (CH3), 33.87 (CH2), 34.52 (CH2), 34.70 (C), 53.34 (CH), 55.77 (CH2), 59.78 (CH), 62.62 (CH), 117.75 (CH2), 120.94 (C), 129.23 (2 x CH), 131.57 (2 x CH), 135.87 (CH), 139.57 (C), 215.55 (C) ppm. HPLC (28% ee) (Daicel Chiralpak IA, hexane/ EtOH 90:10, flow rate 0.8 mL/min, λ= 222.4): major isomer: tR = 7.88 min.; minor isomer: tR = 17.04 min. HRMS calcd for C18H22BrNO: 349.0864, found 349.0857.
(exo)-2-Allyl-3-(4-chlorophenyl)-7,7-dimethyl-2-azabicyclo[2.2.2]octan-5-one (5f): Yield 91 mg (30%), yellow oil. Rf 0.37 (hexane/EtOAc 5:1). 1H-NMR (300 MHz, CDCl3): δ = 0.92 (s, 3H), 1.27 (m, 1H), 1.31 (s, 3H), 1.52 (dd, J = 13.5, 1.8 Hz, 1H), 2.27 (m, 1H), 2.43 (m, 1H), 2.68 (dd, J = 18.7, 2.4 Hz, 1H), 2.79 (t, J = 3 Hz; 1H), 3.11 (m, 2H), 3.84 (s, 1H), 5.15 (m, 2H), 5.88 (m, 1H), 7.33 (d, J = 9 Hz, 2H), 7.42 (d, J = 9 Hz, 2H) ppm. 13C-NMR (75 MHz, CDCl3) δ = 27.83 (CH3), 28.30 (CH3), 33.89 (CH2), 34.54 (CH2), 34.73 (C), 53.43 (CH), 55.80 (CH2), 59.83 (CH), 62.61 (CH), 117.74 (CH2), 128.65 (2 x CH), 128.86 (2 x CH), 132.88 (C) , 135.92 (CH), 139.05 (C), 215.56 (C) ppm. HPLC (33% ee) (Daicel Chiralpak IA, hexane/ EtOH 90:10, flow rate 0.5 mL/min, λ= 219.5): major isomer: tR = 11.33 min.; minor isomer: tR = 17.99 min. HRMS calcd for C18H22ClNO: 303.1390, found 303.1399.
(exo)-2-Allyl-3-(2-bromophenyl)-7,7-dimethyl-2-azabicyclo[2.2.2]octan-5-one (5g): Yield 142 mg (41%), dark orange oil. Rf 0.58 (hexane/EtOAc 3:1). 1H-NMR (300 MHz, CDCl3): δ = 0.93 (s, 3H), 1.31 (m, 1H), 1.34 (s, 3H), 1.60 (dd, J = 13.6, 1.2 Hz, 1H), 2.40 (dd, J = 12.4, 2.8 Hz, 1H), 2.53 (m, 1H), 2.77 (dd, J = 18.2, 2.4 Hz, 1H), 2.83 (s, 1H), 3.06 (d, J = 7 Hz, 2H), 4.10 (s, 1H), 5.19 (m, 2H), 5.87 (m, 1H), 7.16 (t, J = 7.6 Hz, 1H), 7.39 (t, J = 7.6 Hz, 1H), 7.54 (d, J = 7.6 Hz, 1H), 7.94 (d, J = 7.6 Hz, 1H) ppm. 13C-NMR (75 MHz, CDCl3) δ = 27.65 (CH3), 28.31 (CH3), 34.04 (CH2), 34.40 (C), 34.54 (CH2), 49.81 (CH), 55.67 (CH2), 59.82 (CH), 62.72 (CH), 117.73 (CH2), 124.07 (C), 127.19 (CH), 128.78 (CH), 129.71 (CH), 133.36 (CH), 135.93 (CH), 138.25 (C), 215.33 (C) ppm. HPLC (51% ee) (Daicel Chiralpak IA, hexane/ EtOH 90:10, flow rate 0.2 mL/min, λ= 223.1): major isomer: tR = 27.19 min.; minor isomer: tR = 24.63 min. HRMS calcd for C18H22BrNO: 347.0885, found 347.0899.
(exo)-2-Allyl-3-(2-iodophenyl)-7,7-dimethyl-2-azabicyclo[2.2.2]octan-5-one (5h): Yield 30 mg (15%), brown oil. Rf 0.36 (hexane/EtOAc 5:1). 1H-NMR (400 MHz , CDCl3): δ = 0.93 (s, 3H), 1.35 (s, 3H), 1.59 (m, 2H), 2.40 (dd, J = 14, 1.9 Hz, 1H), 2.48 (s, 1H), 2.75 (dd, J = 14, 1.4 Hz, 1H), 2.83 (s, 1H), 3.05 (d, J =4 Hz, 2H), 3.94 (s, 1H), 5.13 (d, J= 8 Hz, 1H), 5.17 (d, J = 17 Hz, 1H), 5.85 (m, 1H), 7.00 (t, J = 5 Hz, 1H), 7.41 (t, J = 5.5 Hz, 1H), 7.87 (m, 2H) ppm. 13C-NMR (100 MHz, CDCl3) δ = 30.87 (CH3), 31.86 (CH3), 36.58 (C), 38.01 (CH2), 45.46 (CH2) , 51.92 (CH), 56.73 (CH2), 59.32 (CH), 69.03 (CH), 99.85 (C), 117.02 (CH2), 128.29 (CH), 128.35 (CH), 129.30 (CH), 136.45 (CH), 139.72 (CH), 142.94 (C), 213.25 (C) ppm. HPLC (60% ee) (Daicel Chiralpak IA, hexane/ EtOH 90:10, flow rate 0.8 mL/min, λ= 204.8): major isomer: tR = 7.43 min.; minor isomer: tR = 6.45 min. MS (ESI) calcd for C18H22INO: 395.07, found 396.0.
(exo)-2-Allyl-7,7-dimethyl-3-(pyridin-3-yl)-2-azabicyclo[2.2.2]octan-5-one (5i): Yield 73 mg (27%), yellow oil. Rf 0.44 (hexane/EtOAc/Et3N 5:4:1). 1H-NMR (300 MHz, CDCl3): δ = 0.91 (s, 3H), 1.29 (s, 3H), 1.33 (m, 1H), 1.49 (d, J = 15 Hz, 1H), 2.40 (d, J = 21 Hz, 1H), 2.65 (m, 2H), 2.80 (m, 1H), 3.15 (m, 2H), 3.91 (s, 1H), 5.15 (m, 2H), 5.89 (m, 1H), 7.15 (m, 1H), 7.71 (m, 1H), 7.81 (d, J = 9 Hz, 1H), 8.54 (m, 1H) ppm.13C-NMR (75 MHz, CDCl3) δ = 27.79 (CH3), 28.46 (CH3), 34.46 (C), 34.66 (2 x CH2), 51.06 (CH), 56.03 (CH2), 60.03 (CH), 64.79 (CH), 117.67 (CH2), 121.52 (CH), 121.96 (CH), 135.84 (CH), 136.35 (CH), 149.70 (CH), 160.43 (C), 214.73 (C) ppm. HPLC (30% ee) (Daicel Chiralpak IA, hexane/ 2-propanol 90:10, flow rate 0.5 mL/min, λ= 202.8): major isomer: tR = 20.20 min.; minor isomer: tR = 25.57 min. MS (APCI) calcd for C17H22N2O: 270.17, found 271.1.
(exo)-2-(4-Methoxyphenyl)-7,7-dimethyl-3-phenyl-2-azabicyclo[2.2.2]octan-5-one (5j): Yield 100 mg (30%), yellow oil. Rf 0.31 (hexane/EtOAc 5:1). 1H-NMR (300 MHz, CDCl3): δ = 1.02 (s, 3H), 1.11 (s, 3H), 1.80 (dd, J = 14, 2.4 Hz, 1H), 1.98 (dd, J = 13.6, 3.2 Hz, 1H), 2.69 (m, 2H), 2.85 (d, J = 15 Hz, 1H), 3.69 (s, 3H), 4.13 (s, 1H), 4.77 (s, 1H), 6.58 (d, J = 9 Hz, 2H), 6.70 (d, J = 9 Hz, 2H), 7.24 (m, 5H) ppm. 13C-NMR (75 MHz, CDCl3) δ = 29.50 (CH3), 30.82 (CH3), 36.69 (C), 38.98 (CH2), 43.11 (CH2), 54.86 (CH), 55.58 (CH3), 59.85 (CH), 62.14 (CH), 114.03 (2 x CH), 114.69 (2 x CH), 125.97 (2 x CH), 127.50 (CH), 128.88 (2 x CH), 141.42 (C), 142.31 (C), 151.24 (C), 211.97 (C) ppm. HPLC (20% ee) (Daicel Chiralpak IA, hexane/EtOH 90:10, flow rate 0.8 mL/min, λ= 232.6): major isomer: tR = 12.85 min.; minor isomer: tR = 15.82 min. HRMS calcd for C22H25NO2: 335.1885, found 335.1896.
(exo)-7,7-Dimethyl-2,3-diphenyl-2-azabicyclo[2.2.2]octan-5-one (5k): Yield 91 mg (30%), orange oil. Rf 0.55 (hexane/EtOAc 3:1). 1H-NMR (300 MHz, CDCl3): δ = 1.09 (s, 3H), 1.48 (s, 3H), 1.53 (m, 1H), 1.76 (dd, J = 12 Hz, 1H), 2.61 (m, 3H), 3.98 (m, 1H), 4.83 (s, 1H), 6.78 (d, J = 9 Hz, 3H), 7.16 (m, 2H), 7.28 (m, 1H), 7.37 (m, 4H) ppm. 13C-NMR (75 MHz, CDCl3) δ = 28.45 (CH3), 28.77 (CH3), 34.04 (CH2), 34.76 (C), 39.45 (CH2), 53.59 (CH), 59.58 (CH), 63.20 (CH), 115.63 (2 x CH), 118.72 (CH), 127.18 (2 x CH), 127.2 (CH), 128.58 (2 x CH), 129.06 (2 x CH), 138.96 (C), 148.43 (C), 214.50 (C) ppm. HPLC (0% ee) (Daicel Chiralpak IA, hexane/2-propanol 99:1, flow rate 0.3 mL/min, λ= 255.8): major isomer: tR = 31.13 min.; minor isomer: tR = 35.01 min. HRMS calcd for C21H23NO: 305.1780, found 305.1780.
(exo)-2-(4-Methoxyphenyl)-3-phenyl-2-azabicyclo[2.2.2]octan-5-one (5l)11: Yield 123 mg (40%), yellow oil. Rf 0.57 (hexane/EtOAc 1:1). 1H-NMR (300 MHz, CDCl3): δ = 1.64 (m, 1H), 1.75 (m, 1H), 1.91 (m, 1H), 2.28 (m, 1H), 2.42 (dd, J = 14, 1.1 Hz, 1H), 2.68 (m, 1H), 2.80 (m, 1H), 3.72 (s, 3H), 4.46 (s, 1H), 4.72 (s, 1H), 6.57 (d, J = 6 Hz, 2H), 6.76 (d, J = 6 Hz, 2H), 7.31 (d, J = 5 Hz, 1H), 7.41 (m, J = 8.2, 4H) ppm. 13C-NMR (75 MHz, CDCl3) δ = 16.35 (CH2), 26.28 (CH2), 41.90 (CH2), 48.86 (CH), 51.02 (CH), 55.62 (CH3), 62.62 (CH), 114.26 (2 x CH), 114.83 (2 x CH), 126.22 (2 x CH), 127.33 (CH), 128.75 (2 x CH), 140.42 (C), 142.64 (C), 152.00 (C), 213.83 (C) ppm. HPLC (64% ee) (Daicel Chiralpak IA, hexane/EtOH 90:10, flow rate 0.8 mL/min, λ= 203.4): major isomer: tR = 18.40 min.; minor isomer: tR = 19.43 min. HRMS calcd for C20H21NO2: 307.1572, found 307.1572.
(exo)-2-Allyl-3-phenyl-2-azabicyclo[2.2.2]octan-5-one (5m): Yield 30 mg (12%), yellow oil. Rf 0.50 (hexane/EtOAc 5:1). 1H-NMR (300 MHz, CDCl3): δ = 1.61 (m, 2H), 1.82 (m, 1H), 2.13 (m, 2H), 2.34 (s, 1H), 2.85 (d, J = 18 Hz, 1H), 3.10 (m, 2H), 3.35 (m, 1H), 3.94 (s, 1H), 5.08 (d, J = 9 Hz, 1H), 5.21 (d, J = 15 Hz, 1H), 5.80 (m, 1H), 7.29 (m, 2H), 7.37 (t, J = 6 Hz, 1H), 7.51 (d, J = 6 Hz, 2H) ppm. 13C-NMR (75 MHz, CDCl3) δ =16.38 (CH2), 27.74 (CH2), 38.47 (CH2), 50.30 (CH), 51.65 (CH), 56.80 (CH2), 63.82 (CH), 116.93 (CH2), 127.18 (CH), 127.29 (2 x CH), 128.35 (2 x CH), 136.19 (CH), 141.19 (C), 215.58 (C) ppm. HPLC (27% ee) (Daicel Chiracel ODH, hexane/2-propanol 99:1, flow rate 0.8 mL/min, λ= 207.6): major isomer: tR = 8.81 min.; minor isomer: tR = 9.26 min. HRMS calcd for C16H19NO: 241.14612, found: 241.145920.
(exo)-6-Allyl-7-phenyl-6-azabicyclo[3.2.2]nonan-8-one (5n): Yield 125 mg (49%), yellow oil. Rf 0.41 (hexane/EtOAc 10:1). 1H-NMR (300 MHz, CDCl3): δ = 1.44 (m, 1H), 1.57 (m, 1H), 1.71 (m, 2H), 1.84 (m, 1H), 1.97 (m, 1H), 2.25 (d, J = 18.4 Hz, 1H), 2.64 (d, J = 8 Hz, 1H), 2.82 (dd, J = 18.4, 4.8 Hz, 1H), 3.08 (dd, J = 14.4, 7.6 Hz, 1H), 3.16 (dd, J = 14.4, 2 Hz, 1H), 3.43 (m, 1H), 4.01 (s, 1H), 5.19 (m, 2H), 5.91 (m, 1H), 7.27 (m, 1H), 7.36 (t, J = 6 Hz, 2H), 7.48 (d, J = 6 Hz, 2H) ppm. 13C-NMR (75 MHz, CDCl3,) δ = 20.22 (CH2), 23.56 (CH2), 36.49 (CH2), 38.83 (CH2), 51.20 (CH), 55.04 (CH), 57.18 (CH2), 64.37 (CH), 117.18 (CH2), 127.11 (CH), 127.67 (2 x CH), 128.39 (2 x CH), 136.21 (CH), 141.31 (C), 214.52 (C) ppm. HPLC (32% ee) (Daicel Chiralpak IA, hexane/EtOH 90:10, flow rate 0.6 mL/min, λ= 211.0): major isomer: tR = 7.60 min.; minor isomer: tR = 9.47 min. HRMS calcd for C17H21NO: 255.1623, found: 255.1632.
ACKNOWLEDGEMENTS
We thank the Ministero de Educación y Ciencia (MEC-07-CTQ2007-61048) for financial support for this work. A FICYT doctoral fellowship to N. Q. is gratefully acknowledged.
References
1. For general reviews on asymmetric organocatalysis, see: (a) P. I. Dalko and L. Moisan, Angew. Chem. Int. Ed., 2001, 40, 3726; CrossRef (b) P. I. Dalko and L. Moisan, Angew. Chem. Int. Ed., 2004, 43, 5138; CrossRef (c) A. Berkessel and H. Gröger, Asymmetric Organocatalysis, Wiley-VCH, Weinheim, 2004; (d) M. J. Gaunt, C. C. C. Johansson, A. McNally, and N. T. Vo, Drug Discovery Today, 2007, 12, 8; CrossRef (e) P. I. Dalko, Enantioselective Organocatalysis, Wiley-VCH, Weinheim, 2007; CrossRef (f) H. Pellissier, Tetrahedron, 2007, 63, 9267; CrossRef For special issues on asymmetric organocatalysis, see: (g) K. N. Houk and B. List, Acc. Chem. Res., 2004, 37, 487; CrossRef (h) B. List and C. Bolm, Adv. Synth. Catal., 2004, 346, 1007; CrossRef (i) B. List, Chem. Rev., 2007, 107, 5413. CrossRef
2. For reviews on asymmetric aminocatalysis, see: (a) B. List, Synlett, 2001, 1675; CrossRef (b) B. List, Tetrahedron, 2002, 58, 5573; CrossRef (c) W. Notz, F. Tanaka, and C. F. Barbas III, Acc. Chem. Res., 2004, 37, 580; CrossRef (d) G. Lelais and D. W. C. MacMillan, Aldrichimica Acta, 2006, 39, 79; (e) B. List, Chem. Commun., 2006, 819; CrossRef (f) A. G. Doyle and E. N. Jacobsen, Chem. Rev., 2007, 107, 5713; CrossRef (g) A. Erkkilä, I. Majander, and P. M. Pihko, Chem. Rev., 2007, 107, 5416; CrossRef (h) S. Mukherjee, J. W. Yang, S. Hoffmann, and B. List, Chem. Rev., 2007, 107, 5471; CrossRef (i) P. Melchiorre, M. Marigo, A. Carlone, and G. Bártoli, Angew. Chem. Int. Ed., 2008, 47, 6138; CrossRef (j) D. W. C. MacMillan, Nature, 2008, 304. CrossRef
3. (a) B. List, R. A. Lerner, and C. F. Barbas III, J. Am. Chem. Soc., 2000, 122, 2395; CrossRef (b) K. Sakthivel, W. Notz, T. Bui, and C. F. Barbas III, J. Am. Chem. Soc., 2001, 123, 5260; CrossRef (c) A. B. Northrup and D. W. C. MacMillan, J. Am Chem. Soc., 2002, 124, 6798; CrossRef (d) L. Hoang, S. Bahmanyar, K. N. Houk, and B. List, J. Am Chem. Soc., 2003, 125, 16; CrossRef (e) C. Pidathala, L. Hoang, N. Vignola, and B. List, Angew. Chem. Int. Ed ., 2003, 42, 2785; CrossRef (f) D. E. Ward, V. Jheengut, and O. T. Akinnusi, Org. Lett., 2005, 7, 1181; CrossRef (g) Y. Hayashi, T. Sumiya, J. Takahashi, H. Gotoh, T. Urushima, and M. Shoji, Angew. Chem. Int. Ed., 2006, 45, 958; CrossRef (h) D. Enders and T. Gasperi, Chem. Commun., 2007, 88; CrossRef (i) L. Zu, H. Xie, J. Wang, and W. Wang, Org. Lett., 2008, 10, 1211. CrossRef
4. (a) B. List, P. Pojarliv, and H. J. Martin, Org. Lett., 2001, 3, 2423; CrossRef (b) J. M. Betancort and C. F. Barbas III, Org. Lett., 2001, 3, 3737; CrossRef (c) D. Enders and A. Seki, Synlett, 2002, 26; CrossRef (d) A. Melchiorre and K. A. Jorgensen, J. Org. Chem., 2003, 68, 4151; CrossRef (e) C. Palomo, A. Landa, A. Mielgo, M. Oiarbide, A. Puente, and S. Vera, Angew. Chem. Int. Ed., 2007, 46, 1; CrossRef (f) P. Dinér, M. Nielsen, M. Marigo, and K. A. Jorgensen, Angew. Chem. Int. Ed., 2007, 46, 1983; CrossRef (g) Y.-J. Cao, Y.-Y. Lai, X. Wang, Y.-J. Li, and W.-J. Xiao, Tetrahedron Lett., 2007, 48, 21. CrossRef
5. (a) B. List, J. Am. Chem. Soc., 2000, 122, 9336; CrossRef (b) B. List, P. Porjaliev, W. T. Biller, and H. J. Martin, J. Am. Chem. Soc., 2002, 124, 827; CrossRef (c) E. N. Wenzel and E. N. Jacobsen, J. Am. Chem. Soc., 2002, 124, 12964; CrossRef (d) A. Córdova, Synlett, 2003, 1651; CrossRef (e) Y. Hayashi, W. Tsuboi, I. Ashimine, T. Urushima, M. Shoji, and K. Sakai, Angew. Chem. Int. Ed., 2003, 42, 3677; CrossRef (f) A. Córdova, Chem. Eur. J., 2004, 10, 1987. CrossRef
6. (a) K. A. Ahnrendt, C. J. Borths, and D. W. C. MacMillan, J. Am. Chem. Soc., 2000, 122, 4243; CrossRef (b) R. Thayumanavan, B. Dhevalapàlly, K. Sakthivel, F. Tanaka, and C. F. Barbas III, Tetrahedron Lett., 2002, 43, 3817; CrossRef (c) D. B. Ramachary, N. S. Chowdari, and C. F. Barbas III, Tetrahedron Lett., 2002, 43, 6743; CrossRef (d) D. B. Ramachary, N. S. Chowdari, and C. F. Barbas III, Angew. Chem. Int. Ed., 2003, 42, 4233; CrossRef (e) D. B. Ramachary, K. Anebouselvy, N. S. Chowdari, and C. F. Barbas III, J. Org. Chem., 2004, 69, 5838. CrossRef
7. (a) T. C. Wabnitz, S. Saaby, and K. A. Jorgensen, Org. Biomol. Chem., 2004, 2, 828; CrossRef (b) F. A. Hernández-Juan, D. M. Cockfield, and D. J. Dixon, Tetrahedron Lett., 2007, 48, 1605; CrossRef (c) Y. Zhao, X.-J. Wang, and J.-T. Liu, Synlett, 2008, 1017; CrossRef (d) C. Gioia, A. Hauville, L. Bernardi, F. Fini, and A. Ricci, Angew. Chem. Int. Ed., 2008, 47, 9236; CrossRef (e) M. A. Terzidis, E. Dimitriadou, C. A. Tsoleridis, and J. Stephanidou-Stephanatou, Tetrahedron Lett., 2009, 50, 2174. CrossRef
8. (a) J. Barluenga, F. Aznar, M.-P. Cabal, and C. Valdés, J. Org. Chem., 1993, 58, 3391; CrossRef (b) J. Barluenga, F. Aznar, C. Ribas, C. Valdés, M. Fernández, M.-P. Cabal, and J. Trujillo, Chem. Eur. J., 1996, 2, 805; CrossRef (c) A.-B. García, C. Valdés, and M.-P. Cabal, Tetrahedron Lett., 2004, 44, 4357. CrossRef
9. (a) F. Aznar, A.-B. García, and M.-P. Cabal, Adv. Synth. Catal., 2006, 348, 2443; CrossRef (b) F. Aznar, A.-B. García, N. Quiñones, and M.-P. Cabal, Synthesis, 2008, 479. CrossRef
10. R. J. Sundberg and S. Q. Smith, The alkaloids, vol. 59, Academic Press 2002, p. 261.
11. (a) G. Babu and P. Perumal, Tetrahedron, 1998, 54, 1627; CrossRef (b) G. Shanti and P. T. Perumal, Synth. Commun., 2005, 35, 1319. CrossRef
12. (a) H. Liu, L.-F. Cun, A.-Q. Mi, Y.-Z. Jiang, and L.-Z. Gong, Org. Lett., 2006, 8, 6023; CrossRef (b) M. Rueping, and C. Azap, Angew. Chem., 2006, 45, 7832; CrossRef (c) U. Constantino, F. Fringuelli, M. Orrù, M. Nocchetti, O. Piermatti, and F. Pizzo, Eur. J. Org. Chem., 2009, 1214. CrossRef
13. H. Sundén, I. Ibrahem, L. Ericsson, and A. Córdova, Angew. Chem. Int. Ed., 2005, 44, 4877. CrossRef
14. (a) T. P. Loh, L. C. Feng, and J. Y. Yang, Tetrahedron Lett., 2002, 43, 8741; CrossRef (b) F. Tanaka, R. Thayumanavan, N. Mase, and C. F. Barbas III, Tetrahedron Lett., 2004, 45, 325. CrossRef
15. (a) P. Västillä, A. B. Zaitsev, J. Wettergreen, T. Privalov, and H. Adolfsson, Chem. Eur. J., 2006, 12, 3218; CrossRef (b) I. Ibrahem, G.-L. Zhao, and A. Córdova, Chem. Eur. J., 2007, 13, 683. CrossRef
16. All the reactions were conducted in a multicomponent fashion except for the cases in which the preformed aldimine gave better values of enantiomeric excess.
17. (a) Y. Yamamoto, N. Momiyama, and H. Yamamoto, J. Am. Chem. Soc., 2004, 126, 5962; CrossRef (b) H. Yamamoto and N. Momiyama, Chem. Commun., 2005, 3514. CrossRef
18. (a) D. B. Ramachary, N. S. Chowdari, and C. F. Barbas III, Synlett, 2003, 1910; CrossRef (c) D. B. Ramachary, and C. F. Barbas III, Chem. Eur. J., 2004, 10, 5323; CrossRef (d) D. B. Ramachary, Y. V. Reddy, and B. V. Prakash, Org. Biomol. Chem., 2008, 6, 719. CrossRef
19. I. Ibrahem, W. Zou, J. Casas, H. Sudén, and A. Córdova, Tetrahedron, 2006, 62, 357 and references cited therein about the si selectivity in Mannich reactions. CrossRef