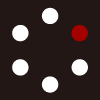
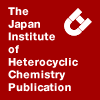
HETEROCYCLES
An International Journal for Reviews and Communications in Heterocyclic ChemistryWeb Edition ISSN: 1881-0942
Published online by The Japan Institute of Heterocyclic Chemistry
e-Journal
Full Text HTML
Received, 12th August, 2009, Accepted, 13th October, 2009, Published online, 13th October, 2009.
DOI: 10.3987/COM-09-S(S)110
■ RhCl3-Catalyzed Disulfide Exchange Reaction of Insulin and Dithiodiglycolic Acid
Mieko Arisawa, Manabu Kuwajima, Atsushi Suwa, and Masahiko Yamaguchi*
Graduate School of Pharmaceutical Sciences, Tohoku University, Aramaki, Aoba-ku, sendai 980-8578, Japan
Abstract
RhCl3 catalyzed the alkylthio exchange reaction of insulin and dithiodiglycolic acid in water under homogeneous conditions. Insulin was rapidly consumed, and mono- and bis-exchanged insulin were formed along with the disulfide exchanged A and B chain. The disulfide bond at the A7/B7 was initially exchanged, which was followed by the A6/A11 and A20/B19 disulfides.Chemical modification of proteins has attracted much interest in regard to the development of artificial proteins possessing novel function, and use of transition metal catalysis has become a topic in recent years.1 It is because the catalysis can exhibit different reactivity from thermal, photochemical, acid, or base methods. Reactive sites in the native protein such as tyrosine phenol, tryptophan indole, or cystein sulfide were reacted with organometallic reagents.1 Cysteine disulfide is a common and essential structure for the construction of protein three-dimentional structure, and the disulfide moiety can be another target of the protein modification. Transition metal catalyzed method to modify disulfide moiety, however, has not been examined. Previously, we reported RhCl3-catalyzed disulfide exchange reaction of unprotected glutathione in water, which proceeded smoothly at ambient temperature tolerating sensitive functional groups.2 The result suggested a potential of this method for the protein modification.
Insulin 1 is a protein that exhibits important biological activities, and contains A and B chains connected by two disulfide linkages at the A7/B7 and A20/B19 cysteine with another disulfide linkage at the A6/A11. Thus, it was considered interesting to apply the rhodium-catalyzed disulfide exchange method to the modification of 1. The disulfide exchange reaction of insulin has a few precedents: Sanger reacted 1 with dinitrophenylcysteine in 12 M hydrochloric acid.3 The reaction site, however, was not determined. Busse reacted 1 and dithiodiglycolic acid 2 or glutachione disulfide in the presence of a thiol under acidic conditions, and obtained small amounts of the exchanged products,4 in which the preferencial clevage of the A7/B7 disulfide over A6/A11 was noted. Described here is the rhodium-catalyzed method for the modification of 1. The reaction of insulin 1 and dithiodiglycolic acid 2 gave a mixture of five disulfide exchange products, 1-mono2, 1-bis2, A-mono2, A-bis2, and B-mono2. The compounds 1-mono2 and 1-bis2 were the mono- and bis-adduct of 1, respectively; A-mono2 and A-bis2, mono- and bis-adduct of the A-chain, respectively; B-mono2, mono-adduct of the B-chain. The reaction proceeded under mild conditions with rapid consumption of 1, and the initial cleavage occurred at the A7/B7 disulfide, which was followed by the reaction of the other disulfides A20/B19 and A6/A11.
RESULTS AND DISCUSSION
In the presence of RhCl3•3H2O (300 mol%), insulin 1 was reacted with dithiodiglycolic acid 2 (500 eq.) in water at room temperature for 2 h. Use of large excess 2 increased the solubility of 1. Then, 0.5 M ammonium acetate was added, and insoluble insulin derivatives were obtained by centrifuge, which were subjected to LC MS analysis detected by TI (total ion between m/z 300 to 4000) and UV (254 nm) (Figure 1). The structures were determined by MS (Figure 2), and the yields by UV analysis with calibration: 1-mono2 (15%), 1-bis2 (25%), B-mono2 (51%), A-mono2 (32%), and A-bis2 (25%) (Table 1, entry 2). The yield of B-mono2 was approximately the same with the combined yield of A-mono2 and A-bis2. Insulin 1 was consumed under the conditions, which showed the high efficiency of the present method. When the reaction was stopped in 1 h, the total yield of the disulfide exchanged products decreased to about 30% giving 1-mono2 (16%) along with small amounts of 1-bis2, B-mono2, and A-mono2 (entry 1). The reaction for 6 h decreased 1-mono2 (3%) (entry3). The results indicated the initial formation of 1-mono2, which was consumed with the reaction course. Longer reaction time resulted in the precipitation of the products, probably because of the lower solubility of the acidic insulin derivatives in acidic media. The rhodium complex is essential for present reaction, and no reaction occurred in its absence.
The disulfide exchanged products were isolated by the following procedures. The crude insulin derivatives obtained by precipitation from the reaction mixture was dissolved in 0.3 M ammonium bicarbonate. Then, 1 M acetic acid/ammonium acetate buffer (pH 3.5) was added to precipitate the products again in order to reduce the amount of rhodium metal in the product. Anion exchange chromatography using aqueous ammonium bicarbonate provided fractions containing B-mono2, a mixture of 1-mono2 and A-mono2, and a mixture of 1-bis2 and A-bis2. Then, the products were subjected to desalting by reverse phase chromatography using methanol and triethylamine/CO2 buffer as eluent, during which process 1-mono2, A-mono2, 1-bis2, and A-bis2 were isolated. At this stage, 98% of the rhodium metal was removed as indicated by ICP OES analysis. The disulfide exchanged insulin derivatives were soluble in basic solutions but not acidic, and triethylamine/CO2 buffer dissolved the compounds at neutral conditions. This buffer system containing a volatile salt could be used for LC MS analysis. The pure samples of B-mono2 and A-bis2 as well as insulin 1 were used to obtain the calibration curves with fluorescein sodium as internal standard. The calibration curve for insulin 1 was also used for the analysis of 1-mono2 and 1-bis2; the curve for A-bis2 was used for the analysis of A-mono2.
The site of the exchanged disulfide were determined by chymotrypsin digestion experiments using isolated 1-mono2, A-mono2, and 1-bis2. Chymotrypsin cleaves peptides and proteins at phenylalanine and tyrosine residues. The compound 1-mono2 was treated chymotrypsin for 1 h, and the reaction mixture was subjected to ESI MS analysis (Figure 3). The peaks m/z 1920 and 1562 were assigned to the fragments A1-A14 and B1-B16 both containing a SCH2COOH moiety (Table 2). Thus, 1-mono2 was the product possessing A6/A11 and A20/B19 disulfides. A small MS peak at m/z 1741 ascribed to the disulfide cleavage product at the A6/A11 was observed in less than 5% of the sum of the m/z 1920 and 1562 peaks. The MS peaks, m/z 1650, 1117, and 973, derived from the product reacted at the A20/B19 disulfide was essentially not detected. Thus, the selective cleavage of 1 occurred at the A7/B7 disulfide under the rhodium conditions. By the digestion experiment, the compound 1-bis2 was shown to be the product of the A6/A11 disulfide exchange in 1-mono2, and the other isomer exchanged at the A6/A11 and A20/B19 disulfide was not detected. Analogously, the product A-mono2 was the A chain derivative exchanged at the A7 and A20 disulfides.
The mechanism of rhodium-catalyzed insulin disulfide exchange was summarized (Scheme 1). The initial reaction of 1 in the present catalysis took place selectively at the A7/B7 disulfide. That 1-bis2 did not contain A6/A11 exchange product was consistent with the high selectivity in the first reaction. The results are in accordance with the previous report by Busse:4 They reported the A7/B7 disulfide exchange and A6/A11 exchange in a 78:22 ratio. However, the selectivity in the first cleavage reaction appears to be higher in the present method, and it should be noted that the efficiency of the exchange reaction was much higher. Then, the next disulfide exchange reaction of 1-mono2 took place both at the A6/A11 and A20/B19 disulfides at comparable rates, which was indicated by the comparable yields of 1-bis2 and A-mono2 after 2 h (Table 1).
To summarize, RhCl3 catalyzed rapid disulfide exchange reaction of insulin with dithiodiglycolic acid giving a series of modified insulins. Transition metal catalyzed method may be used for the disulfide modification of various proteins.
EXPERIMENTAL
ESI LC MS analyses were conducted on a JEOL JMS T100LC equipped with Agilent1100. ICP OES analyses were conducted on an Optima 3300. Wakogel 50C18 (38-63 µm) was used for reverse-phase chromatography, and QA cellulose for ion exchange chromatography. Solution pH was measured by HORIBA pH METER D-51.
General Procedures for Disulfide Exchange Reaction In a test tube was placed insulin 1 (1.3 µmol, 7.5 mg), RhCl3•3H2O (3.9 µmol, 1.0 mg), and dithiodiglycolic acid 2 (0.650 mmol, 118.4 mg) in degassed water (0.1 mL) under an argon atmosphere, and the solution was stirred at room temperature for 2 h. The mixture was transferred into two microtubes (1.5 mL). The test tube was washed with water (0.5 mL) and 0.3 M NH4(HCO3) (0.2 mL), and the solutions were transferred to the microtubes. Then, 0.5 M AcONH4 aqueous solution was added, and the total volume in each microtube was adjusted to 1 mL. The mixtures were shaken, cooled in ice bath for 30 min, and centrifuged (6400 rpm) for 20 min. Top layers were removed, and water was added to each microtube to adjust the volume to 1 mL. The mixtures were shaken, and centrifuged for 20 min. After removing the top layers, the mixture was concentrated in vacuo giving crude insulin derivatives (9.3 mg). Yields were determined by LC MS analysis with UV calibration: 1-mono2 (15%), 1-bis2 (25%), B-mono2 (51%), A-mono2 (32%), and A-bis2 (25%).
Isolation Procedures The crude products obtained as above were dissolved in 0.3 M NH4(HCO3) (0.5 mL) in six microtubes. Then, 1 M AcOH/AcONH4 buffer (pH 3.5) was added in each microtube, and each volume was adjusted to 1 mL. The mixtures were shaken, cooled in ice bath for 1 h, and centrifuged. The top layers were removed, and water was added to adjust the volume to 1 mL. The mixtures were stirred vigorously, and centrifuged for 10 min. The top layers were removed, and the mixtures were concentrated in vacuo. The combined products (8.1 mg) were dissolved in 0.2 M (NH4)HCO3 (3.0 mL), and purified by ion exchange chromatography (QA cellulose). The solvent was changed from 0.1 M to 0.3 M (NH4)HCO3 aqueous solution, and fractions containing B-mono2, 1-mono2 and A-mono2, and 1-bis2 and A-bis2 were obtained in this order. Then, the fractions containing B-mono2 were combined, and subjected to reversed phase chromatography (Wakogel 50C18) eluting with 0.5 M triethylamine-CO2 (pH 7.0) buffer to remove inorganic salts. Then, elution with cooled methanol gave B-mono2 (1.3 mg). The fractions containing 1-mono2 and A-mono2 were combined, and were subjected to reversed phase chromatography. Inorganic salts were removed by eluting with 0.5 M triethylamine-CO2 (pH 7.0) buffer. Elution with cooled 1:1 methanol/triethylamine-CO2 buffer gave A-mono2 (0.5 mg), and with cooled methanol 1-mono2 (1.3 mg). The fractions containing 1-bis2 and A-bis2 were combined, and were subjected to the reversed phase chromatography. Elution with 0.5 M triethylamine-CO2 (pH 7.0) buffer removed inorganic salts. Elution with cooled 1:1 methanol/triethylamine-CO2 buffer gave A-bis2 (1.0 mg), and cooled methanol was used to obtain 1-bis2 (3.1 mg). ICP OES analysis on each isolated sample indicated that the entire products contained 0.1 µg rhodium metal, and accordingly 98% of rhodium metal used was removed.
ICP OES Analysis Rhodium metal concentration was determined by ICP OES spectrometry measured in mg/L at 343.489 nm. After calibration with a sample of known absorption, the concentration of the solution was determined independently. For this analysis, the samples were dried, and dissolved in 25 mL of 0.3 M (NH4)HCO3.
LC MS Analysis Insulin derivatives 1-mono2, A-mono2, 1-bis2, and A-bis2 were analyzed by LC MS using CAPCELL PAK C18 TYPE MGII (5 µm, 2.0x150 mm) (Shiseido) at ambient temperature with flow rate 0.2 mL/min. The compounds were detected by UV (254 nm) and TI (m/z 300 to 4000). Mixtures of methanol and 0.5 M triethylamine-CO2 buffer (pH 7.0) were used with gradient from methanol/buffer = 40/60 to 50/50 for 100 min, then from 50/50 to 60/40 for 20 min, and from 60/40 to 70/30 for 60 min. In case of B-mono2, mixtures of methanol and 0.5 M triethylamine-CO2 buffer (pH 7.0) were used with gradient from methanol/buffer = 40/60 for 20 min, and then gradient from 40/60 to 100/0 for 30 min at ambient temperature.
1-mono2. MS (ESI) Calcd for C258H383N65O79S8: m/z 2957.295, 2957.796, 2958.297, 2958.798, 2959.298, 2959.799, 2960.300, 2960.800, 2961.300 (M+2H/2). Found: m/z 2957.062, 2957.574, 2958.022, 2958.599, 2959.112, 2959.560, 2960.007 (M+2H/2).
1-bis2. MS (ESI) Calcd for C262H389N65O83S10: m/z 3047.779, 3048.280, 3048.781, 3049.282, 3048.783, 3049.282, 3049.783, 3050.284, 3050.784, 3051.285, 3051.785, 3052.286, 3052.786, 3053.286, 3053.787, 3054.287. (M+2H/2). Found: m/z 3047.934, 3048.389, 3048.909, 3049.365, 3049.885, 3050.275, 3050.470, 3050.991, 3051.251, 3051.967 (M+2H/2).
A-mono2. MS (ESI) Calcd for C101H153N25O38S6: m/z 2516.921, 2517.924, 2518.924, 2519.925, 2520.925, 2521.926 (M+H). Found: m/z 2516.648, 2517.650, 2518.656, 2519.572, 2520.636 (M+H).
A-bis2. MS (ESI) Calcd for C105H159N25O42S8: m/z 2698.892, 2699.895, 2700.894, 2701.895, 2702.895, 2703.895 (M+H). Found: m/z 2698.596, 2699.606, 2700.555, 2701.565, 2702.422.
B-mono2. MS (ESI) Calcd for C161H237N40O45S4: m/z 1790.326, 1790.828, 1791.328, 1791.830, 1792.330, 1792.831, 1793.331 (M+2H/2). Found: m/z 1789.937, 1790.410, 1790.907, 1791.404, 1791.927, 1792.374, 1792.871.
Calibration for HPLC Analysis of 1, A-bis2, and B-mono2 Insulin 1 were weighed, 0.746 mg, 2.88 mg, 3.74 mg, and 7.46 mg, and were dissolved in 20 mM triethylamine (1.0 mL) and 0.2 M (NH4)HCO3 (2.0 mL), to which 1.0 mM fluorescein sodium (0.10 mL) was added as internal standard. HPLC-UV analysis at 254 nm provided the calibration curve.
Samples of A-bis2 were weighed, 0.35 mg, 0.87 mg, and 1.57 mg, and were dissolved in 0.2 M (NH4)HCO3 (3.0 mL), to which 1.0 mM fluorescein sodium (0.10 mL) was added as internal standard. HPLC-UV analysis at 254 nm provided the calibration curve.
Samples of B-mono2 was weighed, 0.47 mg, 2.34 mg, and 4.68 mg, and were dissolved in 0.2 M (NH4)HCO3 (3.0 mL), to which 1.0 mM fluorescein sodium (0.10 mL) was added as internal standard. HPLC-UV analysis at 254 nm provided the calibration curves.
Digestion Experiment Compound 1-mono2 (1.5 mg) was dissolved in 0.2 M (NH4)HCO3 (5 mL), and chymotripsinogen (0.01 eq.) in water (0.1 mL) was added. The mixture was stirred at ambient temperature for 1 h, and was analyzed by ESI MS (Figure 3, Table 2). The some experiments were conducted for 1-bis2 (Figure 4, Table 3) and A-mono2 (Figure 5, Table 4).
ACKNOWLEDGEMENTS
This work was supported by JSPS (Nos. 16109001 and 21229001), GCOE program, and WPI Initiative. M. A. expresses her thanks to the Grant-in-Aid for Scientific Research on Priority Areas, “Advanced Molecular Transformation of Carbon Resources” from MEXT (Nos. 18037005 and 19020008) and also to the Asahi Glass Foundation.
References
1. K. C. Brown, S.-H. Yang, and T. Kodadek, Biochem., 1995, 34, 4733; CrossRef X. Luo, W. He, Y. Zhang, Z. Guo, and L. Zhu, Chem. Lett., 2000, 1030; CrossRef J. M. Antos and M. B. Francis, J. Am. Chem. Soc., 2004, 126, 10256; CrossRef J. M. McFarland and M. B. Francis, J. Am. Chem. Soc., 2005, 127, 13490; CrossRef T. Kodadek, I. D.-Richard, and J.-C. Bonnafous, Trends Pharmacol. Sci., 2005, 26, 210; CrossRef S. D. Tilley and M. B. Francis, J. Am. Chem. Soc., 2006, 128, 1080; CrossRef Y. A. Lin, J. M. Chalker, N. Floyd, G. J. L. Bernardes, and B. G. Davis, J. Am. Chem. Soc., 2008, 130, 9642; CrossRef J. M. Antos, J. M. McFarland, A. T. lavarone, and M. B. Francis, J. Am. Chem. Soc., 2009, 131, 6301. CrossRef
2. M. Arisawa, A. Suwa, and M. Yamaguchi, J. Organomet. Chem., 2006, 691, 1159. CrossRef
3. A. P. Ryle and F. Sanger, Biochem. J., 1955, 60, 535.
4. W.-D. Busse and H.-G. Gattner, Hoppe-Seyler's Z. Physiol. Chem., 1973, 354, 1057.