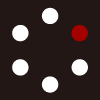
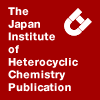
HETEROCYCLES
An International Journal for Reviews and Communications in Heterocyclic ChemistryWeb Edition ISSN: 1881-0942
Published online by The Japan Institute of Heterocyclic Chemistry
e-Journal
Full Text HTML
Received, 7th September, 2009, Accepted, 23rd June, 2010, Published online, 24th June, 2010.
DOI: 10.3987/COM-09-11828
■ Formal Syntheses of Dihydrocorynantheine and Isorhynchophylline via Proline Catalyzed Mannich-Michael Reaction
Kazuhiro Nagata, Hitomi Ishikawa, Ayako Tanaka, Michiko Miyazaki, Takuya Kanemitsu, and Takashi Itoh*
School of Pharmaceutical Sciences, Showa University, 1-5-8 Hatanodai, Shinagawa-ku, Tokyo 142-8555, Japan
Abstract
Proline catalyzed Mannich-Michael reaction of 3-ethyl-3-buten-2-one with 9-tosyl-3,4-dihydro-β-carboline proceeded in a highly stereoselective manner. The reaction was applied to formal syntheses of dihydrocorynantheine and isorhynchophylline.INTRODUCTION
We have been investigating asymmetric addition reactions to cyclic imines and their application to the syntheses of indole alkaloids.1 In the study, we have found a highly enantioselective addition of ketones to 9-tosyl-3,4-dihydro-β-carboline using proline catalyst.2 1-Substituted-β-carbolines thus obtained would be viable precursors for the synthesis of corynanthe-type indole alkaloids and biogenetically related oxindole alkaloids. Dihydrocorynantheine (1) and isorhynchophylline (2) were selected as target molecules for evaluating the availability of the above mentioned reaction. Dihydrocorynantheine was first isolated from the bark of Pseudocinchona africana A. Chev.3 as early as 1938, but few examples of the asymmetric synthesis were reported.4 In the case of isorhynchophylline, which is also an old alkaloid from Uncaria rhynchophylla Miq.,5 no enantioselective synthesis was reported. Thus we have
investigated an application of our method to the syntheses of these alkaloids. In this paper, these results are described.
RESULTS AND DISCUSSION
The synthesis of dihydrocorynantheine (1) was commenced with the compound 3 which was prepared from the enantioselective Mannich-Michael reaction catalyzed by (R)-proline2 (Scheme 1). Since removal of N-9 tosyl group at a later stage of the synthesis was revealed to be difficult, elimination of tosyl group was to be carried out firstly. After protection of carbonyl group by 1,3- propanediol to give compound 4, removal of tosyl group was performed using Red-Al, and then the acetal was cleaved to give 5 as a mixture of epimer at C(20). The structures of the both isomers were determined by comparison of 1H and 13C NMR data with reported ones.6 This epimerization was of no consequence because the chiral center returned to the original configuration in the next step. Thus, without a separation of the epimers, the resulting ketones 5 were subjected to a Horner-Wadsworth-Emmons reaction with trimethyl phosphonoacetate to afford compound 6 in 84% yield.7 A. I. Meyers et al. reported that treatment of either 5 or its epimer at C(20) with 10% KOH gave the 10:1 equilibrium mixture of 5 and its epimer at C(20).6 Thus, in the basic conditions of Horner-Wadsworth-Emmons reaction, the epimer was considered to isomerize to thermodynamically more stable compound 5. Stereochemistry of E/Z configuration was determined by NOE observations (Figure 2). Hydrogenation with Wilkinson’s catalyst under medium pressure gave 7 in 60 % yield. The synthesis of dihydrocorynantheine
from 7 was already reported using condensation reaction with methyl formate followed by methylation with diazomethane.4, 8 Therefore, a new synthetic route to dihydrocorynantheine was established.
We then investigated the synthesis of isorhynchophylline (Scheme 2). Rhynchophylline and isorhynchophylline, which have spiro[pyrrolidine-3,3’-oxindole] ring system, have been used as ingredients of folk medicines, and exhibit antipyretic and hypotensive activities.9 Moreover, these alkaloids possess a protective effect against glutamate-induced neuronal death.10 Although there are two examples for the total synthesis of rhynchophylline and isorhynchophylline,11, 12 enantioselective synthesis has not yet been reported. It was considered that the key step for the synthesis of rhynchophylline and/or isorhynchophylline was oxidative transformation of tetrahydro-β–carboline into oxindole to construct the spiro[pyrrolidine-3,3’-oxindole] ring system. Finch and Taylor reported the oxidative transformation of dihydrocorynantheine to rhynchophylline,13 but the yield was low. Recently, Martin et. al. reported the transformation of an ethyl ester of compound 7 into corresponding oxindole, but products obtained were a mixture of epimers with the selectivity of 42:33, and these compounds were led to rhynchophylline and isorhynchophylline, respectively, in a racemic synthesis.12 Since the stereoselectivity in the oxidative transformation was considered to depend on the conformation of CD ring,14 we examined conversion of tetrahydro-β-carboline skeleton to the spiro- [pyrrolidine-3,3’-oxindole] ring in early stages of the synthesis. When compound 5 was allowed to react with NBS,14, 15 oxindole 8 was obtained in 80 % yield as a single diastereomer. Although stereospecific construction of spiro[pyrrolidine-3,3’-oxindole] ring was achieved in the reaction, following Horner-Wadsworth-Emmons reaction was unsuccessful to give compound 9. Thus, oxidative
transformation of 6 into compound 9 with NBS was investigated. The result was that a single diastereomer was isolated in 57% yield. Configurations at C(7) position of compound 8 and 9 were determined by NOE spectroscopic experiments (Figure 2). Since compound 9 had been converted into isorhynchophylline in three steps,11 a formal synthesis of isorhynchophylline was accomplished.
In summary, we have established a new synthetic route to the synthesis of dihydrocorynantheine and achieved a first formal synthesis of isorhynchophylline as an optically active form. These results show the usefulness of proline catalyzed Mannich-Michael reaction of 9-tosyl-3,4-dihydro-β-carboline. The application of the method to the synthesis of other alkaloids is now under investigation.
EXPERIMENTAL
General. Unless otherwise specified, reagents were purchased from commercial suppliers and used without further purification. NBS was recrystallized from H2O prior to use. Dehydrated toluene, benzene, DMSO, THF, and MeOH were purchased from commercial suppliers. Moisture sensitive reactions were carried out under an atmosphere of Ar. 1H and 13C NMR spectra ware recorded on a 500 MHz (125 MHz for 13C) or a 400 MHz (100 MHz for 13C)spectrometer. All melting points are uncorrected.
Synthesis of compound 4
To a solution of 3 (40 mg, 94 μmol) in toluene was added 1,3-propanediol (69 μL, 0.94 mmol) and p-TsOH ·H2O (3.6 mg, 19 μmol). The solution was refluxed for 1 hour using Dean-Stark apparatus. After cooling to room temperature, saturated aqueous NaHCO3 sol. was added and extracted with AcOEt. The combined organic layers were dried over MgSO4 and evaporated off. The residue was purified by chromatography on silica gel (CH2Cl2:AcOEt=10:1→8:1) to give compound 4 (39 mg) in 86% yield: pale yellow amorphous; [α]17D +174.03 (c 0.98, CHCl3); 1H-NMR (500 MHz CDCl3) δ: 0.95 (3H, t, J=7.4 Hz), 1.12 (1H, septed, J=7.4 Hz), 1.41 (1H, d, J=13.2 Hz), 1.48 (1H, t, J=12.7 Hz), 1.64-1.70 (1H, m), 1.96-2.08 (2H, m), 2.27 (3H, s), 2.71 (2H, brs), 2.77 (1H, dd, J=10.9 Hz, 4.6 Hz), 2.97 (1H, t, J=12.6 Hz), 3.19 (1H, m), 3.49 (1H, dd, J=13.7 Hz, 2.3 Hz), 3.79 (1H, dd, J=11.5 Hz, 3.4 Hz), 3.90 (1H, dd, J=12.0 Hz, 5.7 Hz), 4.16 (1H, td, J=13.2 Hz, 2.9 Hz), 4.41 (1H, d, J=10.9 Hz), 4.55 (1H, td, J=12.6 Hz, 2.3 Hz), 7.08 (1H, d, J=8.0 Hz), 7.21 (2H, td, J=7.4 Hz, 1.1 Hz), 7.25-7.30 (3H, m), 7.51 (2H, d, J=8.0 Hz), 8.12 (1H, d, J=8.0 Hz); 13C-NMR (125 MHz CDCl3) δ: 12.6, 18.1, 21.5, 22.2, 25.7, 31.1, 43.0, 44.9, 55.0 55.7, 59.3, 59.5, 98.1, 115.8, 118.4, 120.1, 124.0, 124.5, 126.4, 127.6, 129.5, 130.8, 134.0, 137.4, 144.6; HR-FAB MS: Calcd for C27H33O4N2S [M+H]+: 481.2161. Found: 481.2137.
(3R,12bS)-Methyl (3-Ethyl-3,4,6,7,12,12b-hexahydro-1H-indolo[2,3-a]quinolizin-2-ylidene)acetate (6)
To a solution of 4 (1.26 g, 2.6 mmol) in toluene (15 mL) was added Red-Al (3.6 mol/L in toluene 4.4 mL, 15.8 mmol) at room temperature, then the solution was refluxed for 3 h. 10% aqueous Rochelle salt solution was added to the solution and extracted with AcOEt. The organic layer was dried over MgSO4, and evaporated off. The residue was purified by chromatography on silica gel (CH2Cl2:AcOEt=2:1→3:2) to give detosylated compound (753 mg). This compound was dissolved in dioxane (22 mL) and 1N aq. HCl (4.5 mL) was added to the solution, and then the solution was refluxed for 1 h. Aqueous K2CO3 solution was added to the solution at 0 ºC, and the aqueous layer was extracted with AcOEt. The combined organic layers were dried over MgSO4, and evaporated off. The residue was purified by chromatography on silica gel (CHCl3:AcOEt= 4:1) to give 5 (533 mg) as a mixture of epimer at C(20) (5:epimer=3:2). Part of this mixture (227 mg, 0.847 mmol) was dissolved in benzene (10 mL). This solution was added to a benzene solution of NaH (60% in oil 169 mg, 4.23 mmol) and trimethyl phosphonoacetate (635 μL, 4.23 mmol) and then refluxed for 2 h. After saturated aqueous NaHCO3 solution was added, the aqueous layer was extracted with AcOEt. The combined organic layers were dried over MgSO4 and evaporated off. The residue was purified by chromatography on silica gel (CHCl3:Et2O=5:1) to give compound 6 (231 mg) in 84 % yield; brown amorphous; [α]21D +60.0 (c 0.40, CHCl3); 1H-NMR (400 MHz CDCl3) δ: 0.96 (3H, t, J=7.4 Hz), 1.43 (1H, septed, J=6.8 Hz), 1.77 (1H, septed, J=6.8 Hz), 2.27-2.33 (2H, m), 2.65-2.70 (2H, m), 2.80 (1H, dt, J=6.6 Hz, 11.4 Hz), 2.94-3.06 (1H, m), 3.12-3.18 (2H, m), 3.65 (1H, d, J=8.1 Hz), 3.73 (3H, s), 3.98 (1H, d, J=13.2 Hz), 5.69 (1H, s), 7.06 (1H, t, J=7.5 Hz), 7.12 (1H, d, J=7.2 Hz), 7.28 (1H, d, J=8.0 Hz), 7.45 (1H, d, J=7.5 Hz), 8.56 (1H, s); 13C-NMR (100 MHz CDCl3) δ: 11.7, 20.5, 22.4, 31.7, 45.9, 51.1, 51.7, 58.6, 59.5, 107.6, 111.0, 112.6, 118.0, 119.2, 121.2, 127.2, 133.7, 136.0, 161.9, 167.8; HR-FAB MS: Calcd for C20H24O2N2 [M+H]+: 325.1926. Found: 325.1916.
(3R,12bS)-Methyl (3-Ethyl-1,2,3,4,6,7,12,12b-octahydroindolo[2,3-a]quinolizin-2-yl)acetate (7)
In the reactor, compound 6 (22 mg, 68 μmol) was dissolved in MeOH (5 mL) and chlorotris- (triphenylphosphine)rhodium (I) (13 mg, 14 μmol) was added. The solution was stirred for 5 h under 5 bar H2 atmosphere at room temperature. The catalyst was removed by filtration through a plug of alumina, and the filtrate was concentrated under reduced pressure. The residue was purified by chromatography on silica gel (CHCl3:Et2O=5:1→ 4:1) to give 7 in 60 % yield; pale yellow amorphous; 1H-NMR (400 MHz CDCl3) δ: 0.93 (3H, t, J =7.4), 1.17 (1H, septed, J =7.6), 1.37 (1H, q, J =12.0), 1.47-1.51 (1H, m), 1.59-1.64 (1H, m), 1.79-1.84 (1H, m), 2.09-2.15 (2H, m), 2.23 (1H, d, J =12.0), 2.60 (1H, dt, J =4.6, 11.5), 2.71 (1H, dt, J =3.4, 14.5), 2.97-3.03 (1H, m), 3.07-3.13 (2H, m), 3.24 (1H, d, J =11.4), 3.73 (3H, s), 7.08 (1H, t, J =8.0), 7.13 (1H, t, J =6.9), 7.29 (1H, d, J =8.0), 7.46 (1H, d, J =7.5), 7.85 (1H, s); 13C-NMR (100 MHz CDCl3) δ: 11.0, 21.7, 23.5, 36.0, 37.3, 38.0, 41.6, 51.6, 53.1, 59.5, 60.1, 108.1, 110.7, 118.1, 119.3, 121.3, 127.3, 134.5, 136.0, 173.7; HR-FAB MS: Calcd for C20H27O2N2 [M+H]+: 327.2073. Found: 327.2101. This compound showed a tendency to turn dark after purification, and varying of the value of specific rotation was observed during measurement. Thus the absolute configuration was confirmed by deriving to dihydrocorynantheol according to a literature16 procedure. Specific rotation of the dihydrocorynantheol thus obtained was [α]24D -9.03 (c 0.080, CHCl3); {lit.,16 [α]22D -14.2 (c 0.921, CHCl3)}.
Spirooxindole 8
To a solution of a single isomer 5 (70 mg, 0.26 mmol) in THF/H2O/AcOH=1/1/1 (6 mL) was added NBS (51 mg, 0.29 mmol) at 0 ºC over 23 min, and the solution was stirred for 1 h. The reaction mixture was made basic with saturated aqueous NaHCO3 solution and the aqueous layer was extracted with AcOEt. The combined organic layers were dried over MgSO4 and evaporated off. The residue was purified by chromatography on silica gel (AcOEt:hexane =5:1) to give compound 8 (60 mg) in 80% yield: pale yellow amorphous; 1H-NMR (500 MHz CDCl3) δ: 0.94 (3H, t, J=7.4 Hz), 1.20-1.30 (1H, m), 1.76-1.82 (1H, m), 1.86-1.94 (1H, m), 2.43 (1H, t, J=12.0 Hz), 2.50-2.56 (1H, m), 2.65 (1H, dt, J=14.9 Hz, 2.3 Hz), 2.85 (2H, dd, J=14.3 Hz, 3.4 Hz), 2.92-3.06 (3H, m), 3.32 (1H, dd, J=10.9 Hz, 5.2 Hz), 3.90 (1H, dd, J=11.5 Hz, 3.4 Hz), 7.31 (1H, t, J=7.4 Hz), 7.40 (1H, td, J=7.4 Hz, 1.1 Hz), 7.53 (1H, d, J=7.4 Hz), 7.63 (1H, d, J=7.4 Hz); 13C-NMR (125 MHz CDCl3) δ: 11.6, 19.3, 37.4, 42.5, 50.2, 51.2, 59.1, 59.7, 60.1, 121.8, 123.0, 127.0, 130.0, 140.8, 151.8, 178.5, 208.6; HR-FAB MS: Calcd for C17H21O2N2 [M+H]+: 285.1603. Found: 285.1598.
Spirooxindole 9
To a solution of compound 6 (28 mg, 87 μmol) in THF/H2O/AcOH=1/1/1 (3 mL) was added NBS (17 mg, 96 μmol) at 0 ºC over 15 min, and the solution was stirred for 1 h. The reaction mixture was diluted with H2O, and K2CO3 was added to neutralize the solution. The aqueous solution was extracted with
ethyl acetate, and the combined organic layer were dried over MgSO4 and evaporated off. The residue was purified by chromatography on silica gel (CHCl3:Et2O=4:1) to give compound 9 in 57% yield: pale yellow amorphous; [α]24D +37.7 (c 0.61, CHCl3); 1H-NMR (400 MHz CDCl3) δ: 1.00 (3H, t, J =7.6), 1.31 (1H, septed, J =7.6), 1.68-1.83 (2H, m), 2.17 (1H, t, J =10.8), 2.26-2.32 (1H, m), 2.51 (1H, t, J =12.9), 2.61 (1H, td, J =2.2, 14.9), 2.86-2.89 (2H, m), 3.22 (1H, dd, J = 4.0, 10.4), 3.64 (1H, dd, J = 3.0, 11.4), 3.73 (3H, s), 4.41 (1H, dd, J = 3.0, 13.6), 5.72 (1H, s), 7.30 (1H, dd, J = 0.7, 7.4), 7.39 (1H, td, J = 1.2, 7.4), 7.50 (1H, dd, J = 0.7, 8.4), 7.65 (1H, d, J =7.8); 13C-NMR (100 MHz CDCl3) δ: 11.6, 21.7, 31.3, 37.8, 45.2, 50.4, 51.2, 60.1, 60.6, 61.3, 112.5, 121.7, 122.9, 126.7, 129.9, 140.8, 152.2, 160.1, 167.1, 179.0; HR-FAB MS: Calcd for C20H25O3N2 [M+H]+: 341.1865. Found: 341.1873.
ACKNOWLEDGEMENTS
This work was supported in part by Grants-in-Aid for Scientific Research and the High-Technology Research Center Project from the Ministry of Education, Culture, Sports, Science and Technology, Japan.
References
1. (a) T. Itoh, Y. Matsuya, Y. Enomoto, and A. Ohsawa, Tetrahedron, 2001, 57, 7277; CrossRef (b) T. Itoh, M. Miyazaki, S. Ikeda, K. Nagata, M. Yokoya, Y. Matsuya, Y. Enomoto, and A. Ohsawa, Tetrahedron, 2003, 59, 3527. CrossRef
2. (a) T. Itoh, M. Yokoya, K. Miyauchi, K. Nagata, and A. Ohsawa, Org. Lett., 2003, 5, 4301; CrossRef (b) T. Itoh, M. Yokoya, K. Miyauchi, K. Nagata, and A. Ohsawa, Org. Lett., 2006, 8, 1533. CrossRef
3. M. M. Janot and R. Goutarel, Compt. Rend., 1938, 206, 1183.
4. (a) L. F. Tietze and Y. Zhou, Angew. Chem. Int. Ed., 1999, 38, 2045; CrossRef (b) M. Amat, A. Gómez-Esqué, C. Escolano, M. M. M. Santos, E. Molins, and J. Bosch, J. Org. Chem., 2009, 74, 1205. CrossRef
5. H. Kondo and T. Ikeda, J. Pharm. Soc. Japan, 1937, 57, 881.
6. R. L. Beard and A. I. Meyers, J. Org. Chem., 1991, 56, 2091. CrossRef
7. Although the other product obtained was likely to be an epimor at C(20), its structure was not precisely determined, because the compound was difficult to be isolated as a pure form.
8. J. A. Weisbach, J. L. Kirkpatrick, K. R. Williams, E. L. Anderson, N. C. Yim, and B. Douglas, Tetrahedron Lett., 1965, 3457. CrossRef
9. (a) J. S. Shi, G. X. Liu, Q. Wu, Y. P. Huang, and X. D. Zhang, Acta Pharmacol. Sin., 1992, 13, 35; (b) J. S. Shi, G. X. Liu, Q. Wu, W. Zhang, and X. N. Huang, Chi. J. Pharmacol. Toxicol., 1989, 3, 205.
10. Y. Shimada, H. Goto, T. Itoh, I. Sakakibara, M. Kubo, H. Sasaki, and K. Terasawa, J. Pharm. Pharmacol., 1999, 51, 715. CrossRef
11. Y. Ban, M. Seto, and T. Oishi, Chem. Pharm. Bull., 1975, 23, 2605. CrossRef
12. A. Deiters, M. Pettersson, and S. F. Martin, J. Org. Chem., 2006, 71, 6547. CrossRef
13. N. Finch and W. I. Taylor, J. Am. Chem. Soc., 1962, 84, 3871. CrossRef
14. (a) C. Pellegrini, C. Strässler, M. Weber, and H. J. Borschberg, Tetrahedron: Asymmetry, 1994, 5, 1979; CrossRef (b) C. Pellegrini, M. Weber, and H. J. Borschberg, Helv. Chim. Acta, 1996, 79, 151. CrossRef
15. (a) E. E. van Tamelen, J. P. Yardley, M. Miyano, and W. B. Hinshaw, Jr., J. Am. Chem. Soc., 1969, 91, 7333; CrossRef (b) S. D. Edmondson and S. J. Danishefsky, Angew. Chem. Int. Ed., 1998, 37, 1138; CrossRef (c) S. D. Edmondson, S. J. Danishefsky, L. Sepp-Lorenzino, and N. Rosen, J. Am. Chem. Soc., 1999, 121, 2147. CrossRef
16. M. Ohba, T. Ohashi, and T. Fujii, Heterocycles, 1991, 32, 319. CrossRef