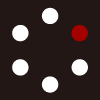
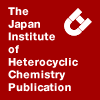
HETEROCYCLES
An International Journal for Reviews and Communications in Heterocyclic ChemistryWeb Edition ISSN: 1881-0942
Published online by The Japan Institute of Heterocyclic Chemistry
e-Journal
Full Text HTML
Received, 4th August, 2009, Accepted, 18th September, 2009, Published online, 24th September, 2009.
DOI: 10.3987/COM-09-S(S)106
■ Gaudichaudysolin A, a New Limonoid from the Bark of Dysoxylum gaudichaudianum
Yuta Nagakura, Reiko Yamanaka, Yusuke Hirasawa, Takahiro Hosoya, Abdul Rahman, Idha Kusumawati, Noor Cholies Zaini, and Hiroshi Morita*
Faculty of Pharmaceutical Sciences, Hoshi University, 2-4-41 Ebara, Shinagawa-ku, Tokyo 142-8501, Japan
Abstract
A new limonoid, gaudichaudysolin A (1) was isolated from the bark of Dysoxylum gaudichaudianum (Meliaceae) and the structure was elucidated by spectroscopic analysis.Limonoids, highly oxidative unique secondary metabolites obtained from Meliaceae are produced by a unique biosynthetic route through tetranortriterpenoid nucleus,1,2 and are known to show various biological activities such as insecticidal, insect antifeedant, antibacterial, antifungal, antimalarial, anticancer, and antiviral activities.3 Recently, we have isolated new limonoids, ceramicines A – D4 with an unique tetranortriterpenoid skeleton from Chisocheton ceramicus and walsogyne A5 with a ring C–seco limonoid from Walsura chrysogyne. They showed an antiplasmodial and cytotoxic activities.4,5
In continuation of our research on limonoids containing in the plants belonging to Meliaceae family, we have isolated a new limonoid, gaudichaudysolin A (1) from the bark of Dysoxylum gaudichaudianum. Herein we report the structure elucidation of gaudichaudysolin A (1) by spectroscopic methods.
The bark of D. gaudichaudianum was extracted with MeOH, and the MeOH extract was in turn partitioned between EtOAc and H2O. EtOAc-soluble materials were subjected to a silica gel column (hexane/EtOAc, 1:0→1:1; CHCl3/MeOH, 1:0→0:1) and the fractions eluted by hexane/EtOAc (1:1) were subjected to a silica gel column (Toluene/EtOAc, 1:0→5:5; CHCl3/MeOH, 1:0→5:5; CHCl3/MeOH/H2O, 5:5:1) followed by C18 HPLC (40% CH3CN/0.1%TFA) to afford gaudichaudysolin A (1, 0.00002 %).
Gaudichaudysolin A {1, [α]D23 -126 (c 0.2, MeOH)} was obtained as a colorless solid and was revealed to have the molecular formula C36H48O17, by HRESITOFMS [m/z 775.2780 (M+Na)+, Δ -0.9 mmu]. IR absorptions implied the presence of hydroxyl (3425 cm-1) and carbonyl (1755 and 1680 cm-1) groups. UV spectrum (230 nm) indicated the presence of an unsaturated carbonyl group. 1H and 13C NMR data (Table 1) revealed thirty six carbon resonances due to seven carbonyls, two sp2 quaternary carbons, three sp3 quaternary carbons, one sp2 methine, ten sp3 methines, one sp2 methylene, five sp3 methylenes, and seven methyl groups. Among them, eight sp3 carbons (δC 67.3, 70.0, 72.0, 75.2, 76.3, 81.4, 93.1, and 98.8) and seven sp2 carbons (δC 163.5, 172.1, 172.9, 173.2, 173.3, 177.8, and 208.0) were ascribed to those bearing an oxygen atom.
Six partial structures a (C-1 to C-2), b (C-5 to C-6), c (C-9 and C-11 to C-12), d (C-16 to C-17), e (C-22 to C-23), and f (C-2´ to C-6´) were deduced from 1H-1H COSY analysis of 1 in CD3OD (Figure 1). Unit A composed of the partial structures a and b was assigned as shown in Figure 1 with a γ-lactone ring by using HMBC and NOESY correlations as follows. HMBC correlations of H3-28 (δH 1.58) to C-4 (δC 93.1), C-5 (δC 43.8), and C-29 (δC 67.3), H2-6 (δH 2.80) to C-4 and C-7 (δC 177.8), and H2-29 (δH 3.72 and 3.78) to C-4 revealed the presence of a γ-lactone ring6 with a hydroxymethyl and a methyl groups at C-4. Acetoxy group at C-1 and a methyl carboxylate at C-2 were assigned by the HMBC correlations as shown in Figure 1. Connection between the partial structures a and b through C-10 (δC 49.6), was deduced by an HMBC correlation of H2-6 to C-10 and the NOESY correlations as shown in Figure 2. Unit B composed of the partial structures c and d was assigned as an octahydroinden-1-one ring system with a methyl, an exo-methylene, a hydroxyl, and two ester functions as follows. These functions can be connected by the HMBC correlations of H3-18 (δH 1.02) to C-12 (δC 75.2), C-13 (δC 51.5), C-14 (δC 81.4), and C-17 (δC 36.8), H-9 (δH 3.29) to C-8 (δC 142.0) and C-14, and H2-30 (δH 5.75 and 5.84) to C-9 and C-14. This ring system and functions were also supported by the comparison of the 1H and 13C NMR chemical shifts [C-14 (δC 81.4), C-15 (δC 208.0), and C-16 (δC 40.8)] with those [C-14 (δC 79.5), C-15 (δC 209.3), and C-16 (δC 42.0)] of rohituka 147 isolated from the seeds of Aphanamixis polystacha. The presence of a formate at C-11 was indicated by an HMBC correlation of an aldehyde proton to C-11 (δC 72.0). Connection between the units A and B was indicated by HMBC correlations of H-9 to C-5 and C-10. Unit C composed of the partial structure e, which was attached at C-17 in the unit B, was assigned as an α-substituted γ-lactone ring system with a hydroxyl at γ position by HMBC correlations of H-17 (δH 3.86) and H-22 (δH 7.19) to C-20 (δC 136.5), H-23 (δH 6.10) to C-21 (δC 172.9), and IR absorption at 1755 cm-1. Unit D composed of the partial structure f was assigned as a 2-hydroxy-3-methylpentanoic acid by the 1H-1H COSY correlation in Figure 1 and the comparison of NMR data in turrapubesin D,8 which might be attached at the hydroxy group at C-12 (δC 75.2). Thus, the gross structure of 1 was assigned as A,B-seco-tetranorlimonoid skeletal system with a γ-butanolide at C-17.
The relative stereochemistry with selected NOESY correlations in unit B and rotation model of C-5/C-10 bond in unit A for 1 were elucidated by NOESY correlations as shown in Figure 2. Configurations of C-9, C-11, C-12, C-13, and C-17 in the unit B were elucidated by NOESY correlations of H3-18/H-11 and H-16a, H-12/H-17, and H-9/H-11. As you can see the rotation model of C-5/C-10 bond, NOESY correlations of H3-19/H-2 and H2-6, H3-28/H-1, and H-5/H2-29 and H-30 indicated connectivity of C-5/C-10 bond and the relative stereochemistry in the unit A as shown in Figure 2. Thus, the relative configuration of 1 was assigned to be shown in computer-generated 3D drawing in Figure 3 except for C-2’ and C-3’ in the 2-hydroxy-3-methylpentanoic acid.
From the bark of Dysoxylum gaudichaudianum, a series of dysoxylins A – D with a tetranortriterpenoid nucleus have already been isolated.9 Biogenetically, gaudichaudysolin A (1) may be derived by a unique oxidative route through this tetranortriterpenoid nucleus. Gaudichaudysolin A (1) was evaluated in vitro for cytotoxicity against five human cancer cell lines, HL60 (human blood premyelocytic leukemia), RPMI8226 (multiple myeloma), NCI-H226 (non-small cell lung carcinoma), HCT116 (human colon cancer), and MCF7 (human breast adenocarcinoma) cells, using MTT assay, but showed no inhibitory activity against five tested cell lines (IC50 >50 µM).
EXPERIMENTAL
General Experimental Procedures. 1H and 2D NMR spectra were recorded on a Bruker AV600 spectrometer and chemical shifts were reported using residual CD3OD (δH 3.31 and δC 49.0) as internal standards. HSQC experiments were optimized for 1JCH=145 Hz and HMBC experiments for nJCH=8Hz. Mass spectra were recorded on a Micromass LCT spectrometer.
Plant Material. The bark of D. gaudichaudianum was collected at Alas Purwo, Indonesia in 2007. A voucher specimen is deposited at the Purwodadi Botanical Garden, Indonesia.
Extraction and Isolation. The bark of D. gaudichaudianum (1370 g) was extracted with MeOH, and the MeOH extract was partitioned between EtOAc and H2O. Water-soluble materials were extracted with BuOH. EtOAc-soluble materials were subjected to a silica gel column (hexane/EtOAc, 1:0→1:1; CHCl3/MeOH, 1:0→0:1) and the fractions eluted by hexane/EtOAc (1:1) were subjected to a silica gel column (toluene/EtOAc, 1:0→5:5; CHCl3/MeOH, 1:0→5:5, CHCl3/MeOH/H2O 5:5:1) followed by C18 HPLC (40% CH3CN/0.1%TFA) to afford gaudichaudysolin A (1, 1.3 mg, 0.00002 %).
Gaudichaudysolin A (1): a colorless amorphous solid; [α]D23 -126 (c 0.2, MeOH); IR (KBr) νmax 3425, 1755, 1680, 1630, 1585, 1440, 1390, 1200, 1135, 1075, 840, and 801 cm-1; UV (MeOH) λmax 230 (ε 9000); 1H and 13C NMR data (Table 1); ESIMS m/z 775 (M+Na)+; HRESITOFMS m/z 775.2780 [(M+Na)+, calcd for C36H48O17, 775.2789].
Cytotoxic Activity. Each cell line [HL60 (human blood premyelocytic leukemia), RPMI8226 (multiple myeloma), NCI-H226 (non-small cell lung carcinoma), HCT116 (human colon cancer), and MCF7 (human breast adenocarcinoma) cells] was seeded onto 96-well microtiter plates at 1 × 104 cells per well for HL60 and RPMI8226 and 5 × 103 cells per well for NCI-H226, HCT116, and MCF7, respectively. Cells were preincubated for 24 h at 37°C in humidified atmosphere of 5% CO2. Different concentrations of each compound (10 µL) were added to the cultures, and then the cells were incubated at 37°C for 48 h. On the third day, 15 µL MTT solution (5 mg/mL) was added into each well of the cultured medium. After further 2 h of incubation, 100 µL of 10% SDS-0.01N HCl solution was added to each well and the formazan crystals in each well were dissolved by stirring with a pipette. The optical density measurements were made using a micropipette reader (Benchmark Plus microplate spectrometer, BIO-RAD) equipped with a two wavelengths system (550 and 700 nm). In each experiment, three replicate of wells were prepared for each sample. The ratio of the living cells was determined based on the difference of the absorbance between those of samples and controls. These differences are expressed in percentage and cytotoxic activity was indicated as an IC50 value.
ACKNOWLEDGMENTS
This work was supported by a Grant-in-Aid for Scientific Research from the Ministry of Education, Culture, Sports, Science, and Technology of Japan, and grants from the Research Foundation for pharmaceutical Sciences and The Open Research Center Project.
References
1. (a) D. A. H. Taylor, In Progress in the Chemistry of Organic Natural Products; ed. by W. Herz, H. Grisebach, and G. W. Kirby; Springer, New York, 1984; Vol. 45; (b) D. A. Mulholland, B. Parel, and P. H. Coombes, Curr. Org. Chem., 2000, 4, 1011. CrossRef
2. (a) S. Yin, X. N. Wang, C. Q. Fan, S. G. Liao, and J. M. Yue, Org. Lett., 2007, 9, 2353; CrossRef (b) C. R. Zhang, S. P. Yang, S. G. Liao, C. Q. Fan, Y. Wu, and J. M. Yue, Org. Lett., 2007, 9, 3383; CrossRef (c) Y. T. Di, H. P. He, H. Y. Liu, P. Yi, Z. Zhang, Y. L. Ren, J. S. Wang, Q. Y. Sun, F. M. Yang, X. Fang, S. L. Li, H. J. Zhu, and X. J. Hao, J. Nat. Prod., 2007, 70, 1352. CrossRef
3. (a) H. Zhang, X. Wang, F. Chen, X. M. Androulakis, and M. J. Wargovich, Phytotherapy Res., 2007, 21, 731; CrossRef (b) A. Roy and S. Saraf, Biol. Pharm. Bull., 2006, 29, 191; CrossRef (c) D. H. Bray, D. C. Warhurst, J. D. Connolly, M. J. O'Neill, and J. D. Phillipson, Phytotherapy Res., 1990, 4, 29. CrossRef
4. K. Mohamad, Y. Hirasawa, M. Litaudon, K. Awang, A. H. A. Hadi, K. Takeya, W. Ekasari, A. Widyawaruyanti, N. C. Zaini, and H. Morita, Bioorg. Med. Chem., 2009, 17, 727. CrossRef
5. K. Mohamad, Y. Hirasawa, C. S. Lim, K. Awang, A. H. A. Hadi, K. Takeya, and H. Morita, Tetrahedron Lett., 2008, 49, 4276. CrossRef
6. L. K. MacLachlan and D. A. H. Taylor, Phytochemistry, 1982, 21, 2426. CrossRef
7. D. A. Mulholland and N. Naidoo, Phytochemistry, 1999, 51, 927. CrossRef
8. X. N. Wang, S. Yin, C. Q. Fan, L. P. Lin, J. Ding, and J. M. Yue, Tetrahedron, 2007, 63, 8234. CrossRef
9. J. L. Chen, M. R. Kernan, S. D. Jolad, C. A. Stoddart, M. Bogan, and R. Cooper, J. Nat. Prod., 2007, 70, 312. CrossRef