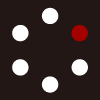
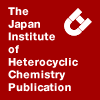
HETEROCYCLES
An International Journal for Reviews and Communications in Heterocyclic ChemistryWeb Edition ISSN: 1881-0942
Published online by The Japan Institute of Heterocyclic Chemistry
e-Journal
Full Text HTML
Received, 8th September, 2009, Accepted, 5th October, 2009, Published online, 5th October, 2009.
DOI: 10.3987/COM-09-S(S)129
■ Synthetic Studies Connected with the Preparation of N-[3-(3-Cyanopyrazolo[1,5-a]pyrimidin-5-yl)phenyl]-N-ethylacetamide, a Zaleplon Regioisomer
Stanislav Rádl,* Michaela Blahovcová, Marcela Tkadlecová, and Jaroslav Havlíček
Organic Synthesis, Zentiva, U Kabelovny 130, 102 01 Prague 10, Czech Republic
Abstract
N-[3-(3-Cyanopyrazolo[1,5-a]pyrimidin-5-yl)phenyl]-N-ethylacetamide, a principal impurity of zaleplon, is prepared by Suzuki-Miyaura cross coupling reaction of the corresponding boronic acid and/or boronates with 5-chloropyrazolo[1,5-a]pyrimidin-3-carbonitrile (7). Various methods of preparation of both components are described, as well as approaches based on the final modification of the 5-(3-aminophenyl)-pyrazolo[1,5-a]pyrimidine-3-carbonitrile moiety prepared by Suzuki-Miyaura cross coupling. All the prepared compounds were unambiguouesly identified by NMR techniques. Spectral characteristics (IR, UV, MS) of these compounds are also given.INTRODUCTION
Zaleplon (1) is a nonbenzodiazepine hypnotic belonging with zolpidem and zopiclon to the so called Z-hypnotic class.1,2 Clinical results have shown that zaleplon is efficacious in the treatment of insomnia where difficulty in falling asleep is the primary problem. Zaleplon unlike many other hypnotic drugs does not interfere with sleep architecture and can be administered for up to 5 weeks without the risk of dependence or rebound insomnia upon discontinuation.3
Most of the described methods4-7 of preparation of zaleplon are based on reaction of N-[3-(3-dimethyl- amino-acryloyl)-phenyl]-N-ethyl-acetamide (2) with 5-amino-1H-pyrazol-4-carbonitrile (3a) under acidic conditions. The original patent4 describes the reaction in anhydrous acetic acid, but under these conditions considerable amounts of the corresponding isomer 4 is formed. Much better results are achieved using aqueous acetic5 or formic6 acids. Probably the best results regarding purity and yields are obtained when the reaction is done in aqueous alcohols in the presence of hydrochloric acid7,8 (Scheme 1).
One of the principal parts of documentation of any active pharmaceutical ingredient (API) is description of impurities and/or degradation products which can be present. Identified impurities should be included in the specification when they are present at a level higher than the identification threshold, which is usually 0.10 %. These impurities must be not only identified but also either isolated or independently synthesized for determination of their response factor for analytical determination.
Several impurities of zaleplon, including zaleplon regioisomer 4, have recently been isolated and identified.9 To the best of our knowledge, no report on its synthesis has been published and therefore we decided to synthetize this impurity as a standard.
RESULTS AND DISCUSSION
Our retrosynthetic analysis is shown in Scheme 2. Our approach is based on Suzuki-Miyaura cross-coupling reaction of boronic acids 6, containing suitable substituent X, with 5-chloropyrazolo[1,5-a]pyrimidine-3-carbonitrile (7), which should be easily obtained from the corresponding oxo derivative.
It is well documented10-16 that reaction of 5-amino-1H-pyrazoles 3 with formylacetates, β-ketoesters or their equivalents, e.g., ethoxymethylenemalonates, provides the corresponding pyrazolo[1,5-a]- pyrimidin-7(4H)-ones 8, 9, and 10, respectively. Similarly, reaction of 5-amino-1H-pyrazol-4-carbonitrile
(3a) with ethyl propiolate is reported15,16 to provide 7-oxo derivative 8a. However, similar reports on pyrazolo[1,5-a]pyrimidin-5(4H)-ones are really scarce. Recently Gavrin et al.14 reported that 5-amino-1H-pyrazoles 3 treated with ethyl 3-ethoxyacrylate in the presence of cesium carbonate yielded exclusively the corresponding 5-oxo derivatives 11 (Scheme 3).
Our synthesis of 7 started from commercially available 5-amino-1H-pyrazol-4-carbonitrile (3a), which treated with ethyl 3-ethoxyacrylate in the presence of cesium carbonate yielded the 5-oxo derivative 11a. Its structure was fully proven by NMR techniques and the data was in accordance with data described for similar compounds.17 Treatment of compound 11a with phosphorus oxychloride provided intermediate 7 in good yields (Scheme 4).
Comercially available boronic acids 6a and 6b were chosen as the suitable intermediates. 3-Nitrophenylboronic acid (6a) treated with 7 using (Ph3P)4Pd, Na2CO3 in DMF at 100 °C, provided good yield of the corresponding coupling product 5a. Due to the limited solubility of this compound even in DMF and DMSO, we failed to reduce the compound to the corresponding amino derivative 5b. In the literature, there are several examples of successful Suzuki-Miyaura cross-coupling reactions using 3-aminophenylboronic acid (6b). When we applied the standard conditions used by Jagusch et al.,18 a complex mixture not containing the required product (LCMS) was obtained. There are several reports on successful application of aqueous Suzuki-Miyaura cross-coupling reactions with 3-aminophenylboronic acid (6b) using water-soluble ligands, for example sodium 2-(dicyclohexylphosphino)- -2',6'-dimethoxybiphenyl-3'-sulfonate19 or trisodium triphenylphosphine- -3,3‘,3‘‘-trisulphonate (TPPTS).20,21 We have applied the latter conditions and obtained good yields of 5b as the single product. This compound was again insoluble in suitable solvents and attempts to acetylate it by heating in a mixture of acetic anhydride and acetic acid provided a complex mixture containing only traces of acetyl derivative 5c. However, stirring a suspension of 5b in acetic anhydride provided moderate yields of the desired product.
3-Aminophenylboronic acid (6b) was acetylated with acetic anhydride to the corresponding N-acetyl derivative 6c. Its Suzuki-Miyaura cross-coupling reaction with 7 in aqueous acetonitrile provided compound 5c. Its alkylation with iodoethane after generating the corresponding sodium salt in situ by sodium hydride in DMF led to the zaleplon regioisomer 4 (Scheme 5).
Attempts to prepare N-ethyl derivative 6d by treating 6c consecutivelly with excess of sodium hydride followed by iodoethane failed. However, when a suspension of 6c in dichloromethane was treated with anhydrous pinacol at ambient temperature, the corresponding pinacol ester 12 was quantitatively formed and its alkylation using NaH/EtI provided compound 13. Suzuki-Miyaura cross-coupling reaction of 13 with 7 catalyzed with (Ph3P)4Pd then provided good yields of the zaleplon regioisomer 4 (Scheme 6).
In order to develop an alternative way to either boronic acid 6d or its pinacol ester 13, we started with 3-bromoaniline 14, which was acetylated with acetic anhydride and the corresponding anilide 15 was then alkylated with iodoethane to provide compound 16. Our attempts of transformation of this compound to 6d using one equivalent of butyllithium led nearly exclusively to deacetylation giving 17, while using two equivalents of the base led to a complex mixture (Scheme 5). Compound 17 was also prepared by acid hydrolysis of 16 (Scheme 7).
After the failure, we decided to protect the NH group in 17 by a suitable group. Surprisingly, the subject has not been widely studied. Schrer et al. prepared anilineboronic acids by converting the halogen substituted aniline to the corresponding N,N-dibenzyl derivatives, treating these intermediates subsequently with a metallating agent and a boronic ester.22 A recent report23 describing procedure of preparation of 4-amino-3-fluorophenylboronic acid transformed the starting 4-bromo-2-fluoro aniline into the corresponding bis-TMS derivative by treatment with two equivalents of butyllithium followed by addition of TMSCl. However, under these condition using one equivalent of butyllithium, partial lithium-bromine exchange in 17 occurred. Therefore we tried to utilize milder conditions using TMSCl and Et3N24 or TMSA,25 but in both cases only partial silylation was observed.
An alternative method of preparing boronic acid derivatives is the transition metal-catalyzed coupling of pinacolborane with aromatic halides. Original procedure26 using PdCl2(dppf) [dpff = 1.1‘-bis(diphenylphosphino)ferrocene] is problematic for partial aryl halide reduction. An improved procedure using Pd(dba)2/bis(2-di-tert-butylphosphinophenyl)ether (t-Bu-DPEPhos) as the catalyst is reported to substantially suppress this side reaction.27 Using this system, the product contained according to GC 77.4 % of 13 and 11.3 % of the reductive product 18 (Scheme 8). Similar results were obtained with analogous 9,9-dimethyl-4,5-bis(di-tert-butylphosphino)xanthene (t-butyl-XantPhos). We have also applied a procedure using SPhos, which has just been pusblished.28 However, even higher amount of 18 was present in the mixture. Using 2-dicyclohexylphosphino-2′,4′,6′-triisopropylbiphenyl (XPhos) under analogous conditions, formation of 18 was suppressed but the mixture contained three major impurities in amounts higher than 10 % (Table 1).
Mixtures obtained by the method (entries 1-3) were used for the Suzuki-Miyaura cross-coupling reaction with 7 catalyzed with (Ph3P)4Pd (Scheme 6). The obtained yields were lower than using pure 13, which can be caused either by the present impurities or by lower content of 13 than indicated by GC.
Broutin et al. reported one-pot preparation of unsymmetric biaryls based on palladium-catalyzed borylation of phenyl bromides in the presence of Pd(OAc)2 and DPEPhos folowed by Suzuki-Miyaura coupling.29 We also tried to prepare compound 4 by application of this strategy starting from 16, pinacolborane, SPhos in the first step and using chloro derivative 7 in the second step, but the yields were substantially lower than reported by Broutin.
Kang et al. recently published33 a procedure of Pd-catalyzed direct arylation of tautomerizable heterocycles with aryl boronic acids via C-OH bond activation using phosphonium salts. First we checked the methodology using the described conditions with oxo derivative 11a and 3-nitrophenylboronic acid (6a). The crude product contained according the TLC the required product 5a and an unknown impurity in about 2 : 1 ratio and due to the low solubility our attempts to purify it failed. Then we applied this method to the reaction of 11a with pinacol ester 13 using bromotris(pyrrolidino)phosphonium hexafluorophosphate (PyBroP) as the phosphonium salt. While the original procedure uses 2 equivalents of the boronic acids, we used only slight excess (1.05 eq.) of the boronic ester 13 and we obtained 75 % yield of the required compound 4. The only disadvantage of the procedure seems to be the price of the reagent.
Spectral characteristics (IR, UV, MS, 1H NMR, 13C NMR) of all new compounds have been measured. Having both zaleplon (1) and isozaleplon (4) in hands, we decided to compare their NMR spectral characteristics in details using 1H NMR (500 MHz) and 13C NMR (125 MHz). The assignment of protons and carbons for both compounds was performed using 2D NMR techniques. Both proton and carbon spectra are very similar; comparison of aromatic regions of proton spectra for isozaleplon (4) and zaleplon (1) is shown in Figure 1. The most important difference is the value of the coupling constant (7.4 Hz) of the protons 6 and 7 of isozaleplon (4) while the corresponding value for protons 5 and 6 of zaleplon (1) is significantly lower (4.4 Hz) and was confirmed in many zaleplon analogues.34
Unfortunately the 1H,15N HMBC NMR spectra did not enable the assignment of all nitrogen signals because of their overlap; only assignment of the amide nitrogen at 139.1 ppm (for NH4+ referencing) is unambiguous.
CONCLUSIONS
Several approaches to the synthesis of isozaleplon (4), a principal impurity of zaleplon, was developed. The best results were obtained using Suzuki-Miyaura cross coupling reaction of pinacol ester 13 with 5-chloropyrazolo[1,5-a]pyrimidin-3-carbonitrile (7). Various methods of preparation of both components are described, as well as approaches based on the final modification of the 5-(3-aminophenyl)-pyrazolo[1,5-a]pyrimidine-3-carbonitrile moiety prepared by Suzuki-Miyaura cross coupling. All the prepared compounds were unambiguouesly identified by NMR techniques. Spectral characteristics (IR, UV, MS) of these compounds are also given.
EXPERIMENTAL
2,2-Bis(di-tert-butylphosphino)diphenyl ether (t-Bu-DPEPhos) vas prepared according to the literature procedure;30 the product was purified by bulb-to-bulb distillation. t-Bu-XantPhos was obtained from Johnson Matthey. 3-Nitrophenylboronic acid (6a), 3-aminophenylboronic acid monohydrate (6b), triphenylphosphine-3,3’,3’’-trisulfonic acid trisodium salt hydrate (TPPTS), and pinacol anhydrous were purchased from Alfa Aesar. Other chemicals used in the synthesis were purchased from Sigma-Aldrich and were used without purification.
Melting points were measured on a Kofler block and are uncorrected. The IR spectra were measured on a Perkin Elmer Spectrum BX FT-IR machine by the diffuse reflectance method (KBr), wavenumbers are given in cm-1. The UV spectra were recorded on a Hewlett-Packard 8452A spectrophotometer (ethanol) in the range 190-400 nm. NMR experiments were carried out on a Bruker Avance 500 (Bruker Biospin GmbH) at 500.13 MHz (1H), 125.77 MHz (13C) and 50.70 MHz (15N) respectively. All experiments were performed in CDCl3 or DMSO-d6 at 298K. COSY, HSQC, 1H,13C HMBC and 1H, 15N HMBC spectra were recorded using pulse programs from the Bruker NMR standard library. At 500 MHz, standard 5 mm TXO (triple-nucleus X-observe) and TBI (triple-broadband inverse) probeheads equipped with z-gradient coils were employed for all measurements. For the 1H-13C HSQC, a dataset was acquired with 8 scans for each t1 increment at a resolution of 2048 and 256 points in the F2 and F1 dimensions, respectively. The time domain data was zero-filled to 2048 and 1024 data points in F2 and F1 dimensions, and multiplied with a sinusoidal squared sine-bell window function in both dimensions prior to Fourier transform. The gradient-selected 1H-13C HMBC and 1H-15N HMBC data sets were recorded with 4K and 512 points in the F2 and F1 dimensions, respectively. The magnetization transfer in the 1H-13C HMBC experiment was optimized for a three-bond coupling constant 3J(C,H) of 8 Hz. The corresponding 1H-15N HMBC was optimized for a long range coupling constant of 4 Hz. 1K increments of 2K data points were recorded with 128 scans for each increment. The data was subsequently processed employing zero-filling to 2K and 1K data points in the F1 and F2 dimensions, using a sinusoidal squared sine-bell window function for apodization prior to Fourier transform in both dimensions.
The Mass spectra (MS/MS; ionization mode APCI(+)) were measured on an API 3000 PE machine (Sciex Instruments, Applied Biosystems). The purity of the prepared substances was evaluated by TLC on silica gel (FP KG F 254, Merck). Preparative TLC was done on pre-coated silica plates Merck 60 F254, layer thickness 2 mm. Flash chromatography was performed on silica gel Merck, particle size 0.04-0.063 mm. Centrifugally accelerated axial chromatography was done using CyclographTM instrument (Analtech) with silica gel pre-scraped rotors.
N-(3-Bromophenyl)acetamide (15)
3-Bromoaniline (68.8 g, 0.4 mol) was added dropwise during 1 h into the stirred mixture of acetic anhydride (80 mL) and acetic acid (80 mL) and the mixture was stirred at ambient temperature for 1 h. Residue after evaporation was crystallized from a mixture of EtOH-water (1 : 1) to give 63.2 g (73.8 %) of white crystals; mp 82-84 °C (mp 87 °C)31.
N-(3-Bromophenyl)-N-ethylacetamide (16)
A solution of 15 (58.9 g, 0.275 mol) in DMF (250 mL) was added dropwise to a stirred mixture of 50% sodium hydride (16.7 g, 0.35 mol) and DMF (500 mL) under nitrogen and the mixture was stirred at ambient temperature for 1 h. Then the mixture was cooled at 0°C and a solution of iodoethane (56 g, 0.36 mol) in DMF (50 mL) was added at this temperature. The mixture was then stirred at ambient temperature for 6 h, poured into water (1000 mL) and extracted with CH2Cl2. The extract was washed with water and dried with magnesium sulfate. The residue after evaporation was then distilled to give 46.8 g (70.3 %) of slightly yellowish oil, bp 88°C/12.4 Pa). HRMS for C10H13BrNO (M+H)+ Calcd: 242.01805, found: 242.01786.
3-Bromo-N-ethylaniline (17)
A mixture of N-(3-bromophenyl)-N-ethylacetamide (16; 38.2 g, 0.158 mol), EtOH (160 mL) and concentrated HCl (160 mL) was refluxed for 24 h. The mixture was evaporated, dissolved in hot water, cooled and alkalinized with 10% NaOH. The mixture was extracted with Et2O, the extract was dried with magnesium sulfate and the residue after evaporation was distilled to give 25.1 g (78.8 %) of yellowish oil bp 62-64°C/4.8 Pa. HRMS for C8H11BrN (M+H)+ Calcd: 200.00749, found: 200.00731. 1H NMR (500.13 MHz, CDCl3) δ, ppm: 1.27 t, 3H, J = 7.2 (CH3); 3.15 q, 2H, J = 7.2 (CH2); 3.69 s, 1H (NH); 6.51-6.86 m, 3H (Ar-H); 7.02 t, 1H, J = (H-5).
3-Bromo-N-ethylaniline Hydrochloride (17.HCl)
A mixture of N-(3-bromphenyl)-N-ethylacetamide (16; 5 g, 20.7 mmol), EtOH (20 mL) and 20% HCl (10 mL) was refluxed for 48 h. The mixture was evaporated and crystallization of the residue from EtOH provided 3.5 g of white crystals (71.5 %); mp 133-140 °C. Anal. Calcd for C8H11BrClN (236.54): C, 40.62; H, 4.69; N, 5.92. Found: C, 40.28; H, 4.72; N, 5.74.
3-Acetamidophenylboronic acid (6c)
A mixture of 3-aminophenylboronic acid monohydrate (6a; 3.1 g, 20 mmol), Ac2O (6 mL), acetic acid (20 mL), and 4-dimethylaminopyridine (0.05 g) was stirred at ambient temperature for 24 h. The formed suspension was evaporated in vacuo and the residue was triturated with Et2O. The insoluble portion was filtered off, washed with Et2O and dried to give 3.5 g (97.8 %) of white solid, mp 286-288 °C [mp 274-275 °C (H2O) 32]. Anal. Calcd for C8H10BNO3 (178.98): C, 53.68; H, 5.63; N, 7.83. Found: C, 53.49; H, 5.87; N, 7.50. HRMS for C8H11BNO3 (M+H)+ Calcd: 180.08320, found: 180.08247. 1H NMR (500.13 MHz, DMSO-d6): 2.04 s, 3H (CH3), 7,21-7,95 m, 4H (Ar-H), 9.81-9.87 m, 2H (2xOH), 11.92 bs, 1H (NH). 13C NMR (125.77 MHz, DMSO): 23.94, 120.55, 124.33, 127.58, 128.32, 138.55, 168.15, 171.98. IR (KBr): ν(NH) 3306, ν(C=O) 1710, 1652, ν(C=C) + ν(C=N) 1486, 1414, δ(NH) 1553, ν(BO) 1339, 1258, δ(CH) 721 cm-1. UV λmax (log ε): 212 (4.48), 244 (4.12), 284 (3.51).
N-(3-(4,4,5,5-Tetramethyl-1,3,2-dioxaborolan-2-yl)phenyl)acetamide (12)
Pinacol anhydrous (1.2 g, 10 mmol) was added to a stirred suspension of 3-acetamidophenylboronic acid (6c; 1.79 g, 10 mmol) in CH2Cl2 (50 mL) and the mixture was stirred at ambient temperature for 10 h. The formed clear solution was evaporated, the residue was triturated with boiling hexane and the insoluble solid was filtered off to give 2.4 g of white crystals (91.9 %); mp 188-190 °C. Anal. Calcd for C14H20BNO3 (261.12): C, 64.39; H, 7.72; N, 5.36. Found: C, 64.13; H, 7.56; N, 5.21. HRMS for C14H21BNO3 (M+H)+ Calcd: 262.16145, found: 262.16086. 1H NMR (500.13 MHz, CDCl3) δ, ppm: 1.32 s, 12H (CH3), 2.15 s, 3H (CH3CO), 7,32-7,70 m, 4H (Ar-H), 11.02 bs, 1H (NH). 13C NMR (125.77 MHz, CDCl3): 24.41, 24.81, 83.84, 123.13, 125.95, 128.44, 130.49, 137.44, 168.53, 172.13. IR (KBr): ν(NH) 3322, ν(CH) 2975, ν(C=O) 1661, δ(NH) 1548, ν(COBOC) 1355, 1138 cm-1. UV λmax (log ε): 216 (4.49), 246 (4.08), 286 (3.02).
N-Ethyl-N-(3-(4,4,5,5-tetramethyl-1,3,2-dioxaborolan-2-yl)phenyl)acetamide (13)
Method A) Sodium hydride (0.3 g, 50 % dispersion, 6.25 mmol) was added to a solution of N-(3-(4,4,5,5-tetramethyl-1,3,2-dioxaborolan-2-yl)phenyl)acetamide (12; 1.3 g, 5 mmol) in dry THF (20 mL) and the mixture was stirred under nitrogen for 1 h. Then iodoethane (1 mL, 12.5 mmol) was added, the flask was closed by a stopper and the mixture was stirred for 24 h at ambient temperature. The mixture was evaporated, the residue was mixed with water (25 mL) and extracted with CH2Cl2. The extract was washed with brine and dried with anhydrous magnesium sulfate. The oily residue after evaporation (1.05 g, 72.6 %) was analyzed by LC-MS and used for the next step without purification. HRMS for C16H25BNO3 (M+H) + Calcd: 290.19275, found: 290.19212.
Method B) A flask with a septum inlet was charged with Pd(dba)2 (56 mg, 0.1 mmol) and t-Bu-DPEPhos (50 mg, 0.1 mmol) and then flushed with argon. Dry dioxane (20 mL) and Et3N (4 mL, 28 mmol) were added via syringe, followed by N-(3-bromophenyl)-N-ethylacetamide (16; 2.42 g, 10 mmol) and pinacolborane (2.2 mL, 15 mmol). The mixture was then stirred for 24 h at 80 °C and the mixture was analyzed by GC. Et2O (50 mL) was added and the solution was washed with brine (4 x 10 mL), dried with magnesium sulfate and evaporated to give 2.2 g of oily residue containing 77.4 % of 13 and 11.3 % of 18 (GC).
Method C) Analogously as Method B, using t-Butyl-XantPhos (48 mg, 0.1 mmol), 2.4 g of an oily residue was obtained. According to GC, the mixture contained 73.5 % of 13 and 12.8 % of 18.
Method D) A pressure tube closed by a septum was charged with PdCl2(MeCN)2 (50 mg, 0.2 mmol) and SPhos (100 mg, 0.25 mmol) and then flushed with argon. Dry dioxane (15 mL) was added via syringe, followed by a solution of N-(3-bromophenyl)-N-ethylacetamide (16, 2.42 g, 10 mmol) in dry dioxane (5 mL), Et3N (4 mL, 28 mmol) and pinacolborane (2.2 mL, 15 mmol). The septum was then replaced with a teflon screw valve and the mixture was then stirred for 4 h at 110 °C. The mixture was filtered through a pad of celite, eluted with Et2O (10 mL) and the filtrate was diluted with Et2O (40 mL). The solution was washed with brine (4 x 10 mL), dried with magnesium sulfate and evaporated to give 2.1 g of oily residue containing 69.6 % of 13 and 22.7 % of 18 (GC).
Method E) Analogously as Method D, using XPhos (48 mg, 0.1 mmol), 2.3 g of an oily residue was obtained. According to GC, the mixture contained 37.7 % of 13 and 0.7 % of 18; however, the mixture contained also three major impurities in amounts higher than 10 %.
5-Oxo-4,5-dihydropyrazolo[1,5-a]pyrimidin-3-carbonitrile (11a)
Ethyl 3-ethoxyacrylate (5 mL, 35 mmol) and cesium carbonate (11.4 g, 35 mmol) were consecutively added to a stirred solution of 5-amino-1H-pyrazole-4-carbonitrile (3a; 2.45 g, 22.7 mmol) in DMF (115 mL) and the mixture was stirred under nitrogen at 110 °C for 2 h. Then the mixture was evaporated, the residue was dissolved in water (100 mL) and after addition of crushed ice (30 g) the solution was acidified with 10% HCl. After cessation of carbon dioxide evolution, the formed solid was filtered off and washed with cold water to give 4.5 g of white solid (80.3 %); mp 293-305 °C. Anal. Calcd for C7H4N4O (160.13): C, 52.50; H, 2.52; N, 34.99. Found: C, 52.67; H, 2.71; N, 34.52. HRMS for C7H5N4O (M+H) + Calcd: 161.04634, found: 161.04596. 1H NMR (500.13 MHz, DMSO-d6) δ, ppm: 6.28 d, 1H, J = 7.3 (H-6), 8.68 s, 1H (H-2), 8.73 d, 1H, J = 7.3 (H-7). 13C NMR (125.77 MHz, DMSO-d6) δ, ppm: 74.60, 107.54, 112.62, 138.78, 142.33, 145.62, 160.68. IR (KBr): ν(CH) 3073, 2929, 2882, ν(CN) 2237, ν(C=O) 1705, 1680, ν(C=C) + ν(C=N) 1586, 1507, 1475, ν(C-N), 1355, 1181, δ(CH) 826 cm-1 . UV λmax (log ε): 208 (4.15), 234 (4.45), 264 (3.93).
5-Chloropyrazolo[1,5-a]pyrimidin-3-carbonitrile (7)
A mixture of 5-oxo-4,5-dihydropyrazolo[1,5-a]pyrimidin-3-carbonitrile (11a; 1.6 g, 10 mmol) and phosphorus oxychloride (10 mL) was stirred in a pressure tube at 150 °C for 1h. The mixture was evaporated under reduced pressure, crushed ice was added to the residue and the mixture was alkalinized with 20% aqueous sodium hydroxide. The formed solid was filtered off, washed with water and crystallized from 2-propanol (charcoal) to give 1.1 g (62.6 %) of white crystals; mp 138-142 °C. Anal. Calcd for C7H3ClN4 (178.58): C, 47.08; H, 1.69; Cl, 19.85; N, 31.37. Found: C, 46.88; H, 1.51; Cl, 19.53; N, 31.42. HRMS for C7H4ClN4 (M+H) + Calcd: 179.01245, found: 179.01307. 1H NMR (500.13 MHz, DMSO-d6) δ, ppm: 7.50 d, 1H, J = 7.3 (H-6), 8.85 s, 1H (H-2), 9.41 d, 1H, J = 7.3 (H-7). 13C NMR (125.77 MHz, DMSO-d6) δ, ppm: 80.98, 112.24, 112.64, 139.82, 148.33, 148.90, 154.40. IR (KBr): ν(CH) 3109, 3091, ν(CN) 2233, ν(C=C) + ν (C=N) 1619, 1543, 1514, 1401, ν(CCl) 1074, δ(CH) 814 cm-1. UV λmax (log ε): 208 (4.21), 234 (4.51), 266 (3.85).
5-(3-Nitrophenyl)pyrazolo[1,5-a]pyrimidin-3-carbonitrile (5a)
A mixture of 3-nitrophenylboronic acid (6a; 0.5 g, 3 mmol), 5-chloropyrazolo- [1,5-a]pyrimidin-3-carbonitrile (7, 0.5 g, 2.8 mmol), sodium carbonate (0.75 g) and tetrakistriphenylphosphinepalladium (0.1 g) in DMF (15 mL) was stirred under argon at 100 ºC for 3 days. Then the mixture was poured into a 10% aqueous solution of sodium carbonate (50 mL) and stirred at ambient temperature overnight. The insoluble portion was filtered off, the beige paste was stirred with water (25 mL) for 2 h and the insoluble portion was again filtered off to give yellow crystals (0.54 g, 67.9 %), mp 272-278 °C. Anal. Calcd for C13H7N5O2 (265.23): C, 58.87; H, 2.66; N, 26.41. Found: C, 58.64; H, 2.87; N, 26.73. HRMS for C13H8N5O2 (M+H) + Calcd: 266.06780, found: 266.06823. 1H NMR (500.13 MHz, DMSO-d6) δ, ppm: 7.82-8.15 m, 1H (Ar-H), 8.20 d, 1H, J = 7.4 (H-6), 8.30-8.80 m, 2H (Ar-H), 8.84 s, 1H (H-2), 9.02 s, 1H (H-2’), 9.53 d, 1H, J = 7.4 (H-7). 13C NMR (125.77 MHz, DMSO-d6) δ, ppm: 81.32, 108.46, 113.27, 122.03, 123.12, 125.88, 130.82, 131.49, 133.88, 136.88, 148.46, 149.32, 156.91. IR (KBr): ν(CH) 3078, ν(CN) 2235, ν(C=C) + ν(C=N) 1613, ν(NO) 1518, ν(NO) + ν(CN) 1355, δ(CH) 738 cm-1. UV λmax (log ε): 218 (4.35), 258 (4.48), 300 (3.98).
5-(3-Aminophenyl)pyrazolo[1,5-a]pyrimidine-3-carbonitrile (5b)
A solution of palladium acetate (0.056 g, 0.29 mmol), TPPTS hydrate (0.71 g) in a mixture of water (10 mL) and MeCN (5 mL) was added to a stirred solution of 3-aminophenylboronic acid monohydrate (6a; 0.78 g, 5 mmol), 5-chloropyrazolo[1,5-a]pyrimidin-3-carbonitrile (7, 0.86 g, 4.8 mmol), and cesium carbonate (4 g) in a mixture of water (20 mL) and MeCN (15 mL) and the mixture was refluxed for 1 h under argon. The cold reaction mixture was diluted with water (50 mL), the mixture was stirred at 0 °C for 2h and the insoluble portion was filtered off, washed with cold water (20 mL) and dried to give 1.05 g (89.3 %) of yellowish crystals, mp 160-165 °C. A small sample was crystallized from a mixture of MeCN - DMF 9 : 1 to give beige crystals, mp 164-166 °C. Anal. Calcd for C13H9N5 (235.24): C, 66.37; H, 3.86; N, 29.77. Found: C, 66.51; H, 3.72; N, 29.92. HRMS for C13H10N5 (M+H) + Calcd: 236.09362, found: 236.09719. 1H NMR (500.13 MHz, DMSO-d6) δ, ppm: 5.43 s, 2H (NH2), 6.80 ddd, 1H, J = 0.9; 2.4; 7.9 (H-4’), 7.23 t, 1H, J = 7.9 (H-5’), 7.37-7.53 m, 2H (Ar-H), 7.80 d, 1H, J = 7.4 (H-6), 8.77 s, 1H (H-2), 9.32 d, 1H, J = 7.4 (H-7). 13C NMR (125.77 MHz, DMSO-d6) δ, ppm: 80.54, 108.25, 112.12, 113.54, 113.65, 115.42, 117.34, 129.58, 137.67, 140.09, 148.02, 149.43, 159.98. IR (KBr): ν(NH) 3391, 3300, ν(CH) 2916, 2848, ν(CN) 2225, ν(C=C) + ν(C=N) + δ(NH) 1604, ν(C=C) + ν(C=N) 1550, 1410, δ(CH) 764 cm-1. UV λmax (log ε): 218 (4.41), 270 (4.37), 298 (4.08).
N-(3-(3-Cyanopyrazolo[1,5-a]pyrimidin-5-yl)phenyl)acetamide (5c)
Method A) A suspension of 5-(3-aminophenyl)pyrazolo[1,5-a]pyrimidine-3-carbonitrile (5b; 0.28 g, 1 mmol) in Ac2O (10 mL) was stirred at ambient temperature for 24 h. Then the mixture was diluted with ice water (50 mL), the mixture was stirred for 1 h, the insoluble portion was filtered off and dried to give 0.23 g (82.9 %) of beige solid; mp 253 – 255 °C. A small sample was crystallized from acetic acid to give white crystals, mp 255-256 °C.
Method B) A solution of palladium acetate (10 mg, 0.05 mmol), TPPTS hydrate (0.15 g) in a mixture of water (5 mL) and MeCN (2.5 mL) was added to a stirred solution of 3-acetamidophenylboronic acid (6c; 0.45 g, 2.5 mmol), 5-chloropyrazolo[1,5-a]pyrimidin-3-carbonitrile (7, 0.45 g, 2.5 mmol), and cesium carbonate (2 g) in a mixture of water (10 mL) and MeCN (5 mL) and the mixture was refluxed under argon for 2 h. The cold reaction mixture was diluted with water (25 mL), the insoluble portion was filtered off, washed with cold water (10 mL) and dried to give 0.65 g (93.8 %), mp 253-256 °C. Anal. Calcd for C15H11N5O (277.28): C, 64.97; H, 4.00; N, 25.26. Found: C, 64.39; H, 4.45; N, 25.55. HRMS for C15H12N5O (M+H)+ Calcd: 278.10419, found: 278.10355. 1H NMR (500.13 MHz, DMSO-d6) δ, ppm: 2.09 s, 3H (CH3), 7.51 t, 1H, J = 7.9 (Ar-H), 7.86 d, 1H, J = 7.4 (H-6), 7.90-8.42 m, 3H (Ar-H), 8.80 s, 1H (H-2), 9.38 d, 1H, J = 7.4 (H-7), 10.23 s, 1H (NH). 13C NMR (125.77 MHz, DMSO-d6) δ, ppm: 23.95, 80.81, 108.23, 113.54, 117.65, 122.12, 122.50, 129.52, 135.76, 138.05, 140.13, 148.20, 149.50, 159.06, 168.58. IR (KBr): ν(NH) 3340, ν(CH) 3072, ν(CN) 2227, ν(C=O) 1682, 1682, ν(C=C) + ν(C=N) 1620, 1596, δ(NH) 1551, δ(CH) 785 cm-1. UV λmax (log ε): 220 (4.36), 262 (4.55), 304 (4.00).
N-(3-(3-Cyanopyrazolo[1,5-a]pyrimidin-5-yl)phenyl)-N-ethylacetamide (4)
Method A) A 50% sodium hydride dispersion (0.1 g, 2 mmol) was added to a solution of N-(3-(3-cyanopyrazolo[1,5-a]pyrimidin-5-yl)phenyl)acetamide (5c; 0.28 g, 1 mmol) in DMF (3 mL) and the mixture was stirred at ambient temperature for 1 h. Then iodoethane (0.4 mL, 5 mmol) was added and the mixture was stirred at this temperature for 24 h. The reaction mixture was evaporated, mixed with water (5 mL) and extracted with CH2Cl2 (4 x 5 mL). The extract was washed with water (5 mL) and dried with magnesium sulfate. The residue after evaporation (0.4 g) was purified by centrifugally accelerated axial chromatography (CH2Cl2 – acetone 20 : 1 to 5 : 1 v/v) to give 0.17 g (55.7 %) of 4; mp 194-198 °C.
Method B) A mixture of N-ethyl-N-(3-(4,4,5,5-tetramethyl-1,3,2-dioxaborolan-2-yl)phenyl)acetamide (13; 0.28 g, 1 mmol), 5-chloropyrazolo[1,5-a]pyrimidin-3-carbonitrile (7; 0.18 g, 1 mmol), (Ph3P)4Pd (0.06 g, 5 mol %), sodium carbonate (0.25 g, 2.4 mmol) and toluene (3 mL) was stirred in a vial at 100 °C for 10 h. The mixture was evaporated, mixed with water (5 mL), extracted with CH2Cl2 (4 x 5 mL) and the extract was dried with magnesium sulfate. The residue after evaporation (0.45 g) was crystallized from MeOH to give 0.25 g (81.9 %) of 4; mp 197-199 °C. Anal. Calcd for C17H15N5O (305.33): C, 66.87; H, 4.95; N, 22.94. Found: C, 67.03; H, 5.09; N, 22.78. HRMS for C17H16N5O (M+H)+ Calcd: 306.13549, found: 306.13495. 1H NMR (500.13 MHz, CDCl3) δ, ppm: 1.17 t, 3H, J = 7.2 (CH3), 1.87 s, 3H, (CH3CO), 3.84 q, 2H, J = 7.2 (CH2), 7.38 d, 1H, J = 7.8 (H-4’), 7.54 d, 1H, J = 7.4 (H-6), 7.64 t, 1H, J = 7.8 (H-5’), 8.05 s, 1H (H-2’), 8.19 d, 1H, J = 7.8 (H-6’), 8.41 s, 1H (H-2), 8.82 d, 1H, J = 7.4 (H-7). 13C NMR (125.77 MHz, CDCl3) δ, ppm: 13.2 (CH3), 22.9 (CH3CO), 44.0 (CH2), 83.4 (C-3), 107.6 (C-6), 112.7 (CN), 127.1 (C-6’), 127.4 (C-2’), 130.6 (C-5’), 131.6 (C-4’), 136.5 (C-7), 137.4 (C-1’), 144.1 (C-3’), 148.2 (C-2), 149.9 (C-3a), 158.4 (C-5), 169.6 (CO). IR (KBr): ν(CH) 3103, 2922, 2853, ν(CN) 2227, ν(C=O) 1652, ν(C=C) + ν(C=N) 1626, 1601, 1553, 1522, δ(CH) 700 cm-1. UV λmax (log ε): 204 (4.40), 262 (4.48), 302 (3.95).
Method C) Products obtained by Methods B, C and D of preparation of N-ethyl-N-(3-(4,4,5,5-tetramethyl-1,3,2-dioxaborolan-2-yl)phenyl)acetamide containing 77.4 %, 73.5 % and 69.6 % of 13, respectively were used. A mixture of these products (0.4 g), 5-chloropyrazolo[1,5-a]pyrimidin-3-carbonitrile (7, 0.18 g, 1 mmol), (Ph3P)4Pd (0.05 g, mmol), sodium carbonate (0.25 g, mmol) and toluene (3 mL) was stirred in a vial at 100 °C for 10 h, then worked-up as in Method A to give 0.18-0.21 g of 4.
Method D) To a mixture of PdCl2(MeCN)2 (50 mg, 0.2 mmol), SPhos (100 mg, 0.25 mmol) and dry dioxane (20 mL) was added via syringe a solution of N-(3-bromophenyl)-N-ethylacetamide (16; 2.42 g, 10 mmol) in dry dioxane (5 mL), Et3N (4 mL, 28 mmol) and pinacolborane (2.2 mL, 15 mmol). The mixture was stirred for 4 h at 110 °C using a round-bottom flask with threaded joint. The mixture was stirred without heating for 1 h, then CsF (12.2 g, 80 mmol), Pd(OAc)2 (0.11 g, 5 mol %) and 5-chloropyrazolo[1,5-a]pyrimidin-3-carbonitrile (7, 1.8 g, 10 mmol) were added and the mixture was stirred at 100 °C for 10 h. The mixture was filtered through a pad of Celite, eluted with dioxane (10 mL) and the filtrate was evaporated. The obtained residue was mixed with water (25 mL), extracted with CH2Cl2 (4 x 25 mL) and the extract was dried with magnesium sulfate. The residue after evaporation (3.2 g) was crystallized from MeOH to give 0.9 g (29 %) of 4.
Method E) PyBroP (0.56 g, 1.2 mmol) was added to a solution of 11a (0.16 g, 1 mmol) in dry dioxane (8 mL) and triethylamine (0.3 g) in a sealed tube and the mixture was stirred under agron for 2h. Then 13 (0.275 g, 0.95 mmol), sodium carbonate (0.53 g, 5 mmol), PdCl2(Ph3P)2 (50 mg, 7 mol %) and water (2 mL) were added and the mixture was stirred in the sealed tube for 2 h. The mixture was stirred overnight. at room temperature and the liquid portion was diluted with EtOAc (25 mL). The insoluble portion on the walls of the sealed tube was boiled with EtOAc (2 x 25 mL), the hot solutions were decanted and combined. All the organic portions were combined, washed with water and dried with magnesium sulfate. The residue after evaporation (1.2 g) was purified by centrifugally accelerated axial chromatography (CH2Cl2 – acetone 20 : 1 to 5 : 1 v/v) to give 0.22 g (75.8 %) of 4; mp 194-197 °C.
N-(3-(3-Cyanopyrazolo[1,5-a]pyrimidin-7-yl)phenyl)-N-ethylacetamide, zaleplon (1)
Standard of zaleplon (1) used for the spectral studies was prepared according to the patent.8 Mp 183-185 °C (mp 186-187 °C)4. HRMS for C17H16N5O (M+H)+ Calcd: 306.13548, found: 306.13477. 1H NMR (500.13 MHz, CDCl3) δ, ppm: 1.17 t, 3H, J = 7.2 (CH3), 1.94 s, 3H (CH3CO), 3.83 q, 2H, J = 7.2 (NCH2), 7.22 d, 1H, J = 4.4 (H-6), 7.46 d, J = 7.9 (H-4’), 7.69 t, J = 7.9 (H-5’), 7.95 s, 1H (H-2’), 7.99 d, J = 7.9 (H-6’), 8.44 s, 1H (H-2), 8.82 d, 1H, J = 4.4 (H-5). 13C NMR (125.77 MHz, CDCl3) ) δ, ppm: 13.1 (CH3); 23.0 (CH3CO); 44.0 (CH2); 83.7 (C-3); 109.8 (C-6); 112.5 (CN); 128.7 (C-6’); 129.6 (C-2’); 130.3 (C-5’); 131.0 (C-1’); 131.6 (C-4’); 143.5 (C-3’); 146.9 (C-7); 147.1 (C-2); 151.3 (C-3a); 152.6 (C-5); 169.6 (CO). IR (KBr) cm-1: ν(CH) 3054, 2972, ν(C=N) 2228, ν(C=O) 1644, ν(C=C) + (C=N) 1613, 1547, 1486. UV λmax (log ε): 204 (4.40), 232 (4.54), 338 (3.81).
ACKNOWLEDGEMENTS
This work was supported by Zentiva Prague. The authors’ thanks are also due to Dr. Lukáš Plaček and Dr. Tomáš Pekárek for measuring and interpreting the MS (L.P.), UV and IR spectra (T. P.).
References
1. Anon., Drugs of the Future, 1996, 21, 37.
2. M. Doplet and G. L. Plosker, Drugs, 2000, 60, 413. CrossRef
3. J. K. Walsh, C. P. Pollak, M. B. Scharf, P. K. Schweitzer, and G. W. Vogel, Clin. Neuropharmacol., 2000, 23, 17. CrossRef
4. J. P. Dusza, A. S. Tomcufcik, and J. D. Albright (American Cyanamide), US 4626538 (1985) (Chem. Abstr., 1986, 105, 72777).
5. P. Thurajrajan (American Cyanamide), US 5714607 (1995) (Chem. Abstr., 1998, 128, 154095).
6. M. Korycinska, T. Stawinski, and M. Wieczorek (Adamed), WO 95456 (2003) (Chem. Abstr., 2003, 139, 381506).
7. F. Korodi, E. Fehér, and E. Magyar (TEVA Pharmaceuticals), WO 100828 (2002) (Chem. Abstr., 2002, 138, 24725.
8. S. Rádl (Zentiva), WO 37824 (2004) (Chem. Abstr., 2004, 143, 43894).
9. C. Bharathi, K. J. Prabahar, C. S. Prasad, K. M. Karavana, S. Magesh, V. K. Handa, R. Nadala, and A. Naidu, J. Pharm. Biomed. Anal., 2007, 77, 101. CrossRef
10. R. K. Robins, G. R. Revankar, D. E. O'Brien, R. H. Springer, T. A. Novinson, S. K. Antony, J. P. Miller, and D. G. Streeter, J. Heterocycl. Chem., 1985, 22, 601. CrossRef
11. S. V. Sunthankar and S. D.Vaidya, Ind. J. Chem., Sect. B: Org. Chem. Incl. Med. Chem., 1977, 15B, 349.
12. K. Nagahara, H. Kawano, S. Sasanka, C. Ukawa, T. Horana, and A. Takada, J. Heterocycl. Chem., 1994, 31, 239. CrossRef
13. B. Al-Saleh, S. H. Makhseed, M. E. Huwaida, and M. H. Elnagdi, Synthesis, 2006, 59. CrossRef
14. L. K. Gavrin, A. Lee, B. A. Provencher, W. W. Massefski, S. D. Huhn, G. M. Ciszewski, D. C. Cole, and J. C. McKew, J. Org. Chem., 2007, 72, 1043. CrossRef
15. A. Deeb, M. El-Mobayed, A. Adel El-Hamid, and A. Atef El-Hamid, Pol. J. Chem., 1992, 66, 44.
16. A. Deeb, M. El-Mobayed, A. A. Essawy, A. Adel El-Hamid, and A. Atef El-Hamid, Rev. Roum. Chim., 1993, 38, 303.
17. C. Mustazza, M. R. Del Giudice, A. Borioni, and F. Gatta, J. Heterocycl. Chem., 2001, 38, 1119. CrossRef
18. C. Jagusch, M. Negri, U. E. Hille, Q. Hu, M. Bartels, K. Jahn-Hoffmann, M. A. E. Pinto-Bazurco Mendieta, B. Rodenwaldt, U. Mueller-Vieira, D. Schmidt, T. Lauterbach, M. Recanatini, A. Cavalli, and R. W. Hartmann, Bioorg. Med. Chem., 2008, 16, 1992. CrossRef
19. K. W. Anderson and S. L. Buchwald, Angew. Chem. Int. Ed., 2005, 44, 6173. CrossRef
20. H. Cahová, L. Havran, P. Brázdilová, H. Pivoňková, R. Pohl, M. Fojta, and M. Hocek, Angew. Chem. Int. Ed., 2008, 47, 2059. CrossRef
21. P. Čapek, M. Vrábel, Z. Hasník, R. Pohl, and M. Hocek, Synthesis, 2006, 3515. CrossRef
22. S. Scherer, A. Meudt, B. Lehnemann, A. Kalinin, and V. Snieckus (Archimica), EP 1479686 (2004) (Chem. Abstr., 2004, 141, 424290).
23. S. Das, V. L. Alexeev, A. C. Sharma, S. J. Gleib, and S. A. Aster, Tetrahedron Lett., 2003, 44, 7719. CrossRef
24. G. Snatzke and J. Vlahov, Liebigs Ann. Chem., 1985, 439. CrossRef
25. J. F. Klebe, H. Finkbeiner, and D. M. White, J. Am. Chem. Soc., 1966, 88, 3390. CrossRef
26. M. Murata, T. Oyama, S. Watanabe, and Y. Mazura, J. Org. Chem., 2000, 65, 164. CrossRef
27. M. Murata, T. Sambommatsu, S. Watanabe, and Y. Masuda, Synlett, 2006, 1867. CrossRef
28. K. L. Billingsley and S. L. Buchwald, J. Org. Chem., 2008, 73, 5589. CrossRef
29. P.-E. Broutin, I. Čerňa, M. Campaniello, F. Leroux, and F. Colobert, Org. Lett., 2004, 24, 4419. CrossRef
30. Yu. A. Viets, E. V. Mutsenek, E. G. Neganova, and I. P. Beletskaya, Zh. Org. Khim., 2001, 31, 1657 (Russ. J. Org. Chem., 2001, 37, 1538).
31. E. D. Bergmann and M. Bentov, J. Org. Chem., 1955, 20, 1654. CrossRef
32. W. Seaman and J. R. Johnson, J. Amer. Chem. Soc., 1931, 53, 711. CrossRef
33. F.-A. Kang, Z. Sui, and W. V. Murray, J. Am. Chem. Soc., 2008, 130, 11300. CrossRef
34. S. Rádl, M. Blahovcová, L. Plaček, T. Pekárek, and J. Havlíček, J. Heterocycl. Chem., in preparation.