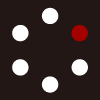
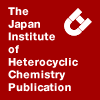
HETEROCYCLES
An International Journal for Reviews and Communications in Heterocyclic ChemistryWeb Edition ISSN: 1881-0942
Published online by The Japan Institute of Heterocyclic Chemistry
e-Journal
Full Text HTML
Received, 16th July, 2009, Accepted, 31st August, 2009, Published online, 1st September, 2009.
DOI: 10.3987/COM-09-S(S)56
■ Selective Formation of 5- or 6-Membered Rings, 1,3-Thiazolidin-4-one vs. 1,3-Thiazin-4-one, from Acridine Thiosemicarbazides by the Use of Ethyne Acid Esters
Ján Imrich,* Jana Tomaščiková, Ivan Danihel, Pavol Kristian, Stanislav Böhm, and Karel D. Klika
Institute of Chemistry, Faculty of Science, P. J. Šafárik University, Moyzesova 11, SK-041 67 Košice, Slovak Republic
Abstract
A set of 2-substituted 1-(9,10-dihydroacridin-9-ylidene) thiosemicarbazides 4a-d (H, methyl, ethyl, and n-butyl substituents) were treated with dimethyl acetylenedicarboxylate (DMAD) to yield 5-membered ring 1,3-thiazolidin-4-one products 5a-d. The methyl derivative 4b, upon reaction with methyl propiolate (MP), yielded a 6-membered thiazin-4-one ring product 7b. The structures of the methyl derivative products 5b and 7b were conclusively proven by NMR examinations and compared to X-ray crystallographic analysis. The ring−chain tautomerism of the thiosemicarbazides, the regio- and stereoselectivity of the reactions, the adopted s-cis conformations of the Z configurations of the products, and the prototropic tautomerism of all the compounds were all substantiated.INTRODUCTION
We have long been interested1−8 in the chemistry, the biological properties, and the potential Alzheimer’s disease treatments1f of acridine, in particular, when the substrate is conjugated with other heterocyclic moieties, e.g. oxadiazoles1b or thiazolidines2,3,6 amongst others. Acridine derivatives are notable for their intercalating1,2 and fluorescent properties9 with many being utilized as sensitive biomarkers or as chemotherapeutic agents.10 Thiazolidine entities, on the other hand, are known for their broad spectral range of biological activities.11 Acridinylthiosemicarbazides are especially interesting for their inherent proclivity towards spiro−open-chain tautomerism2−5 which can lead to an array of structures and, sometimes, resulting confusion regarding the identity of products arising from a reaction.4,5
Previously we had found that thiosemicarbazides or thioureas differ in their behavior towards bielectrophilic reagents in substitution reactions and hence the consequent cyclization to form a heterocyclic ring can yield unexpected or difficult to rationalize structures. For example, N-(anthracen-9-yl)-N'-ethylthiourea upon reaction with bromoacetic acid derivatives yielded regioisomeric 1,3-thiazolidin-4-one derivatives12 and the reactions of 1,1-dimethyl-4-(acridin-9-yl)-13 and 1-tert-butyl-4-(acridin-9-yl)thiosemicarbazides3 with dimethyl acetylenedicarboxylate (DMAD) to also yield 1,3-thiazolidin-4-one derivatives can be contrasted to the unexpected regioisomeric product5 from the reaction of 1-(9,10-dihydroacridin-9-ylidene)-2-methylthiosemicarbazide with methyl bromoacetate which arose by way of rearrangement of the substrate prior to reaction.
Herein we pursue our study of the reaction of thiosemicarbazides with DMAD2,3,13 and augment it by contrasting it with methyl propiolate (MP). This is because, based on previous results, it seemed plausible to us that control of 5- vs. 6-membered ring formation (i.e. 1,3-thiazolidin-4-one vs. 1,3-thiazin-4-one products, respectively) using ethyne acid esters could be accomplished by adduct reagent selection. The
formation of 5-membered rings using DMAD occurs because the addition of S to the triple bond is followed by the kinetically-controlled displacement by N at the proximal carboxymethyl group. It thus seemed likely that the formation of a 6-membered, 1,3-thiazin-4-one ring should result if the C3 fragment synthon is utilized rather as an unsymmetrical entity whereby the opposite ends of the reagent are unreactive to each one of the nucleophiles, i.e. one end unreactive towards N and the other end towards S, thus facilitating a C3 fragment synthon to result in the formation of a 6-membered ring rather than a 5-membered one. This is easily perceived by the use of MP where only the β carbon is susceptible to S attack and the sole carboxymethyl group is at the other end of the triple bond. We now report on the success of this elegant-in-its-simplicity synthetic strategy by the reaction of 1-(9,10-dihydroacridin-9-ylidene)-2-methylthiosemicarbazide with DMAD and MP in addition to the reaction of 2-(un)substituted 1-(9,10-dihydroacridin-9-ylidene)thiosemicarbazides (ethyl and n-butyl substituents) with DMAD. The methyl-substituted products were emphatically proven by single crystal X-ray diffraction analysis complemented by thorough 1H, 13C, and 15N NMR analysis and supplemented by IR. In addition, comprehensive modeling calculations on these systems have been reported14 elsewhere.
RESULTS AND DISCUSSION
Synthesis
The required thiosemicarbazides 4a-d for reaction with the ethyne acid esters DMAD and MP were generated by treating 9-isothiocyanatoacridine15 (1) with methyl- (b), ethyl- (c), and n-butylhydrazine (d) as well as unsubstituted hydrazine (a) itself. The treatment of 1 with methylhydrazine (b) has been performed previously4,5 and thus it is known that, firstly, attack by the α nitrogen of methylhydrazine (b) onto the isothiocyanate carbon occurs preferentially and, secondly, the initial product 2b undergoes rearrangement first to the spiro structure 3b and then onto the other open-chain form 4b (Scheme 1).
The final state is an equilibrium dominated by the latter open-chain form 4b (96% in DMSO) but also present is a minor amount of the spiro tautomer 3b (4% in DMSO). The unsubstituted compound 2a (R = H), undergoes a similar rearrangement5 though the equilibrium in this case is even more strongly biased−effectively 100%−towards the open-chain form 4a.4 Similar results−the reaction course and tautomeric equilibria based on consistency with NMR expectations−were obtained when using ethyl- (c) and n-butylhydrazine (d), though in these cases the amount of the spiro forms was estimated to be slightly lower than 3b at less than 3% in each case. With rare exception,3 the acridine nitrogen (N-10' according to the atom numbering given below) has an extremely strong tendency to retain a proton1b,f,2,4−7,16 submitting thus dihydroacridine ring structure. This case was no exception, i.e. the NH-10',N-11' tautomers of 4b-d were determined by standard methods of appraisal (diagnostic NMR chemical shifts, e.g. C-4a' and C-10a' at ca. 140 ppm, in line with previous reports1b,f,2,4−7 and 2D HMBC correlations). Additionally, the NH-10',N-11' tautomer was also confirmed by X-ray analysis in the case of 4a.17 Alternative N-10',NH-11' tautomeric forms of 4b-d possessing aromatic acridine ring, which could be easily recognized by typical 13C chemical shifts of the bridge acridine carbons C-4a' and C-10a' at ca. 150 ppm, were not observed.
Compounds 4b−d upon reaction with DMAD (4a is discussed separately below) provided the 1,3-thiazolidin-4-one compounds 5b−d (Scheme 2) as the major products rather than the 1,3- thiazin-4-one compounds 6b−d.
The structure of the major product was anticipated based on similarity to previous results2,3 and a presumed course of reaction. Though the NMR spectra of the products were tacitly consistent with this assumption, by NMR it is not trivial to emphatically distinguish between the two candidate structures 5 and 6 due to a dearth of Hs in the 1,3-thiazolidin-4-one/1,3-thiazin-4-one ring and attendant side-chain. Thus, to firmly establish the identity of the products in terms of whether either a 5- or a 6-membered ring had been formed (5 or 6, respectively), X-ray diffraction analysis was performed on suitable crystals harvested from the methyl-substituted derivative. This analysis categorically provided the structure of the product as 5b with attendant fine structural elements, viz. the Z configuration of the C5=C6 double bond and the s-cis conformation of the C5=C6−C7=O7 segment (Scheme 2).17 The Z configuration of the C5=C6 double bond−which results from the course of the reaction, viz. concerted addition−and the s-cis conformation of the C5=C6−C7=O7 segment for 5 were both anticipated based on previous X-ray-derived results regarding the structure of a reaction product of 4-(acridin-9-yl)-1-(tert-butyl)thiosemicarbazide with DMAD.3
Interestingly, the Hs in each pair of methylenes in the side-chains of 5c and 5d were chemically distinct due to restricted rotation, though only for the H-1''s was the disparity large (ca. 1 ppm). With heating to 80 °C, the H-1'' signals of 5d started to coalesce but resumed their normal appearance upon lowering the temperature back to 25 °C. The inequivalence of geminal protons of the methylene group was also observed for 4c and 4d where the disparity was even greater for the H-1''s (1.3 and 1.5 ppm, respectively).
In addition to the isolated and identified major product 5b (ca. 97%; H-6, 6.68 ppm), there were two more compounds present in the crude reaction mixture, a minor one (ca. 2%; H-5, 6.89 ppm) and a trace one (less than 1%; H-6, 6.63 ppm), which were evidenced also by their NH, NMe, OMe, and some acridine 1H NMR signals. The trace component was shown by variable-temperature and saturation transfer NMR experiments to exchange with the major species, probably due to an isomerization about the C6-C7 bond (Scheme 2), therefore it was assigned as the s-trans conformer of 5b on this basis (not depicted). The DFT calculated14 value of ΔG between these two species implies a population ratio of 95:5, a ratio close to observation. The minor species, which does not exchange with the major, is tentatively assigned as the 1,3-thiazin-4-one compound 6b, with chemical shifts consistent with DFT calculated14 values, most notably H-5 which is deshielded by 0.21 ppm relative to H-6 in 5b (DFT calculated difference, 0.35 ppm14).
Compound 4a, the unsubstituted parent compound, was also treated with DMAD (Scheme 3). Due to another reactive centre NH-12' besides the NH2 nitrogen present in this molecule, two reaction pathways to yield either 2-imino-3-substituted amino-1,3-thiazolidin-4-one structures 92,3 or substituted 2-hydrazino -1,3-thiazolin-4-one structure 5a could be expected. Moreover, the product 5a, in contrast to 5b−d, can undergo prototropic tautomerization with H-12' moving into the ring onto the nitrogen N-3. The resultant species 10 can be present as either an E or a Z isomer about the new imine double bond C2=N12'. Similarly, for the alternate product 9, E and Z isomers are possible. To distinguish between the
various possibilities, the five candidate structures were geometrically optimized by DFT14 and their 13C NMR chemical shifts calculated14. As shown in Table 1, the lowest energy found pointed to a candidate structure 10Z but further evidence for this assumption was necessary and has been obtained from experimental NMR data. In solution NMR spectra, the analyzed product of the reaction of 4a with DMAD exhibited two sets of slightly exchanged-broadened NMR signals indicating that two interconverting species, major and minor, were present. These two species possessed very similar chemical shifts for the corresponding signals in both the 1H and 13C spectra, accordingly, with the most notable feature the strong shielding of C-4 (C=O, ca. 10 ppm) and C-2 (C=N, ca. 20 ppm) relative to the analogous nuclei in 5b−d. Only two NH NMR signals of the major species have been observed, those of the minor one were not recognized. The first signal at 10.85 ppm assigned via three-bond HMBC crosspeaks with acridine C-4',5' and C-8a',9a' carbons belongs to acridine NH-10' proton. This finding and observed typical NMR inequivalence of the outer rings of the acridine moiety both confirm a NH-10',N-11' (9,10-dihydroacridin-9-ylidene) tautomeric structure of the acridine skeleton. Other labile NH proton at 12.82 ppm not associated with the acridine moiety was too broad to provide any correlations.
Since two very similar structures were present in solution, this suggested that either the pair of structures 10 or the pair of structures 9 isomers were the responsible participants. The most decisive chemical shifts
are C-2, C-4, and C-5 which exclude structure 5a and the chemical shift of C-9' which eliminates the pair of structures 9. Additionally, the experimentally observed chemical shift difference between C-1' and C-8' also helps to exclude the pair of structures 9, as does the chemical shifts for C-2 and C-4 based on the incompatibility with 9E. Thus, the results clearly indicate that the pair 10Z and 10E are the products of the reaction and, furthermore, that the major species was the Z isomer based on the calculated14 energy difference of 2.20 kcal mol−1 between the two.
The likely course of the reaction2 of 4 with DMAD leading to the formation of the 5-membered rings is that the sulfur atom of 4, as thiol after prototropic tautomeric shift, attacks first at one of the ethynyl carbons to yield S-alkylated intermediate, e.g. 1,4-dimethyl-2-{[N'-(9,10-dihydroacridin-9-ylidene amino)carbamimidoyl]sulfanyl}but-2-enedioate in the case of 4a. The products yielded are determined by
the N,S-tautomeric equilibrium of two tautomeric forms A or B (Scheme 4). It is expected that the conjugated imine, tautomeric structure A, will dominate strongly over the non-conjugated, terminal imine tautomeric structure B, thus leading steadfastly to the tautomeric products 5a, 10Z/10E instead of the alternate products 9. This is borne out by calculations14 favoring structure A over tautomeric structure B by 7.30 kcal mol−1.
Within intermediate of 4a, the terminal nitrogen (which is the only reactive nitrogen in the case of 4b−d) has a choice of two carboxyls to attack to form a new heterocyclic ring (the N-11' nitrogen is precluded from participating in the reaction because of the strong retention of the other labile H by N-10'). Preference is dictated by proximity and thus for kinetic reasons the carboxyl nearest the site of attachment succumbs to nucleophilic displacement by the terminal nitrogen, yielding the 1,3-thiazolidin-4-one ring of 5. The observed product 5 is also favored thermodynamically14 over the alternative product 6 by a sizeable amount, though the course of the reaction is not expected to be reversible. Thus the reaction follows the same course (and regioselectivity) as previously described2,3,13 with the same stereochemical consequence.
Compounds 4b−d were also treated with MP but only in the case of the methyl derivative 4b was reaction successful and provided 1,3-thiazin-4-one 7b as the only product without any sign of the 1,3-thiazolidin-4-one compound 8b (Scheme 5). In this reaction, the replacement of one carboxymethyl
group in 5b and 6b with H renders the distinction between 5- and 6-membered ring formation, viz. 8b vs. 7b, respectively, trivial since the comparison reduces to evaluation between a geminal pair of alkenyl Hs (=CH2, 8b) and a vicinal pair of alkenyl Hs (CH=CH, 7b). The ring size in this case was proved by the 13C NMR spectra where two methine carbons were observed for a 6-membered ring as opposed to the one quarternary carbon and one methylene carbon for a 5-membered ring formation. The acridine NH-10',N-11' tautomer as depicted in Scheme 5 is based on NMR chemical shift assignments and HMBC correlations with proton NH-10'. For comparative purposes, even though the structural assignment was unequivocal by NMR, X-ray analysis was also performed on suitable crystals of 7b.17
Concerning the course of the reaction of 4b with MP leading to the formation of the 6-membered ring 1,3-thiazin-4-one product 7b, the sulfur atom, again as thiol after tautomerization, initially attacks an ethynyl carbon of MP and does so preferentially at the β carbon since this is the β carbon of an α,β-unsaturated carbonyl. Addition across an unsaturated system, i.e. attack at the α carbon, without the assistance of a carbonyl would not be feasible even though the atomic charge on the α carbon is considerably more positive than the β carbon (+0.387 vs. −0.263 for the β carbon with the charge of the hydrogen summed in, data available from DFT calculations14). The terminal nitrogen attacks the sole carboxy carbon of MP, to displace the methoxy group yielding the 1,3-thiazin-4-one ring of 7b. The reaction mechanism and progress for MP, apart from being constrained by limited reaction sites, is otherwise akin to that for DMAD. Of note, 7b has been calculated14 to be thermodynamically less stable than the other potential product 8b by a considerable amount.
Both of these reactions involving the sulfur nucleophile should be concerted with concomitant addition of H from an external source given the stereoselectivity obtained, viz. the Z configuration, for 5b−d (confirmed by X-ray17). Moreover, it is clear that these reactions are kinetically driven and not thermodynamically controlled. Thus our reasoning to gain control over the ring formation size was successful simply based on the concept of regioselectivity via reagent selection.
Structures of the compounds 4a, 5b and 7b were also determined by means of X-ray analysis and all data in details will be published elsewhere.17
EXPERIMENTAL
General
NMR chemical shift assignments were rendered by standard application of 1-D and 2-D correlation spectra (H,H-COSY, TOCSY, NOESY, gHSQC, gHMBC) on a NMR spectrometer Varian Mercury Plus operating at 400.13 MHz for 1H and 100.61 MHz for 13C. 1H,15N-HSQC and 1H,15N-HMBC NMR spectra were taken on a NMR spectrometer Varian Inova operating at 599.78 MHz for 1H and 60.78 MHz for 15N. Variable temperature 1H and 13C NMR measurements in the range +25 to +80 °C and exchange spectroscopy were employed to study the isomerisation of 5b. A description of the NMR methodology has been described elsewhere.7a,8,18,19 Tetramethylsilane was used as an internal chemical shift reference for both 1H and 13C nuclei (δTMS = 0.00 ppm for both nuclei) whilst nitromethane was used as an external chemical shift reference for 15N (δnitro = 0.00 ppm).
Calculations
Quantum chemical calculations were carried out within the framework of the DFT method according to the original proposal at the B3LYP/6-311++G(2d,2p) level of theory20 using the Gaussian 03 program.21
General procedure3,4 for the preparation of thiosemicarbazides 4a−d
To a solution of 9-isothiocyanatoacridine15 (1, 300 mg, 1.27 mmol) in CH2Cl2 (2–3 mL), the appropriate hydrazine oxalate (1.27 mmol) and triethylamine (0.17 mL, 1.27 mmol) were added. The reaction was stirred at room temperature until completion (monitored by TLC, toluene−acetone 5:2). The precipitate that had formed was filtered off, washed with a minimum of CH2Cl2 and then dried.
1-(9,10-Dihydroacridin-9-ylidene)thiosemicarbazide (4a). The spectral data of the compound were found to be consistent with literature values.4,5
1-(9,10-Dihydroacridin-9-ylidene)-2-methylthiosemicarbazide (4b). The spectral data of the compound were found to be consistent with literature values.4,5
1-(9,10-Dihydroacridin-9-ylidene)-2-ethylthiosemicarbazide (4c). Yield 80%; mp 211−213 °C; 1H NMR (400 MHz, DMSO-d6, 25 °C) δ: 1.09 (t, 3H, H-2'', J = 7.0 Hz), 3.30 (broad, 1H, H-1a''), 4.59 (broad, 1H, H-1b''), 6.90 and 7.42 (broad, 2H, NH2), 7.08 (dd, 1H, H-2', J = 8.4, 6.8 Hz), 7.14 (dd, 1H, H-7', J = 8.0, 7.2 Hz), 7.34 (d, 1H, H-5', J = 7.6 Hz), 7.41 (d, 1H, H-4', J = 8.4 Hz), 7.54 (m, 1H, H-3'), 7.58 (m, 1H, H-6'), 8.15 (d, 1H, H-1', J = 8.4 Hz), 8.48 (d, 1H, H-8', J = 8.0 Hz), 11.28 (s, 1H, H-10'); 13C NMR (100 MHz, DMSO-d6, 25 °C) δ: 12.1 (C-2''), 46.6 (C-1''), 113.7 (C-9a'), 115.9 (C-5'), 117.3 (C-4'), 118.0 (C-8a'), 120.4 (C-2'), 121.1 (C-7'), 126.3 (C-8'), 127.2 (C-1'), 131.6 (C-6'), 132.6 (C-3'), 138.2 (C-10a'), 140.3 (C-4a'), 156.5 (C-9'), 176.5 (C-2); Anal. Calcd for C16H16N4S (296.39): C, 64.84; H, 5.44; N, 18.90. Found: C, 64.51; H, 5.19; N, 18.65%.
1-(9,10-Dihydroacridin-9-ylidene)-2-n-butylthiosemicarbazide (4d). Yield 74%; mp 168−170 °C; 1H NMR (400 MHz, DMSO-d6, 25 °C) δ: 0.77 (t, 3H, H-4'', J = 7.2 Hz), 1.18 (m, 1H, H-3a''), 1.19 (m, 1H, H-3b''), 1.59 (m, 1H, H-2a''), 1.63 (m, 1H, H-2b''), 3.16 (m, 1H, H-1a''), 4.63 (m, 1H, H-1b''), 6.88 and 7.43 (broad, 2H, NH2), 7.07 (dd, 1H, H-2', J = 8.0, 7.2 Hz), 7.14 (dd, 1H, H-7', J = 8.0, 7.2 Hz), 7.33 (d, 1H, H-5', J = 8.0 Hz), 7.41 (d, 1H, H-4', J = 8.0 Hz), 7.56 (m, 1H, H-3'), 7.58 (m, 1H, H-6'), 8.13 (d, 1H, H-1', J = 8.0 Hz), 8.46 (d, 1H, H-8', J = 8.0 Hz), 11.26 (s, 1H, H-10'); 13C NMR (100 MHz, DMSO-d6, 25 °C) δ: 13.7 (C-4''), 19.7 (C-3''), 29.0 (C-2''), 51.3 (C-1''), 113.7 (C-9a'), 115.9 (C-5'), 117.3 (C-4'), 118.1 (C-8a'), 120.4 (C-2'), 121.1 (C-7'), 126.2 (C-8'), 127.3 (C-1'), 131.6 (C-6'), 132.6 (C-3'), 138.2 (C-10a'), 140.3 (C-4a'), 156.1 (C-9'), 176.5 (C-2); Anal. Calcd for C18H20N4S (324.45): C, 66.63; H, 6.21; N, 17.27. Found: C, 66.27; H, 5.94; N, 16.95%.
General procedure for the reaction of thiosemicarbazides 4a−d with DMAD
To a solution of the appropriate thiosemicarbazide (0.30 mmol) in MeOH (1−2 mL), DMAD (0.05 mL, 0.40 mmol) was added dropwise at room temperature. The reaction mixture was stirred for 24 h until completion (monitored by TLC, toluene−acetone 5:2). The precipitant was then filtered off, washed with a small amount of MeOH and Et2O followed by recrystallization from MeOH.
(Z)-Methyl 2-{2-[2-(9,10-dihydroacridin-9-yliden)hydrazono]-4-oxothiazolidin-5-yliden}acetate (10Z). Major species in solution (87%). Yield 83%; mp 282−285 °C; IR ν: 1685 (C7=O7), 1647 (C4=O4) cm−1; 1H NMR (400 MHz, DMSO-d6, 25 °C) δ: 3.80 (s, 3H, H-9), 6.66 (s, 1H, H-6), 7.03 (ddd, 1H, H-2', J = 8.0, 7.2, 1.2 Hz), 7.17 (ddd, 1H, H-7', J = 8.0, 7.2, 1.0 Hz), 7.25 (m, 1H, H-5'), 7.29 (dd, 1H, H-4', J = 8.4, 1.2 Hz), 7.51 (ddd, 2H, H-3',6', J = 8.4, 7.2, 1.2 Hz), 8.31 (dd, 1H, H-1', J = 8.0, 1.2 Hz), 9.36 (dd, 1H, H-8', J = 8.0, 1.2 Hz), 10.85 (s, 1H, H-10'), 12.82 (s, 1H, H-3); 13C NMR (100 MHz, DMSO-d6, 25 °C) δ: 52.3 (C-9), 113.2 (C-6), 115.5 (C-9a'), 115.8 (C-5'), 116.3 (C-4'), 119.2 (C-8a'), 119.7 (C-2'), 121.1 (C-7'), 125.1 (C-8'), 131.0, 131.6 (C-3',6'), 131.9 (C-1'), 138.4 (C-10a'), 140.4 (C-4a'), 143.6 (C-5), 148.3 (C-9'), 155.0 (C-2), 165.5 (C-4), 166.1 (C-7); Anal. Calcd for C19H14N4O3S (378.41): C, 60.31; H, 3.73; N, 14.81. Found: C; 59.98; H, 3.48; N, 14.56%.
(E)-Methyl 2-{2-[2-(9,10-dihydroacridin-9-yliden)hydrazono]-4-oxothiazolidin-5-yliden}acetate (10E). Minor species in solution (13%). 1H NMR (400 MHz, DMSO-d6, 25 °C) δ: 3.81 (s, 3H, H-9), 6.75 (s, 1H, H-6), 7.04–7.28 (overlapped m, 4H, H-2',4',5',7'), 7.42 (m, 2H, H-3',6'), 8.80 (d, 1H, H-1', J = 7.6 Hz), 9.15 (d, 1H, H-8', J = 8.8 Hz); 13C NMR (100 MHz, DMSO-d6, 25 °C) δ: 52.4 (C-9), 138.5 (C-10a'), 140.5 (C-4a'), 166.3 (C-7).
Methyl 2-{2-[2-(9,10-dihydro-9-acridinyliden)-1-methylhydrazino]-4-oxo-4,5-dihydro-1,3-thiazol-5-yliden}acetate (5b). Yield 94%; mp 291−293 °C; ESI-MS: m/z = 393 (100%, [M + H+]), 361 (25, [M + H+ − CH3OH]), 193 (55, [Acr=N]+), 166 (38, [M + H+ − [AcrNH2] − CH3OH]); IR ν: 1711 (C7=O7), 1670 (C4=O4) cm−1; 1H NMR (400 MHz, DMSO-d6, 25 °C) δ: 3.52 (s, 3H, H-1''), 3.72 (s, 3H, H-9), 6.68 (s, 1H, H-6), 7.21 (m, 1H, H-2'), 7.29 (m, 1H, H-7'), 7.47 (d, 1H, H-5', J = 8.0 Hz), 7.53 (d, 1H, H-4', J = 8.8 Hz), 7.69 (m, 1H, H-3'), 7.71 (m, 1H, H-6'), 7.96 (d, 1H, H-1', J = 8.4 Hz), 8.44 (d, 1H, H-8', J = 8.0 Hz), 11.73 (s, 1H, H-10'); 13C NMR (100 MHz, DMSO-d6, 25 °C) δ: 38.8 (C-1''), 52.3 (C-9), 112.3 (C-9a'), 115.1 (C-6), 116.6 (C-5'), 116.7 (C-8a'), 118.0 (C-4'), 121.3 (C-2'), 122.0 (C-7'), 125.2 (C-8'), 126.5 (C-1'), 132.6 (C-6'), 133.3 (C-3'), 138.1 (C-10a'), 140.4 (C-4a'), 148.6 (C-5), 158.1 (C-9'), 166.2 (C-7), 174.4 (C-2), 176.6 (C-4); 15N NMR (40 MHz, DMSO-d6, 25 °C) δ: −262.0 (N-10'), −225.1 (N-12'), −98.0 (N-11'); Anal. Calcd for C20H16N4O3S (392.43): C, 61.21; H, 4.11; N, 14.28. Found: C, 60.87; H, 4.04; N, 13.98%.
Methyl 2-{2-[2-(9,10-dihydro-9-acridinyliden)-1-ethylhydrazino]-4-oxo-4,5-dihydro-1,3-thiazol-5-yliden}acetate (5c). Yield 44%; mp 293−296 °C; IR ν: 1716 (C7=O7), 1674 (C4=O4) cm−1; 1H NMR (400 MHz, DMSO-d6, 25 °C) δ: 1.28 (t, 3H, H-2'', J = 7.2 Hz), 3.58 (broad, 1H, H-1a''), 3.70 (s, 3H, H-9), 4.54 (broad, 1H, H-1b''), 6.66 (s, 1H, H-6), 7.19 (dd, 1H, H-2', J = 8.4, 7.2 Hz), 7.29 (dd, 1H, H-7', J = 8.0, 7.2 Hz), 7.48 (d, 1H, H-5', J = 8.8 Hz), 7.53 (d, 1H, H-4', J = 8.8 Hz), 7.69 (m, 1H, H-3'), 7.72 (m, 1H, H-6'), 8.00 (d, 1H, H-1', J = 8.4 Hz), 8.48 (d, 1H, H-8', J = 8.0 Hz), 11.74 (s, 1H, H-10'); 13C NMR (100 MHz, DMSO-d6, 25 °C) δ: 12.3 (C-2''), 48.1 (C-1''), 52.2 (C-9), 112.5 (C-9a'), 114.9 (C-6), 116.5 (C-5'), 116.8 (C-8a'), 118.0 (C-4'), 121.2 (C-2'), 121.9 (C-7'), 125.3 (C-8'), 126.3 (C-1'), 132.6 (C-6'), 133.2 (C-3'), 138.1 (C-10a'), 140.3 (C-4a'), 148.4 (C-5), 158.9 (C-9'), 166.1 (C-7), 173.4 (C-2), 176.6 (C-4); Anal. Calcd for C21H18N4O3S (406.46): C, 62.06; H, 4.46; N, 13.78. Found: C, 61.76; H, 4.30; N, 13.57%.
Methyl 2-{2-[2-(9,10-dihydro-9-acridinyliden)-1-n-butylhydrazino]-4-oxo-4,5-dihydro-1,3-thiazol-5-yliden}acetate (5d). Yield 38%; mp 265−268 °C; 1H NMR (400 MHz, DMSO-d6, 25 °C) δ: 0.83 (t, 3H, H-4'', J = 7.2 Hz), 1.30 (m, 2H, H-3''), 1.72 (m, 2H, H-2''), 3.47 (broad, 1H, H-1a''), 3.70 (s, 3H, H-9), 4.48 (broad, 1H, H-1b''), 6.66 (s, 1H, H-6), 7.19 (dd, 1H, H-2', J = 8.0, 6.4 Hz), 7.30 (dd, 1H, H-7', J = 7.6, 6.8 Hz), 7.48 (d, 1H, H-5', J = 8.0 Hz), 7.53 (d, 1H, H-4', J = 8.4 Hz), 7.68 (m, 1H, H-3'), 7.71 (m, 1H, H-6'), 7.99 (d, 1H, H-1', J = 8.0 Hz), 8.47 (d, 1H, H-8', J = 7.6 Hz), 11.75 (s, 1H, H-10'); 13C NMR (100 MHz, DMSO-d6, 25 °C) δ: 13.5 (C-4''), 19.5 (C-3''), 29.3 (C-2''), 52.4 (C-9), 52.6 (C-1''), 112.5 (C-9a'), 115.1 (C-6), 116.7 (C-5'), 116.9 (C-8a'), 118.2 (C-4'), 121.4 (C-2'), 122.1 (C-7'), 125.4 (C-8'), 126.5 (C-1'), 132.8 (C-6'), 133.4 (C-3'), 138.2 (C-10a'), 140.4 (C-4a'), 148.6 (C-5), 158.6 (C-9'), 166.3 (C-7), 173.7 (C-2), 176.7 (C-4); Anal. Calcd for C23H22N4O3S (434.51): C, 63.58; H, 5.10; N, 12.89. Found: C, 63.22; H, 4.93; N, 12.78%.
Preparation of 2-[2-(9,10-dihydroacridin-9-yliden)-1-methylhydrazino]-4H-1,3-thiazin-4-one (7b)
To a solution of thiosemicarbazide 4b (0.30 mmol) in MeOH (1−2 mL), MP (0.05 mL, 0.60 mmol) was added dropwise at room temperature. The reaction mixture was stirred at room temperature for 24 h until completion (monitored by TLC, toluene−acetone 5:2). The precipitant was then filtered off, washed with a small amount of MeOH and Et2O followed by recrystallization from MeOH. Yield 51%; mp 302−304 °C; IR ν: 1628 (C4=O4) cm−1; 1H NMR (400 MHz, DMSO-d6, 25 °C) δ: 3.25 (s, 3H, H-1''), 6.45 (d, 1H, H-5, J = 10.2 Hz), 7.17 (m, 1H, H-2'), 7.23 (m, 1H, H-7'), 7.41 (d, 1H, H-5', J = 8.0 Hz), 7.47 (d, 1H, H-4', J = 8.0 Hz), 7.64 (m, 2H, H-3',6'), 7.76 (d, 1H, H-6, J = 10.2 Hz), 8.08 (dd, 1H, H-1', J = 8.4, 0.8 Hz), 8.46 (dd, 1H, H-8', J = 8.4, 0.8 Hz), 11.46 (s, 1H, H-10'); 13C NMR (100 MHz, DMSO-d6, 25 °C) δ: 37.0 (C-1''), 113.0 (C-9a'), 116.3 (C-5'), 117.4 (C-8a'), 117.5 (C-4'), 118.9 (C-5), 120.8 (C-2'), 121.6 (C-7'), 125.2 (C-8'), 126.8 (C-1'), 132.1 (C-6'), 132.9 (C-3'), 136.3 (C-6), 138.1 (C-10a'), 140.3 (C-4a'), 156.6 (C-9'), 164.1 (C-2), 167.3 (C-4); Anal. Calcd for C18H14N4OS (334.40): C, 64.65; H, 4.22; N, 16.75. Found: C, 64.32; H, 4.12; N, 16.56%.
SUPPORTING INFORMATION
Crystallographic tables (selected bond lengths, bond angles, and dihedral angles) and X-ray-derived xyz coordinates for compounds 4a, 5b, and 7b and additional packing figures for compounds 5b and 7b are available upon request from the authors.
ACKNOWLEDGEMENTS
Financial support for this work by the Slovak Grant Agency VEGA (Grant No. 1/0476/08), Slovak State NMR Program (Grant No. 2003SP200280203) and Turun Yliopistosäätiö is gratefully acknowledged. We also thank the Center for Scientific Computing (CSC) for computational support and services.
References
1. a) D. Sabolová, M. Kožurková, P. Kristian, I. Danihel, D. Podhradský, and J. Imrich, Int. J. Biol. Macromol., 2006, 38, 94; CrossRef b) Z. Fröhlichová, J. Tomaščiková, J. Imrich, P. Kristian, I. Danihel, S. Böhm, D. Sabolová, M. Kožurková, and K. D. Klika, Heterocycles, 2009, 77, 1019; CrossRef c) M. Kožurková, D. Sabolová, H. Paulíková, L. Janovec, P. Kristian, M. Bajdichová, J. Buša, D. Podhradský, and J. Imrich, Int. J. Biol. Macromol., 2007, 41, 415; CrossRef d) M. Kožurková, D. Sabolová, L. Janovec, J. Mikeš, J. Kovaľ, J. Ungvarský, M. Štefanišinová, P. Fedoročko, P. Kristian, and J. Imrich, Bioorg. Med. Chem., 2008, 16, 3976; CrossRef e) Z. Gažová, A. Bellová, Z. Daxnerová, J. Imrich, P. Kristian, J. Tomaščiková, J. Bágeľová, D. Fedunová, and M. Antalík, Eur. Biophys. J., 2008, 37, 1261; CrossRef f) S. Hamuľaková, P. Kristian, D. Jun, K. Kuča, J. Imrich, I. Danihel, S. Böhm, and K. D. Klika, Heterocycles, 2008, 76, 1219. CrossRef
2. J. Tomaščiková, J. Imrich, I. Danihel, S. Böhm, P. Kristian, J. Pisarčíková, M. Sabol, and K. D. Klika, Molecules, 2008, 13, 501. CrossRef
3. J. Tomaščiková, I. Danihel, S. Böhm, J. Imrich, P. Kristian, I. Potočňák, J. Čejka, and K. D. Klika, J. Mol. Struct., 2008, 875, 419. CrossRef
4. K. D. Klika, E. Balentová, J. Bernát, J. Imrich, M. Vavrušová, E. Kleinpeter, K. Pihlaja, and A. Koch, J. Heterocycl. Chem., 2006, 43, 633. CrossRef
5. K. D. Klika, E. Balentová, J. Bernát, J. Imrich, M. Vavrušová, K. Pihlaja, A. Koch, E. Kleinpeter, A. Kelling, and U. Schilde, ARKIVOC, 2006, (xvi), 93.
6. K. D. Klika, J. Bernát, J. Imrich, I. Chomča, R. Sillanpää, and K. Pihlaja, J. Org. Chem., 2001, 66, 4416. CrossRef
7. a) E. Balentová, J. Imrich, J. Bernát, L. Suchá, M. Vilková, N. Prónayová, P. Kristian, K. Pihlaja, and K. D. Klika, J. Heterocycl. Chem., 2006, 43, 645; CrossRef b) J. Bernát, E. Balentová, P. Kristian, J. Imrich, E. Sedlák, I. Danihel, S. Böhm, N. Prónayová, K. Pihlaja, and K. D. Klika, Coll. Czech. Chem. Commun., 2004, 69, 833; CrossRef c) K. D. Klika, J. Imrich, M. Vilková, J. Bernát, and K. Pihlaja, J. Heterocycl. Chem., 2006, 43, 739; CrossRef d) P. Kristian, E. Balentová, J. Bernát, J. Imrich, E. Sedlák, I. Danihel, S. Böhm, N. Prónayová, K. D. Klika, K. Pihlaja, and J. Baranová, Chem. Pap. - Chem. Zvesti, 2004, 58, 268.
8. Z. Fröhlichová, J. Imrich, I. Danihel, P. Kristian, S. Böhm, D. Sabolová, M. Kožurková, O. Hritzová, B. Horváth, T. Bušová, and K. D. Klika, Spectrochim. Acta, Part A, 2009, 73, 238. CrossRef
9. J. E. Sinsheimer, V. Jagodic, J. Polak, D. D. Hong, and J. A. Burckhalter, J. Pharm. Sci., 1975, 64, 925. CrossRef
10. M. Demeunynck, F. Charmantray, and A. Martelli, Curr. Pharm. Des., 2001, 7, 1703. CrossRef
11. S. P. Singh, S. S. Parmar, K. Raman, and V. I. Stenberg, Chem. Rev., 1981, 81, 175. CrossRef
12. a) K. D. Klika, L. Janovec, J. Imrich, G. Suchár, P. Kristian, R. Sillanpää, and K. Pihlaja, Eur. J. Org. Chem., 2002, 1248; CrossRef b) K. D. Klika, P. Valtamo, L. Janovec, G. Suchár, P. Kristian, J. Imrich, H. Kivelä, J. Alföldi, and K. Pihlaja, Rapid Commun. Mass Spectrom., 2004, 18, 87; CrossRef c) L. Janovec, S. Böhm, I. Danihel, J. Imrich, P. Kristian, and K. D. Klika, Collect. Czech. Chem. Commun., 2007, 72, 1435. CrossRef
13. J. Tomaščiková, J. Imrich, I. Danihel, S. Böhm, and P. Kristian, Collect. Czech. Chem. Commun., 2007, 72, 347. CrossRef
14. S. Böhm, J. Tomaščiková, J. Imrich, I. Danihel, P. Kristian, H. Kivelä, A. Koch, E. Kleinpeter, and K. D. Klika, submitted to Theochem.-J. Mol. Struct., 2009.
15. P. Kristian, Chem. Pap. - Chem. Zvesti, 1961, 15, 641.
16. B. Adcock, 'Aminoacridines' In 'Acridines', ed. by R. M. Acheson, (from 'The Chemistry of Heterocyclic Compounds' ed. by A. Weissberger and E. C. Taylor), J. Wiley: New York, 1973, p. 109.
17. I. Potočňák, J. Imrich, J. Tomaščiková, J. Kožíšek, I. Danihel, and P. Kristian, Acta Crystallogr., Sect. C, to be published.
18. P. Virta, A. Koch, M. U. Roslund, P. Mattjus, E. Kleinpeter, L. Kronberg, R. Sjöholm, and K. D. Klika, Org. Biomol. Chem., 2005, 3, 2924. CrossRef
19. J. Mäki, P. Tähtinen, L. Kronberg, and K. D. Klika, J. Phys. Org. Chem., 2005, 18, 240. CrossRef
20. A. D. Becke, J. Chem. Phys., 1993, 98, 5648. CrossRef
21. M. J. Frisch, G. W. Trucks, H. B. Schlegel, G. E. Scuseria, M. A. Robb, J. R. Cheeseman, J. A. Montgomery, Jr., T. Vreven, K. N. Kudin, J. C. Burant, J. M. Millam, S. S. Iyengar, J. Tomasi, V. Barone, B. Mennucci, M. Cossi, G. Scalmani, N. Rega, G. A. Petersson, H. Nakatsuji, M. Hada, M. Ehara, K. Toyota, R. Fukuda, J. Hasegawa, M. Ishida, T. Nakajima, Y. Honda, O. Kitao, H. Nakai, M. Klene, X. Li, J. E. Knox, H. P. Hratchian, J. B. Cross, V. Bakken, C. Adamo, J. Jaramillo, R. Gomperts, R. E. Stratmann, O. Yazyev, A. J. Austin, R. Cammi, C. Pomelli, J. W. Ochterski, P. Y. Ayala, K. Morokuma, G. A. Voth, P. Salvador, J. J. Dannenberg, V. G. Zakrzewski, S. Dapprich, A. D. Daniels, M. C. Strain, O. Farkas, D. K. Malick, A. D. Rabuck, K. Raghavachari, J. B. Foresman, J. V. Ortiz, Q. Cui, A. G. Baboul, S. Clifford, J. Cioslowski, B. B. Stefanov, G. Liu, A. Liashenko, P. Piskorz, I. Komaromi, R. L. Martin, D. J. Fox, T. Keith, M. A. Al-Laham, C. Y. Peng, A. Nanayakkara, M. Challacombe, P. M. W. Gill, B. Johnson, W. Chen, M. W. Wong, C. Gonzalez, J. A. Pople, Gaussian03, Revision D.02, Gaussian, Inc., Wallingford CT, 2003.