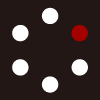
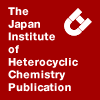
HETEROCYCLES
An International Journal for Reviews and Communications in Heterocyclic ChemistryWeb Edition ISSN: 1881-0942
Published online by The Japan Institute of Heterocyclic Chemistry
e-Journal
Full Text HTML
Received, 10th September, 2009, Accepted, 23rd October, 2009, Published online, 5th November, 2009.
DOI: 10.3987/REV-09-659
■ Utility of 2,4-Dioxoesters in the Synthesis of New Heterocycles
Kamal M. Dawood, Hassan Abdel-Gawad, Hanan A. Mohamed, and Bakr F. Abdel-Wahab*
Applied Organic Chemistry Department, National Research Center, Dokki, Giza, Egypt
Abstract
This review deals with synthesis and reactions of 2,4-dioxoesters. Some of these reactions have been applied successfully to the synthesis of biologically important compounds. The data published over the last years on the methods of synthesis and chemical properties of 2,4-dioxoesters are reviewed here for the first time.INTRODUCTION
2,4-Dioxoesters, the acylation products of methyl ketones with dialkyl oxalate, are valuable multi-purpose intermediates in organic synthesis and their preparation is well documented. 2,4-Dioxoesters are used in production e.g. pyrazole-3(5)-ethyl esters and their derivatives which are known to be important intermediates in the preparation of agrochemicals, microbicides, herbicides,1 plant growth regulators and protectants,2 and also production of 3(2H)-furanone ring system which is the key skeletal element of many natural product antitumor agents.3 Recently a review on utility of regio- and chemoselective features of benzoylpyuravtes in heterocyclic synthesis has been appeared.4 The main purpose of this review is to present a survey of the literature on the synthesis of 2,4-dioxoesters and its reactions and provides useful and up-to-date data for medicinal chemists.
METHODS OF SYNTHESIS
2,4-Dioxoesters 1 were prepared by Claisen condensation of the appropriate methylketone with oxalic acid dialkyl esters in the presence of sodium alkoxides (Scheme 1) then acidified with dilute acid to give an excellent yields of the precursors 1.5-21
Cyclic ketones 2 were condensed with dimethyl or diethyl oxalate to give the diketoester 3 which present in two tautomeric structures (Scheme 2).22-26
CHEMICAL PROPERTIES OF 2,4-DIOXOESTERS
2,4-Diketoalkanoates have both the structural features of α-keto esters and β-diketones (Figure 1). In the case of α-keto esters 4, the adjacent carboxyl moiety imparts the ketone with an enhanced electrophilic character due to its inductive withdrawal. However, this may be moderated by the presence of active protons due to keto/enol tautomerisation between 5 and 6. In the case of β-diketones 4, sharing an active methylene group enables both carbonyl functionalities to undergo keto/enol tautomery forming a Michael acceptor.4
1. HYDROLYSIS
The β-diketo esters are converted to the corresponding pyruvic acids 8 (Scheme 3).8, 27
2. REDUCTION
The hydrogenation of 2,4-dioxovalerates 9, in the presence of chiral rhodium or ruthenium catalysts provides direct access to 3-hydroxy-5-subs.tetrahydrofuran-2-one with syn:anti ratios of up to 84:16 and with up to 98% and 94% ee in the syn and anti form 10 and 11 respectively (Scheme 4).28, 29
The derivatives of 4-substituted 2-hydroxybutyric acids are valuable synthons for the production of antihypertensive substances, homoamino acids, hydroxamic acids, and other compounds.30,31 Thus hydrogenation of ethyl 4-substituted 2,4-dioxobutyrate 12 at palladium black and Pt/Al2O3 were studied. During the hydrogenation of compounds 12 at palladium black at room temperature with a hydrogen pressure of 1 atm in ethanol solution, the first reaction products are ethyl 2-hydroxy-4-oxo-4-subs.-butyrate 13, which then reduced with Pd/C to give ethyl 2-hydroxy-4-phenylbutanoate 14 (Scheme 5).32
Ethyl 2,4-dioxo-4-phenylbutyrate was converted to 3-oxo-3-phenyl-1-propanol 15 in 90% yield by reaction with baker's yeast. Reductive amination with sodium cyanoborohydride in the presence of ammonium acetate gave the racemic 3-amino-3-phenyl-1-propanol 16 in 65% yield. Enzymic resolution of the corresponding N-phenylacetyl derivative with penicillin G acylase, immobilized on an epoxy resin gave (S)-amide 18 and (R)-amino 19 alcohols in high enantiomeric purity (ee >99%) and >45% yields for each enantiomer in addition to phenylacetic acid as side product, while reduction of 15 with sodium borohydride gave 1,3-diols 20 (Scheme 6).33
Ethyl 2,4-dioxo-4-phenylbutyrate, was reduced enantio- and regiospecifically by baker's yeast in a diisopropyl ether/water two-phase system to give (-)-ethyl (R)-2-hydroxy-4-oxo-4-phenylbutyrate 21 with an 98% ee in 80% isolated yield. This (hydroxy)keto ester 21 was hydrogenated over Pd-C to obtain (-)-ethyl (R)-2-hydroxy-4-phenylbutyrate (HPB ester) 22, an important intermediate for the synthesis of ACE inhibitors. Prolonged contact of the reduction product with baker's yeast produced 3-phenyl-3-oxopropanol 23 in 90% yield (Scheme 7).8
2,4-Dioxoalkanoates 24 and the parent compound γ-keto-α-enamino esters 25 are regioselectively reduced by baker’s yeast to α-hydroxy-γ-keto esters 26, in moderate to good yields (Scheme 8).34
Ethyl 2,4-dioxo-4-phenylbutanoate was hydrogenated in the presence of (+)-dihydrocinchonidine and the O-acetylated product underwent hydrolysis in the presence of lipase PS to give (R)-ethyl 2-acetoxy-4-oxo-4-phenylbutanoate of 99.6% ee 27 and (S)-2-hydroxy-4-oxo-4-phenylbutanoic acid of 99.4% ee 28 (Scheme 9).35
Ethyl 2,4-dioxoalkanoates 29 react chemoselectively with pyrrolidine acetate at the more electrophilic C-2 carbonyl, producing enaminone esters 31. Reduction of 31 with sodium cyanoborohydride followed by pyrrolidine elimination gave β-oxo-acrylates 3236 (Scheme 10).
3. DECARBONYLATION
The α,γ-diketoesters 33 are subjected to decarbonylation conditions with excess methanol in a sealed reactor at a lower temperature (105-120 °C) to give carbon monoxide and ketenes 34 (Scheme 11).37
4. ALKYLATION
Methylation of ethyl acetylpyruvate with diazomethane gave a mixture of two enol ethers, ethyl 2-methoxy-4-oxopent-2-enoate 35 and ethyl 4-methyl-2,5-dioxohex-3-enoate 36 in 68:77 to 23:32 ratios depending on the temperature (Scheme 12).38,39
REACTIONS OF 2,4-DIOXO-ESTERS
1. FORMATION OF BENZENE DERIVATIVES
3-Hydroxy-5-methylbenzoic acid 37, which used in the synthesis of thrombin inhibitor, was prepared in high yield from ethyl 2,4-dioxopentanoate in two reaction steps by the reaction with acetic acid followed by treatment with magnesium oxide (Scheme 13).40
The pyridones 38 reacted with sodium salt of ethyl acetopyruvate in pyridine at 70 °C to give 45.0-98.1% phenols 39 and 10.9-84.2% nitroacetamide 4041 (Scheme 14).
2. FORMATION OF HETEROCYCLES
2.1. FIVE MEMBERED SYSTEMS
2.1.1. 2,4-PYRROLIDINEDIONES
The condensation of α,γ-diketoesters 41 with aromatic amine and aromatic aldehydes was a convenient method for the synthesis of 4-acyl-2,3-pyrrolidinediones 42 (Scheme 15).14,42-47
The condensation of α,γ-diketoesters 43 with aldimines 44 derived from o-hydroxybenzaldehydes provides a route to the pyrrolo[3,2-c]benzopyran ring system 46, via cyclization of the intermediate pyrrolidinedione 45 which was not isolated (Scheme 16).45
Some substitution products of 2,3-pyrrolidinones 47 and 48 are obtained by condensing an easily saponifiable derivative of a hydroxybenzaldehyde or a hydroxybenzaldehyde alkylcarbonic ester with primary amines and α,γ-diketoesters.48 The products 47 may be treated then to remove the saponifiable group (Scheme 17). It has been found that these products are useful as drugs or intermediates for drugs.
The condensation of α,γ-diketoesters with Schiff bases, was further extended to the use of ketimines, in such case, the spirocyclic system 49 was obtained via the formation of 50 (Scheme 18).45
Reaction of 2-oxocycloalkylglyoxylate esters 50-52 with N-phenylmethyleneaniline yields 2-aza-3,4,6-trioxo-1,2-diphenylspiroalkanes 53-55 (Scheme 19).49
2.1.2. PYRROLES
3-Acetylpyrrole-2-carboxylic acid 56 was prepared by treatment of ethyl 2,4-dioxovalerate with aminoacetaldehyde in presence of 20% aqueous sodium hydroxide, followed by acidification (Scheme 20).50
Ethyl 2-(hydroxyimino)acetate and ethyl 2,4-dioxopentanoate were reacted with at 45 °C diethyl 5-methyl-2,3-pyrroledicarboxylate 57 and ethyl 5-ethoxycarbonyl-2,4-dimethyl-3-pyrroleglyoxylate 58 (Scheme 21).51
Low-valent rhodium complexes are efficient catalysts for the activation of α-C-H bond of isonitriles. Catalytic synthesis of pyrrole 59, in 76% yield, can be performed by cyclocondensation of ethyl 2-cyanoacetate with ethyl 2,4-dioxopentanoate (Scheme 22).52
Pyrrole chalcone 61 was prepared in by piperidineacetate-catalyzed condensation of 2,5-dimethyl-1-phenyl-1H-pyrrole-3,4-dicarbaldehyde 60 with ethyl acetylpyruvate (Scheme 23).53
2.1.3. PYRAZOLES
Hydrazine and its monosubstituted derivatives react smoothly with 2,4-dioxoesters in a highly chemoselective manner to afford 3,5-difunctionalized pyrazoles as only one product.54–59 Thus, 1-alkyl-5-pyrazolecarboxylic acid esters 64, which are intermediates for pyrazolecarboxamide derivatives useful as drugs and agrochemicals, are in high yields prepared by mixing alkylhydrazine 63 with acylpyruvic acid 62 (Scheme 24).60-65
Ethyl 3-ethyl-5-pyrazolecarboxylate 65, which is an intermediate for the acaricide tebufenpyrad, was synthesized at a yield of more than 87% with ethyl propionylpyruvate and hydrazine hydrate or dihydrazine sulfate (Scheme 25).66-69
Ethyl propionylpyruvate was reacted with methylhydrazine with the molar ratio above 1: 1.2 to give 95% of ethyl 3-ethyl-1-methyl-1H-pyrazole-5-carboxylate 66 (Scheme 26).70-72
Ethyl 2,4-dioxopentanoate was cyclocondensed with methylhydrazine and the product 67 was saponified to give 1,5-dimethylpyrazole-3-carboxylic acid 68 which were amidated by 2,6-dimethylbenzenamine to give title compound 69 (Scheme 27). The latter compound gave complete protection against electroshock-induced convulsions to mice at 15 mg/kg i.v.73,74
4-Bromo-2-(hydrazinylmethyl)phenol treated with ethyl 2,4-dioxopentanoate to yield predominantly the desired regioisomer 70 which was conveniently purified by preferential crystallization from the reaction mixture upon cooling (Scheme 28).75
The synthesis of 1,5-diarylpyrazoles 72 was done (Scheme 29) by regioselective cyclization of arylhydrazines 71 with ethyl 2,4-dioxo-4-phenylbutanoate in refluxing ethanol. Esters 72 were reduced with LiBH4 (anhydrous THF, argon), yielding the primary alcohols 73. Bromination of the crude products with (C6H5)3P-NBS gave the pyrazoles 74. The alkyl bromides 74 were reacted with diverse phenols in basic medium to give 3-Phenoxymethylpyrazoles 75 which are useful in prostate cancer chemotherapy.76
The diarylpyrazole backbone 76 was prepared from ethyl 2,4-dioxo-4-phenylbutanoate and the corresponding 4-methylsulfonylphenylhydrazine and acetic acid in refluxing ethanol. Compound 76 was reduced in the presence of LiAlH4 in anhydrous THF yielding the 1-(4-methylsulfonylphenyl)-5-phenyl-1H-pyrazole-3-methanol 77. The alcohol 77 was then mesylated in the presence of triethylamine in dichloromethane and reacted with 3-fluoro-5-(4-methoxytetrahydro-2H-pyran-4-yl)phenol and Cs2CO3 in DMF at 80 °C to yield 3-{[(3-fluoro-5-(4-methoxytetrahydro-2H-pyran-4-yl)phenoxy)methoxy]methyl}-1-(4-(methylsulfonyl)phenyl)-5-phenyl-1H-pyrazole 78 (Scheme 30) which used as dual cyclooxygenase-2/5-lipoxygenase inhibitor.77
Synthesis of ethyl 1-(2`-hydroxy-3`-aroxypropyl)-3-aryl-1H-pyrazole-5-carboxylate 81 is outlined in Scheme 31. Starting compounds, ethyl 3-aryl-1H-pyrazole-5-carboxylate and hydrazine. The reaction of ethyl 3-aryl-1H-pyrazole-5-carboxylate 79 with 2-aryloxymethylepoxide 80 in the presence of potassium carbonate at refluxing in acetonitrile afforded ethyl 1-(2`-hydroxy-3`-aroxypropyl)-3-aryl-1H-pyrazole-5-carboxylate 81 in moderate yields and completely regioselectivity.7
1-(3-Methoxyphenyl)-5-phenyl-1H-pyrazole-3-carboxylic acid ethyl ester 82 was prepared in 36% yield by the reaction of ethyl 4-phenyl-2,4-dioxobutanoate with 3-methoxyphenylhydrazine hydrochloride in dry ethanol under a nitrogen atmosphere and in the presence of equivalent of triethylamine at refluxing temperature (Scheme 32).78
Cyclocondensation of 1-(phthalazin-1-yl)hydrazine-HCl and substituted ethyl cinnamoylpyruvates 83 gave 1-(1-phthalazinyl)-3-carbethoxy-5-(3- or 4-substituted styryl)pyrazoles 8479 (Scheme 33).
Ethyl (2-furoyl)pyruvate condensed with hydrazine to give bis(furylpyrazole)hydrazide 85. While condensing with formaldehyde and phenols and hydrazine gave 86 (R = CH2R1)80 (Scheme 34).
Cyclization of ethyl 2-thenoylpyruvate with hydrazines in acetic acid gave pyrazole derivative 87 which reacted with hydrazine in refluxing EtOH/AcOH to give hydrazides 88 in high yields. Reaction of 88 with aldehydes and formic acid gave 89 and pyrazolotriazinone 90, respectively in good yields (Scheme 35).17,81
While reaction of ethyl 2-thienoylpyruvate with hydrazine hydrate in neutral or basic medium led to the decomposition to 2-acetylthiophene and oxalohydrazide 92 (Scheme 36).17
Recently, Abdel-Wahab et al. reported the reaction of ethyl 2-benzofuroylpyruvate with phenylhydrazine in glacial acetic acid to give the pyrazole-3-carboxylate 93 which then reacted with hydrazine hydrate to give the corresponding carbohydrazide 94, Reactivity of the latter hydrazide towards aromatic aldehydes and phathalic anhydride were studied to give the corresponding hydrazones 95 and imides 96 (Scheme 37).15,82,83
When 5-hydrazinylmethyl-3-methyl-1-(3-methyl-1H-pyrazol-5-yl)methyl)-1H-pyrazole 97 was treated with ethyl 2,4-dioxopentanoate in ethanol gave a mixture of two tris-pyrazoles 98 and 99 in 10% and 25% yields respectively (Scheme 38).84
When ethyl 2,4-dioxo-4-(pyridin-3-yl)butanoate was treated with methoxyamine hydrochloride gave ethyl 2-methoxyimino-4-oxo-4-(pyridin-3-yl)butanoate 100, which then reacted with 3-hydrazinylbenzonitrile to give a mixture of two isomers 101 and 102 in a percentage 1:9 respectively (Scheme 39).65
Treatment of pyruvate 103 with dinitrogen trioxide (generated from sodium nitrite and HCl) in ethanol gave the oxime 104. Cyclization of 104 with methyl hydrazine gave a mixture of the nitroso pyrazoles 105 and 106, which were separated by flash chromatography. Reduction of the nitroso group with sodium dithionite in aqueous THF gave the respective aminopyrazoles 107 and 108 (Scheme 40).85
Ethyl 3-methyl-1-phenyl-1H-5-carboxypyrazoles 109 were prepared regioselectively from a condensation of the appropriate phenylhydrazine and ethyl 2-(N-methoxyimino)-4-oxopentanoate.86,87 Coupling of ester 109 with the known amines 110 gave good yields of the amides 111. Deprotection of the tert-butylsulfonamide moiety, by treatment with trifluoroacetic acid, afforded compounds 112. Treatment of the methoxy-substituted precursor compounds 112 with excess boron tribromide afforded the phenolic analogues 113 (Scheme 41), which is useful as non-amidine factor Xa inhibitors.88,89
The pyrazole derivatives were synthesized from para or meta nitrobenzaldehydes which was refluxed with triethyl orthoformate to give the enol ether 114. Cyclization of 114 with hydrazine afforded 115 that were then linked to trityl resin through one of the pyrazole nitrogens. The hydrolysis of the ester 116 to 117 by alkali (Scheme 42).90
C-Alkylation of α,γ,-diketoesters with 6-chloropiperonyl chloride were performed in the presence of EtONa and NaI in DMF. The crude compounds 118 were allowed to react directly with hydrazine monohydrate in ethanol to afford the desired pyrazole esters 119. Regioselective alkylation of 119 with the requisite alkyl halide or alkyl tosylate in the presence of NaH in DMF at room temperature gave compounds 120. Finally, the pyrazole acids 121 (Scheme 43) were obtained after saponification in good yields which useful as potent nonpeptide endothelin antagonists.6
The target compounds 123, 124 starts from 2,4-dioxopentanoic acid ethyl ester 122 (R1 = Me) or the 2,4-dioxo-4-phenyl-butyric acid ethyl ester 122 (R1 = Ph). These β-diketo compounds undergo reactions with methyl and phenyl hydrazine, yielding a 2:1 to 1:2 mixture of the two isomeric disubstituted pyrazole-3-carboxylic acid ethyl esters 123 and 124, respectively (Scheme 44).91,92
The reaction of the ethyl 4-(furan-2-yl)-2,4-dioxobutanoate with methylhydrazine in ethanol at room temperature afforded an almost 1:1 mixture of the two 5-furyl 125 and 3-furyl 126 regioisomers in high yield; these were then easily separated with the aid of flash chromatography. In contrast to the lack of regioselectivity observed in EtOH, when the condensation reaction was carried out with the fluorinated solvents TFE and HFPI, the ratio increased to 93:7 in favor of the desired regioisomer 125, which was obtained in almost quantitative yield. 3-(Ethoxycarbonyl)-5-(2-furyl)-N-methylpyrazole 125 was then converted into the aldehyde 128, obtained in 75% combined yield through a two-step sequence involving LiAlH4 reduction to the corresponding alcohol 128 and subsequent oxidization with MnO2, Compound 127 was then used as the key intermediate for the preparation of the rest of the fluorinated analogs of tebufenpyrad (Scheme 45). Reaction of 128 with MeMgBr afforded the 1-hydroxyethyl derivative 129 in 94% yield. Subsequent treatment of 129 with deoxofluor provided the monofluorinated derivative 130 in 61% yield.93,94
GSK183390A 134 has recently emerged as a potent dual agonist of PPARα/γ and a candidate for treatment of dyslipidemia, the synthesis of 2-{4-[(5-(4-tert-butylphenyl)-1-methyl-1H-pyrazole-3-carboxamido]methyl]-2-methylphenoxy}-2-methylpropanoic acid 134 is depicted in Scheme 46 via coupling of benzylamine and pyrazole. The condensation between methylhydrazine and diketoester produced a mixture of 1,3,5-trisubstituted pyrazole 131 and its isomer 132. Coupling of 131 with benzylamine derivatives in the presence gave the target drug 134 in 73% yield.16
Solventless condensation of ethyl 2,4-dioxopentanoate and a hydrazine in the presence of a catalytic amount of sulfuric acid at room temperature afforded pyrazole derivatives 135 in high yield, while its reaction with phenylhydrazine under the same conditions gave the equal percentage of two pyrazole isomers 136 and 137 (Scheme 47).95
Compound 136 was prepared from commercially available 5-methyl-1H-pyrazol-3-amine by diazotation in HCl followed by reduction with tin chloride and the intermidiate diamine is not isolated but has immediately undergone a condensation with the β-diketones to give the ester 136 in a 36% yield. The methylation of this product in the presence of t-BuOK as base led to one isolated α-isomer 137 in a 29%, Finally, the compound 137 was convert to the target product 138 using LiAlH4 as agent of reduction (Scheme 48).96
Reaction of methyl 5,5-dimethyl-3-dimethylaminomethylene-2,4-dioxohexanoate 139 with phenylhydrazine afforded methyl 1-phenyl-4-pivaloyl-1H-pyrazole-5-carboxylate 140 which was converted to 1-phenyl-4-pivaloyl-1H-pyrazole 141 by basic hydrolysis followed by loss of carbon dioxide (Scheme 49).97
Reaction of ethyl 4-(1H-indol-3-yl)-2,4-dioxobutanoate98,99 with phenylhydrazine in refluxing acetic acid gave the pyrazole 142 (Scheme 50).99
Condensed pyrazoles 146 which exhibit antiproliferative activity were prepared by treating 143 with arylhydrazines, followed by ester hydrolysis and amination of 145 to give the target molecules (Scheme 51).21-25
The 1H-pyrazolo-[3,4-d]-pyridazin-7(6H)-one core analog 149 was prepared. Therefore, 1-(1-bromo-2,2,2-trifluoroethylidene)-2-(4-methoxyphenyl)hydrazine was treated with ethyl 2,4-dioxovalerate in the presence of ethanolic sodium ethoxide to afford a good yield of a separable 1:1 mixture of pyrazole regioisomers 147 and 148. The desired 148 was smoothly condensed with 4-bromophenylhydrazine in refluxing ethanol to give the 1H-pyrazolo[3,4-d]pyridazin-7(6H)-one core 149 (Scheme 52), which crystallized out of solution upon cooling.100
Aminoguanidine was treated with ethyl acetopyruvate at 100 °C and pH 1 to give pyrazole 150, whereas the reaction at pH 4 gave triazine 151. While at room temperature and at pH 6-7 gave the dimer 152 that was hydrolyzed by dil. HCl to give 151 (Scheme 53).101
Nitroaminoguanidine was treated with ethyl acetopyruvate at pH 1-7 to give 153 and pyrazole-5-ester 154 (Scheme 54).101
Dihydralazine 155 reacted with ethyl 2,4-dioxoalkanoates to give dipyrazolylphthalazines 156 (Scheme 55).102
2.1.4. ISOXAZOLES
Diketoester 157 was converted to isoxazole ester 158 upon reaction with hydroxylamine hydrochloride in refluxing ethanol. Isoxazole ester 158 was reduced to the corresponding alcohol 159 using lithium aluminum hydride in THF at -78 °C. Alcohol 159 was treated with triphenylphosphine dibromide to furnish bromide 160, which was converted to isoxazole azide 161 by reaction with sodium azide in acetone, azide 161 can be converted to the corresponding amine 162 by using 1,3-propanedithiol reduced organic azides selectively to amines, itself being oxidized to the cyclic disulfide (Scheme 56).10
The reaction of 2,4-diketo esters 163 with hydroxylamine hydrochloride gave 3-isoxazole esters 164, in excellent yield, these ester were reduced with lithium aluminum hydride and the resulting alcohols 165 were oxidized with pyridinium chlorochromate (PPC) to yield substituted 3-isoxazolecarbaldehydes 166. These aldehydes were then treated with various activated alkenes in the presence of DABCO in the absence of any solvent to furnish the Baylis-Hillman adducts 167 in excellent yields, also, the isoxazolecarboaldehydes undergo fast reaction with cyclohexenone in the presence of DMAP to give 168 (Scheme 57).103
Ethyl 2-furoylpyruvate was reacted with hydroxylamine hydrochloride in the presence of sodium carbonate to afford ethyl 5-(2-furyl)-3-isoxazolecarboxylate 169 which then hydrolyzed with HCl-H2O to give 5-(2-furyl)-3-isoxazolecarboxylic acid 170 (Scheme 58).14
Ethyl 2-benzofuroylpyruvate on treatment with hydroxylamine hydrochloride in water give the dioxime 171, while in acetic acid afforded ethyl 5-(2-benzofuryl)-3-isoxazolecarboxylate 172 (Scheme 59).15
Reaction of with hydroxylamine hydrochloride in the presence of anhydrous potassium carbonate to give the corresponding oxime 173 while in pyridine gave ethyl isoxazole-3-carboxylate 174 in good yields (Scheme 60).18
2.1.5. TRIAZOLES
The reactivity of benzolpyruvates as β-diketo moiety towards 1,3-dipoles was studied. Thus, when treated with an organic azide 175, benzoylpyruvates may react to form cycloadducts, like 1,2,3-triazole 176 (Scheme 61).104
2.1.6. FURANONES
Ethyl 2,4-dioxoalkanoates, are used in the synthesis of 3(2H)-furanone ring system, the key skeletal element of many natural product antitumor agents. The first method consisted in the reaction of the 2,4-dioxoalkanoates with hydroxylamine hydrochloride in ethanol to form in good yield the corresponding 3,5-disubstituted isoxazoles 177.105 Isoxazoles have long been regarded as a protected form of l,3-diketones, from which are commonly prepared, by virtue of its catalytic or chemical reduction to β-enamino-ketones.106 Primary alcohols 178 were obtained in essentially quantitative yield by action of sodium borohydride on 177 in methanol, while secondary alcohols 182 were derived in a two-step sequence, namely reaction with methyl magnesium iodide in the presence of triethylamine to give the ketone 181 followed by reduction with sodium borohydride. Tertiary alcohols 185 were directly obtained by treatment of 177 with an excess of Grignard reagent in more than 80% yield. On exposure of all the isoxazole alcohols to hydrogen and PtO2/Ni-Raney mixture of catalysts in methanol a rapid reaction ensued to give the corresponding β-enaminoketones having a primary 179, 183 or tertiary 186 γ-hydroxy group. All these vinylogous amides were cleanly transformed to 3(2H)-furanones 180, 184 and 187 respectively by treatment at room temperature in AcOH:H2O 2:l mixture in good yields (Scheme 62).3
2.2. SIX-MEMBERED SYSTEMS
2.2.1. PYRIDINE DERIVATIVES
Ethyl 2,4-dioxopentanoate was condensed with 2-cyanoacetamide in the presence of diethyl amine to give ethyl 3-cyano-2-hydroxy-6-methylpyridine-4-carboxylate 188 which on nitration with nitric acid in acetic anhydride give the corresponding 5-nitro derivatives 189, halogenation followed with reduction of the latter compound gave ethyl 3-amino-6-chloro-5-cyano-2-methylpyridine-4-carboxylate 191 (Scheme 63).107
Ethyl 2-hydroxy-4-oxopent-2-enoate was condensed with 2-cyanoacetamide in H2O/ EtOH in piperdine to give ethyl 2-methyl-5-cyano-6(1H)-pyridone-4-carboxylate the tautomeric form of 192 which then treated with a mixture of PCl5 and POCl3 to give ethyl 2-methyl-5-cyano-6-chloro-4-pyridinecarboxylate 193, Reduction of the latter compound followed by cyclization afforded 2-methyl-5-aminomethyl-4-pyridinecarboxylic acid lactam 194 in 41.7% (Scheme 64).108
Oka et al. reported the synthesis of diethyl 2-methyl-6-(naphthalen-2-yl)pyridine-3,4-dicarboxylate 196 by reaction of ethyl 4-(naphthalen-2-yl)-2,4-dioxobutanoate 195 with ethyl 3-aminobut-2-enoate (Scheme 65).109
For the Hantzsch pyridine synthesis, methyl acetopyruvate was reacted with methyl 3-aminocrotonate and 3-nitrobenzaldehyde in isopropanol to give a mixture of two isomers 197 and 198 in 26.7 % and 9.2% yields, which separeted by column chromatogrphy. Reduction of 197 with sodium borohydride in alcohol led to lactone 199 (Scheme 66).110
2.2.2. PYRIMIDIMES
Benzoylpyruvates can be reacted as β-diketones111–114 with N,N`-dinucleophiles such as amidines 200a, isoureas 200b, guanidines 200c (R =NH2, NH-alkyl, NH-aryl etc.) and ureas 201 (R = O or S), Resulting in the formation of an aromatic or latent aromatic system, the reaction should accordingly be highly favored, yielding 2,4,6-trifunctionalized pyrimidines 202 or derivatives thereof (Scheme 67).
The synthesis of pyrimidin-2-ones like 204 has been reported. Thus, the presence of base has been used to promote condensation between a benzoylpyruvate 203 and urea (Scheme 68).115
In a similar fashion, condensation between a benzoylpyruvate 205 and guanidine gives an entry to the imprinted 2-aminopyrimidine 206 (Scheme 69).116
2.2.3. PIPERAZINES
Benzoylpyruvate can react across α-keto ester moiety with N,N`-dinucleophiles. Reactions involving ethylene diamines 207 or α-amino acrylamides 209 lead to piperazine-2-one derivatives like 208 and 210 (Scheme 70).117–120
2.2.4. 1,2,4-TRIAZINES
Condensation of ethyl 2,4-dioxobutyrates with imidio-hydrazides 211 or S-methylisothiosemicarbazide hydroiodide in pyridine gave the corresponding 1,2,4-triazin-5(2H)-ones 212 and 213 in 50-80% (Scheme 71).20,120-122
Similarly, reaction with semicarbazides 214 and aminoamidine 215 lead to 1,2,4-triazine-5-one derivatives like 216 and 217 (Scheme 72).117-120
2.2.5. PYRONE DERIVATIVES
2-Pyrone derivatives 219 were prepared in a one step procedure from (chlorocarbonyl)phenylketene 218 and ethyl 2,4-dioxopentanoate (Scheme 73).123
Thus the cycloaddition mechanism represented in Scheme 74 accomplished by mixing the equimolar quantities of (chlorocarbonyl)phenyl ketene 218 and 1,3-diketones at ambient temperature in dry ether. 1,3-Diketones exist mainly in the enol forms, therefore, the OH group of the enol form will attack the acyl chloride of the ketene followed by ring closure of 220 to produce the product 219.123
The reaction between malononitrile and benzoylpyruvate 222 gave 3,4,6-functionalized pyran-2-one 223 (Scheme 75).115 The incipient enolate, formed by initial conjugate addition, attacks in turn one of the electrophilic nitriles to render a 3,4,6-functionalized pyran-2-one 223.
Benzoylpyruvates may themselves serve as cyclic precursors in the absence of external nucleophiles. With heteroatoms located at the 2-position of the aryl moiety, as in the case of 224, it is possible to obtain fusion by intramolecular cyclodehydration across the a-keto group. This approach has been used to prepare chromone esters like 225 (Scheme 76).4
2.2.6. THIADIAZINE DERIVATIVES
The synthesis of 2-substituted 1,2,6-thiadiazine-5-carboxylates were carried out with ethyl 2,4-dioxovalerate 228. Thus, the reaction of 227 with sulfamides 226 in ethanol or diglyme afforded the corresponding 3-methyl-1,2,6-thiadiazine-3-carboxylates 228.The preparation of the amides 229 was carried by reaction of 228 with amines124 (Scheme 77).
2.3. FUSED HETEROCYCLIC SYSTEMS
2.3.1. BENZIMIDAZOLE DERIVATIVES
The synthetic pathway to 1-aryl-3-(1H-benzimidazol-2-yl)-3-hydroxypropenone 232 are depicted in Scheme 1. The pyruvates 230 were converted by basic hydrolysis into the corresponding acids 231 which were subsequently condensed with 1,2-phenylenediamine hydrochloride to give the target compounds in microwave oven and conventional heating (Scheme 78).19
Condensation of ethyl 2-thionyl pyruvate with o-phenylenediamine in glacial acetic acid under reflux, gave the corresponding 1-(1H-benzimidazole-2-yl)-3-thiophen-2-yl)-propane-1,3-dione 233. Pyrrolo[1,2-a]benzimidazole derivatives 234 and 235 were prepared via cyclocondensation of compound 233 with acetic anhydride in presence of sodium acetate and phosphorus oxychloride respectively (Scheme 79).125
2.3.2. PYRROLO[2,3-c]PYRIDINE
Ethyl 6,7-dihydro-2,6-dimethyl-1H-pyrrolo[2,3-c]pyridine-3-carboxylate 236 was obtained similarly by treatment of ethyl 2,4-dioxopentanoate with 3-ethoxycarbonyl-1-methyl-4-piperidone (Scheme 80).51
2.3.3. PYRAZOLO[3,4-c]PYRIDINES
Pyrazolo[3,4-c]pyridines 241 were prepared by catalytic hydrogenation of 240 followed by cyclization. 240 were obtained (Scheme 81) by treating ω-bromoacetophenone oxime with sodium salt of acylpyruvate 237 and then hydrazine (yield 70-75%).126
2.3.4. PYRAZOLOPYRIMIDINES
Reaction between benzoylpyruvates and five-membered aza-heterocyclic amines may offer an entry to a variety of [a]-fused pyrimidines, in consonance with the regiochemical supposition. An illustration of this strategy is cyclodehydration involving 3-aminopyrazole 242, which affords only pyrazolo[1,5-a]pyrimidine 243 (Scheme 82).127,128
Cyclocondensation of 3-amino-3-pyrazolin-5-one with ethyl acylpyruvate gave chiefly pyrazolo[3,4-b]pyridinones 244 (Scheme 83) and smaller amount of pyrazolo[2,3-a]pyrimidin-2-(1H)-one 245 up to 30%.129
2.3.5. PYRAZOLO[1,5-d]TRIAZINES
Diaminoguanidine was treated with ethyl 2,4-dioxopentanoate in either acid of neutral aqueous solution to give pyrazolo[1,5-d]triazine 246 (Scheme 84).101
2.3.6. IMIDAZO[1,5-b]PYRIDAZINES
Ethyl or methyl acylpyruvates 247 were condensed with ω-bromo-acetophenonesemicarbazone in sodium ethoxide to give semicarbazone derivatives 248, which underwent acid catalyzed intramolecular cyclization to afford the corresponding imidazo[1,5-b]pyridazinediones 249, methylation of the latter with diazomethane afforded the N-methyl derivatives 250 (Scheme 85).130
2.3.7. ISOXAZOLO[3,4-d]PYRIDAZINE DERIVATIVES
Ethyl 4-ethyloxoacetate-5-methylisoxazole-3-carboxylate 251, was prepared by reaction of ethyl 2,4-dioxopentanoate with ethyl chloro(hydroximino)acetate in ethoxide solution. Reaction of 251 with hydrazine hydrate in ethanol gave ethyl {(6,7-dihydro-3-methyl-7-oxoisoxazole[3,4-d]pyridazinyl}-4-carboxylate 252. Treatment of the latter with 1-(3-bromopropyl)-4-(3-chlorophenyl)piperazine in anhydrous DMF containing anhydrous K2CO3 give Ethyl 6-{[4-(3-chlorophenyl)piperazin-1-yl]propyl}-3-methylisoxazolo[3,4-d]pyridazin-7-(6H)-one-4-carboxylate 253 which is useful as potent antinociceptive agent.131
2.3.8. QUINOLINE
Treatment of cyclohexane-1,3-dione with ethyl 4-(4-chlorophenyl)-2,4-dioxobutanoate led to 5,6,7,8-tetrahydro-5-oxoquinoline-4-carboxylate derivative 254 (Scheme 87).132
2.3.9. QUINOXALINE DERIVATIVES
2-Chloro-3-(2'-thenoylmethyl)quinoxaline 256 was prepared by the reaction of 1,2-benzenediamine and 2-thienoyl pyruvate, followed by treatment with phosphorus oxychloride (Scheme 88).133
3-Functionalized 1H-quinoxaline-2-ones 257 can be made by reacting benzoylpyruvates with N-phenylbenzene-1,2-diamine (Scheme 89).79,134–137
Reaction of 3-hydrazino-2(1H)-quinoxalinone 258 with ethyl aroyl pyruvates 259 afforded the corresponding hydrazones 260 which upon thermolysis at 230 °C eliminated a molecule of ethanol to give triazinoquinoxaline 261 (Scheme 90).138
3-(2-Oxo-2-phenylethylidene)-3,4-dihydro-1H-quinoxalin-2-one 262 was prepared in 38% yield by solid state synthesis. Thus, when ethyl benzoylpyruvate and o-phenylenediamine were mixed and stirred at room temperature gave the title compound 262. In the case of the condensation of ethyl benzoylpyruvate with o-aminophenol at room temperature, the solution-phase reaction afforded just product 3-(2-oxo-2-arylethylidene)-3,4-dihydro-benzo[1,4]oxazin-2-ones 262 in 31% yield. When sulfamic acid (SA) was used as catalyst in concentration 10% the yield of 262 raised 81% while 262 to 73% without using any solvent Also, the yield of other derivatives increased (Scheme 91).139
Cyclic N,O-dinucleophiles appended by a two-unit linker are restricted to 2-aminophenols. Thus, when a benzoylpyruvate reacts with 2-aminophenol 263 itself, the result is formation of the corresponding 1,4-benzoxazine-2-one 264 (Scheme 92).140-142
The Beirut reaction of benzoyl pyruvate with 1,3-benzoxadiazole 1-oxide and ethyl 2,4-dioxo-4-phenylbutyrate in ethanol or acetonitrile, catalyzed by triethylamine, gave first ethyl 3-benzoylquinoxaline-2-carboxylate 1,4-dioxide 266 and then, in a slower reaction, 2-benzoylquinoxaline 1,4-dioxide 269.143
Pathways for the formation of the dioxides 266 and 269 are suggested in Scheme 93. The intermediate 265 is of the type proposed previously for the Beirut reaction of 1,3-benzoxadiazole 1-oxide with carbonyl compound cyclization and dehydration, path a, leads to the ester dioxide 266. Nucleophilic attack on compound 265 with concomitant loss of carbon dioxide, path b, yields, after protonation of intermediate 267 the aldehyde 268, Subsequent cyclodehydration produces the dioxide 269.143
2.3.10. PYRIDO[2,3-d]PYRIMIDINE
Condensation of ethyl benzoylpyurvate and 4-aminouracil in refluxing acetic acid afforded ethyl 2,4-dioxo-7-phenyl-1,2,3,4-tetrahydropyrido[2,3-d]pyrimidine-5-carboxylate 270 as shown in Scheme 94. Basic hydrolysis and coupling with (R,S)-1-phenylpropylamine provided compound 271.144
2.3.11. PYRIDO[2,3-b]PYRAZINES
Compound 276 was prepared as shown in Scheme 95. Thus, Guareschi condensation of nitroacetamidine with ethyl 2,4-dioxo-4-phenylbutanoate in refluxing ethanol resulted in high yields of ethyl 2-amino-3-
nitro-6-phenylpyridine-4-carboxylate 272, which was transformed into the diamine 273 by catalytic hydrogenation of the nitro function. The six-membered fused pyrazine ring was incorporated by condensation of 274 with a masked dialdehyde, the 2,3-dihydroxy-1,4-dioxane, producing ethyl 6-phenylpyrido[2,3-b]pyrazine-8-carboxylate 275.145 Subsequent hydrolysis of the ester functions under basic conditions and coupling reaction with (S)-(-)-1-phenylpropylamine afforded the desired secondary amide 276.144
2.3.12. PTERIDINE
In some cases, the ethene bridged diamines need not an aromatic framework as support. For instance, benzoylpyruvates 277 react with 5,6-diaminopyrimidine-2,4-dione derivatives 278 yielding dihydropteridine-2,4,6-trione 279 and 280 (Scheme 96).146 It has been demonstrated that, depending on whether the reaction media is basic or acidic, it is possible to influence the regioselectivity residing on the non-symmetrical nature of the N,N`-dinucleophile.
2.3.13. TRIAZOLO[3,4-a]PHTHALAZINES
Cyclocondensation of 1-hydrazinophthalazine 281 with substituted ethyl benzoylpyruvates 282 gave under neutral reaction conditions, 3-[2-oxo-2-(substituted phenyl)ethyl]-4H-as-triazino[3,4-a]phthalazin-4-ones 283. Under acidic but otherwise identical conditions, depending on the substituent, cyclocondensation gave 3-carbethoxy-s-triazolo[3,4-a]phthalazine 28479 (Scheme 97).
2.4. SEVEN MEMBERED RING
Cyclocondensation of ethyl 1,2,3,4-tetrahydro-1-oxo-2-naphthaleneglyoxylate with o-phenylenediamine gave 78% 285 which was treated with hydroxylamine hydrochloride to give 82% 286. Amination of 285 by various amines and sulfa compounds gave 63-74% benzonaphtho-1,4-diazepines 287 (Scheme 98) which were useful as bactericides.147
Benzoylpyruvates 288, act as α-keto-ester on cyclodehydration with 289 led to intermediate β-enaminone 290 and then to 1,2-dihydrobenzo[e][1,4]oxazepin-3(5H)-one 291 by refluxing in acetic anhydride (Scheme 99).148
DIFFERENT REACTIONS
The self-condensation reaction of β-acylpyruvates 292 in the presence of 1,4-diazabicyclo[2.2.2]octane (DABCO) afforded 4,5,5-trisubstituted tetrahydrofuran-2,3-diones derivatives 294 (Scheme 100).149,150
6-(1-Methylhydrazino)isocytosine 295 cyclizes with α,γ-dioxo esters 296 to give pyrimido[4,5-c]pyridazines 297, 298 and 1H-pyrimido[4,5-c]-1,2-diazepines 299 and 300, the latter being predominant in each case (Scheme 101).151
Cyclic monodithioacetals 301 of ethyl acetylpyruvate was prepared in 80% yield using boron trifluoride etherate as the acid catalyst (Scheme 102).152
Tetracyclen 303 was prepared in 60% yield by reaction of bis(4,5-dihydro-1H-imidazol-2-yl)methane 302 with ethyl 2,4-dioxopentanoate (Scheme 103).153
1,2,3-Triazolo-1,2,3-triazine salts 306 were prepared in 27-94% yields by cyclocondensation of the corresponding 1-aminotriazole 304 with ethyl acylpyruvates 305 (Scheme 104).154
The condensation of 2,5-diamino-4-methylamino-6-oxo-1,6-dihydropyrimidine 307 with ethyl acetopyruvate gave 8,9-dimethylguanine 308 and isoxanthopterin 309 at pH 1, and 8-methyl-6-acetonylisoxanthopterin 310 at pH 5 (Scheme 105).155
Ethyl 2,4-dioxopentanoate was added to 3-hydroxy-4-thiacyclohexanone 311 in potassium hydroxide several weeks followed by acidification with acetic acid and then treatment with sodium nitrite and finally with Zn dust, to give ethyl 4,5,6,7-tetrahydro-2-methyl-1-aza-6-thiaindene-3-carboxylate 312 (Scheme 106).51
Ethyl (2-furoyl)pyruvate underwent Mannich alkylation to give the β-furoyl-β-(arylmethyl)pyruvate 313 (Scheme 107).62
Ethyl 4-aryl-2,4-dioxobutanoates 314 undergo intramolecular Wittig reaction with a vinyltriphenylphosphonium salt to yield cyclobutene derivative 315 which undergo electrocyclic ring-opening reactions in boiling toluene to produced highly electron-deficient 1,3-dienes 316 in quantitative yields (Scheme 108).156
The mechanism involves addition-cyclization products apparently result from initial addition of triphenylphosphine to the acetylenic ester and concomitant protonation of the 1:1 adduct 317, followed by attack of the anion of 314 to vinyltriphenylphosphonium cation to form a phosphorane 318, which is converted to strained carbocyclic ring system 316 (Scheme 109).156
Reaction of hydrazines 319, with ethyl 2,4-dioxo-4-phenylbutanoate in absolute ethanol at 60 °C gave 91% hydrazones 320 (Scheme 110).157
ACKNOWLEDGEMENTS
The authors would like to thank Abdelbasset A. Farahat, chemistry department, Georgia State University, Atlanta, Georgia, USA for helping in data collection and refining.
References
1. H. Moser, B. Boehner, and W. Foery, EP 268, 554 (Chem. Abstr., 1988, 110, 23879); T. Ishii, H. Shimortori, Y. Tanaka, and K. Ishikawa, JP 01, 168, 675 (Chem. Abstr., 1989, 112, 35854); I. Okada, K. Yoshida, and M. Sekine, JP 02, 292, 263 (Chem. Abstr., 1990, 114, 185497).
2. E. Sohn, R. Handle, H. Mildenberger, H. Buerstell, K. Bauer, and H. Bieringer, Ger. Patent 3, 633, 840 (Chem. Abstr., 1988, 110, 8202).
3. P. G. Baraldi, A. Barco, S. Benetti, S. Manfredini, G. P. Pollini, and D. Simoni, Tetrahedron, 1987, 43, 235. CrossRef
4. J. M. J. Nolsoe and D. Weigelt, J. Heterocyclic Chem., 2009, 46, 1.
5. A. Cox, In Comprehensive Organic Chemistry; ed. by D. H. R. Barton, W. D. Ollis, I. O. Sutherland, Pergamon Press, New York, 1969; Vol. 2, p. 702.
6. J. Zhang, S. Didierlaurent, M. Fortin, D. Lefrancois, E. Uridat, and J. P. Vevert, Bioorg. Med. Chem. Lett., 2000, 10, 2575. CrossRef
7. F. Wei, B.-X. Zhao, B. Huang, L. Zhang, C.-H. Sun, W.-L. Dong, D.-S. Shin, and J.-Y. Miao, Bioorg. Med. Chem. Lett., 2006, 16, 6342. CrossRef
8. N. W. Fadnavis and K. R. Radhika, Tetrahedron: Asymmetry, 2004, 15, 3443. CrossRef
9. R. Braga, L. Hecquet, and C. Blonski, Bioorg. Med. Chem., 2004, 12, 2965. CrossRef
10. Y. Pei and B. O. S. Wickham, Tetrahedron Lett., 1993, 34, 7509. CrossRef
11. Y. Wakabayashi, A. Miyata, and H. Sato, JP 04149154(1992) (Chem. Abstr., 1992, 117, 170989).
12. S. Imaki, J. Takuma, and K. Endo, Jpn. Kokai Tokkyo Koho JP04069361 (1992) (Chem. Abstr., 1992, 117, 7522).
13. S. Matsutani, K. Hirai, M. Tsutsumiuchi, and T. Mizui, JP 61134346 (1986) (Chem. Abstr., 1986, 105, 225805).
14. C. Musante and S. Fatutta, Gazz. Chim. Ital., 1958, 88, 879.
15. S. Fatutta, Gazz. Chim. Ital., 1959, 89, 964.
16. L. M. Oh, H. Wang, S. C. Shilcrat, R. E. Herrmann, D. B. Patience, P. G. Spoors, and J. Sisko, Org. Proc. Res. Develop., 2007, 11, 1032. CrossRef
17. K. M. Ghoneim, M. M. Badran, M. A. Shaaban, and S. El-Meligie, Egypt. J. Pharm. Sci., 1988, 29, 571 (Chem. Abstr., 1989, 111, 23436).
18. V. P. Gorbunova and N. N. Suvorov, Chem. Heterocycl. Compd. (N. Y., NY, U. S), 1977, 14, 754.
19. S. Ferro, A. Rao, M. Zappala, A. Chimirri, M. L. Barreca, M. Witriam, Z. Debyser, and P. Monforte, Heterocycles, 2004, 63, 2727. CrossRef
20. Y. A. Ibrahim, B. Al-Saleh, and A.-A. A. Mahmoud, Tetrahedron, 2003, 59, 8489. CrossRef
21. F. Kipnis, I. Levy, and J. Ornfelt, J. Am. Chem. Soc., 1948, 70, 4265, Figure 1. CrossRef
22. R. W. Hamilton, J. Heterocycl. Chem., 1976, 13, 545. CrossRef
23. H. M. Faidallah, S. A. Basaif, E. M. Sharshira, and A.A-Ba-Oum, Egypt. J. Chem., 2002, 45, 905 (Chem. Abstr., 2004, 141, 410854).
24. G. A. Pinna, M. A. Pirisi, J.-M. Mussinu, G. Murineddu, G. Loriga, A. Pau, and G. E. Grella, Farmaco, 2003, 58, 749.
25. F. Campagna, F. Palluotto, A. Carotti, and E. Maciocco, Farmaco, 2004, 59, 849. CrossRef
26. J.-M. Mussinu, S. Ruiu, A. C. Mule, A. Pau, M. A. M. Carai, G. Loriga, G. Murineddu, and G. A. Pinna, Bioorg. Med. Chem., 2003, 11, 251. CrossRef
27. S. Patil, S. Kamath, T. Sanchez, N. Neamati, R. F. Schinazi, and J. K. Buolamwini, Bioorg. Med. Chem., 2007, 15, 1212. CrossRef
28. V. Blandin, J.-F. Carpentier, and A. Mortreux, Tetrahedron: Asym., 1998, 9, 2765. CrossRef
29. V. Blandin, J.-F. Carpentier, and A. Mortreux, Eur. J. Org. Chem., 1999, 8, 1787. CrossRef
30. G. I. Chipens, V. A. Slavinskaya, A. K. Strautinya, D. R. Kreile, D. E. Sile, E. Kh. Korchagova, and O. M. Galkin, Structure and Action of Inhibitors of Zinc-Containing Enzymes – Kinase II and Encephalinase [in Russian], Zinatne, Riga (1990), p. 238.
31. V. Slavinska, D. Sile, E. Korchagova, and M. Katkevich, Synth. Commun., 1996, 26, 2229. CrossRef
32. V. Slavinska, D. Sile, G. Rozenthal, G. Maurops, J. Popelis, M. Katkevich, V. Stonkus, and E. Lukevics, Chem. Heterocycl. Compd. (N. Y., NY, U. S), 2006, 42, 570. CrossRef
33. N. W. Fadnavis, K. R. Radhika, and D. A. Vedamayee, Tetrahedron: Asymmetry, 2006, 17, 240. CrossRef
34. P. G. Baraldi, S. Manfredini, G. P. Pollini, R. Romagnoli, and V. Z. Simoni, Tetrahedron Lett., 1992, 33, 2871. CrossRef
35. R. Oehrlein and G. Baisch, PCT Int. Appl. WO 2002040438 (2002) (Chem. Abstr., 2002, 136, 385942).
36. S. Manfredini, D. Simoni, V. Zanirato, and A. Casolari, Tetrahedron Lett., 1988, 29, 3997. CrossRef
37. D. W. Emerson, R. L. Titus, and R. M. Gonzaez, J. Org. Chem., 1991, 56, 5301. CrossRef
38. A. Haider and H. Wyler, Helv. Chim. Acta, 1983, 66, 606. CrossRef
39. F. Chen and L. Zhao, CN 1995006 (2007) (Chem. Abstr., 2007, 147, 18943).
40. T. Lu, T. Markotan, F. Coppo, B. Tomczuk, C. Crysler, S. Eisennagel, J. Spurlino, L. Gremminger, R. M. Soll, E. C. Giardino, and R. Bone, Bioorg. Med. Chem. Lett., 2004, 14, 3727. CrossRef
41. E. Matsumura, M. Ariga, and Y. Tohda, Tetrahedron Lett., 1979, 16, 1393. CrossRef
42. A. N. Maslivets, O. P. Krasnykh, and Yu. S. Andreichikov, Zh. Organ. Kh., 1988, 24, 2233.
43. M. Dohrn and A. Thiele, Ber., 1931, 64B, 2863 (Chem. Abstr., 1932, 26, 1928).
44. Y. Chigira, M. Masaki, and M. Ohta, Chem. Pharm. Bull., 1969, 42, 228.
45. E. M. Afsah, H. A. Etman, W. S. Hamama, and A. F. Sayed, Boll. Chim. Farmaceutico, 1995, 134, 547 (Chem. Abstr., 1996, 124, 260756).
46. Yu. S. Andreichikov, V. L. Gein, and I. N. Anikina, Chem. Heterocycl. Compd. (N. Y., NY, U. S), 1985, 21, 1178 (Chem. Abstr., 1987, 107, 39766). CrossRef
47. N. M. S. Hasanien, Studies on Claisen condensation and its products. M. Sc. Thesis, Faculty of Science, Mansoura University, 1996.
48. A. G. Schering, M. Dohrn, and H. Nahme, Ger. 678, 152, 1939 (Chem. Abstr., 1939, 33, 7963).
49. D. W. Emerson, R. L. Titus, and M. D. Jones, J. Heterocycl. Chem., 1998, 35, 611. CrossRef
50. M. K. A. Khan, K. J. Morgan, and D. P. Morrey, Tetrahedron, 1966, 22, 2095. CrossRef
51. G. H. Cookson, J. Chem. Soc., 1953, 2789. CrossRef
52. H. Takaya, S. Kojima, and S.-I. Murahashi, Org. Lett., 2001, 3, 421. CrossRef
53. J. Vorkapic-Furac and M. Suprina, Z. Chemie, 1989, 29, 176 (Chem. Abstr., 1990, 112, 76847).
54. J. Büchi, H. R. Meyer, R. Hirt, F. Hunziker, E. Eichenberger, and R. Lieberherr, Helv. Chim. Acta, 1955, 79, 670. CrossRef
55. L. M. Subramanian and G. S. Misra, Synthesis, 1984, 1063. CrossRef
56. F. Arano, D. Catarzi, V. Colotta, G. Filacchioni, A. Galli, C. Costagli, and V.Carlá, J. Med. Chem., 2002, 45, 1035. CrossRef
57. T. Herk, J. Brussee, A. M. C. H. Nieuwendijk, P. A. M. Klein, A. P. Ijzerman, C. Stannek, A. Burmeister, and A. Lorenzen, J. Med. Chem., 2003, 46, 3945. CrossRef
58. T. Majid, C. R. Hopkins, B. Pedgrift, and N. Collar, Tetrahedron Lett., 2004, 45, 2137. CrossRef
59. G. Szabo, J. Fischer, and A. Kis-Varga, Pharmazie, 2006, 61, 522.
60. H. Yoshida, K. Oomori, M. Yokoi, and K. Fuse, Jpn. Kokai Tokkyo Koho JP 07118238 (1995) (Chem. Abstr., 1995, 123, 169615).
61. K. Harada, S. Nishino, and T. Harada, Jpn. Kokai Tokkyo Koho JP 2001335564 (2001) (Chem. Abstr., 2001, 136, 5989).
62. R. M. Saleh and I. M. El-Deen, Rev. Roumain. de Chim., 1993, 38, 1333 (Chem. Abstr., 1994, 121, 108560).
63. G. J. Reddy, D. Latha, and K. S. Rao, Org. Prep. Proc. Int., 2004, 36, 494. CrossRef
64. E. Sohn, H. Mildenberger, K. Bauer, and H. Bieringer, EP 333131 (1989) (Chem. Abstr., 1990, 112, 153721).
65. J. M. Smallheer, R. S. Alexander, J. Wang, S. Wang, S. Nakajima, K. A. Rossi, A. Smallwood, F. Barbera, D. Burdick, J. M. Luettgen, R. M. Knabb, R. R. Wexler, and P. K. Jadhav, Bioorg. Med. Chem. Lett., 2004, 14, 5263. CrossRef
66. C. Tan, D. Shen, J. Weng, and D. Fan, Zhejiang Daxue Xuebao, Lixueban, 2005, 32, 204 (Chem. Abstr., 2005, 144, 369957).
67. J. Yang and Y. Tian, Xiandai Nongyao, 2002, 1, 11 (Chem. Abstr., 2002, 138, 221511).
68. J. Yang and Y. Tian, CN 1305996 (2001) (Chem. Abstr., 2002, 137, 140519).
69. M. J. S. Dewar and F. E. King, J. Chem. Soc., 1945, 114. CrossRef
70. C. Tan, D. Shen, J. Weng, and N. Sun, Zhejiang Gongye Daxue Xuebao, 2005, 33, 331 (Chem. Abstr., 2005, 144, 294684).
71. B. Gu, W. Zhu, and W. Fan, Xiandai Nongyao, 2002, 1, 9 (Chem. Abstr., 2003, 139, 52927).
72. M. Nakagawa, S. Hara, and M. Sogawa, JP 04224565 (1992) (Chem. Abstr., 1992, 117, 251349).
73. F. Lepage and B. Hublot, EP 459887 (1991) (Chem. Abstr., 1992, 116, 128914).
74. J. L. Huppatz, Aust. J. Chem., 1983, 36, 135 (Chem. Abstr., 1983, 98, 160637).
75. S. C. McKeown, A. Hall, G. M. P. Giblin, O. Lorthioir, R. Blunt, X. Q. Lewell, R. J. Wilson, S. H. Brown, A. Chowdhury, T. Coleman, S. P. Watson, I. P. Chessell, A. Pipe, N. Claytonb, and P. Goldsmith, Bioorg. Med. Chem. Lett., 2006, 16, 4767. CrossRef
76. N. Pommery, T. Taverne, A. Telliez, L. Goossens, C. Charlier, J. Pommery, J.-F. Goossens, R. Houssin, F. Durant, and J.-P. He´nichart, J. Med. Chem., 2004, 47, 6195. CrossRef
77. S. Barbey, L. Goossens, T. Taverne, J. Cornet, V. Choesmel, C. Rouaud, G. Gimeno, S. Yannic-Arnoult, C. Michaux, C. Charlier, R. Houssin, and J.-P. Henichart, Bioorg. Med. Chem. Lett., 2002, 12, 779. CrossRef
78. A. C. Donohue, S. Pallich, and T. D. McCarthy, J. Chem. Soc., Perkin Trans. 1, 2001, 21, 2817. CrossRef
79. A. Amer, K. Weisz, and H. Zimmer, Heterocycles, 1987, 26, 1853. CrossRef
80. R. M. Saleh and I. M. El-Deen, J. Serbian Chem. Soc., 1991, 56, 595 (Chem. Abstr., 1992, 116, 106163).
81. B. F. Abdel-Wahab and A.-A. S. El-Ahl, Phosphorus, Sulfur Silicon Relat. Elem., (2009), in press.
82. B. F. Abdel-Wahab, H. A. Abdel-Aziz, and E. M. Ahmed, Arch. Pharm. (Weinheim, Ger), 2008, 341, 734.
83. B. F. Abdel-Wahab, H. A. Abdel-Aziz, and E. M. Ahmed, Monatsh. Chem., 2009, 140, 601. CrossRef
84. A. Fruchier, B. Lupo, and G. Tarrago, Can. J. Chem., 1985, 63, 375. CrossRef
85. J. Yuan, M. Gulianello, S. De Lombaert, R. Brodbeck, A. Kieltyka, and K. J. Hodgetts, Bioorg. Med. Chem. Lett., 2002, 12, 2133. CrossRef
86. D. J. P. Pinto, M. J. Orwat, S. Wang, J. M. Fevig, M. L. Quan, E. Amparo, J. Cacciola, K. A. Rossi, R. S. Alexander, A. M. Smallwood, J. M. Luettgen, L. Liang, B. J. Aungst, M. R. Wright, R. M. Knabb, P. C. Wong, R. R. Wexler, and P. Y. S. Lam, J. Med. Chem., 2001, 44, 566. CrossRef
87. W. T. Ashton and G. A. A. Doss, J. Heterocycl. Chem., 1993, 30, 307. CrossRef
88. J. R. Pruitt, D. J. P. Pinto, R. A. Galemmo, R. S. Alexander, K. A. Rossi, B. L. Wells, S. Drummond, L. L. Bostrom, D. Burdick, R. Bruckner, H. Chen, A. Smallwood, P. C. Wong, M. R. Wright, S. Bai, J. M. Luettgen, R. M. Knabb, P. Y. S. Lam, and R. R. Wexler, J. Med. Chem., 2003, 46, 5298. CrossRef
89. Z. J. Jia, Y. Wu, W. Huang, E. Goldman, P. Zhang, J. Woolfrey, P. Wong, B. Huang, U. Sinha, G. Park, A. Reed, R. M. Scarborough, and B.-Y. Zhu, Bioorg. Med. Chem. Lett., 2002, 12, 1651. CrossRef
90. D. Berta, M. Villa, A. Vulpeti, and E. Felder, Tetrahedron, 2005, 61, 10801. CrossRef
91. P. Herold, A. F. Indolese, M. Studer, H. P. Jalett, U. Siegrist, and H. U. Blaser, Tetrahedron, 2000, 56, 6497. CrossRef
92. A. Schmidt, T. Habeck, M. K. Kindermann, and M. Nieger, J. Org. Chem., 2003, 68, 5977. CrossRef
93. S. Fustero, R. Roman, J. F. Sanz-Cervera, A. Simon-Fuentes, J. Bueno, and S. Villanova, J. Org. Chem., 2008, 73, 8545. CrossRef
94. S. Fustero, R. Román, J. F. Sanz-Cervera, A. Simon-Fuentes, A. C. Cunat, S. Villanova, and M. Murgui, J. Org. Chem., 2008, 73, 3523. CrossRef
95. Z.-X. Wang and H.-L. Qin, Green Chem., 2004, 6, 90. CrossRef
96. A. Attayibat, S. Radi, A. Ramdani, Y. Lekchiri, B. Hacht, M. Bacquet, S. Willai, and M. Morcellet, Bull. Korean Chem. Soc., 2006, 27, 1648.
97. G. Menozzi, L. Mosti, P. Schenone, D. Donnoli, F. Schiariti, and E. Marmo, Farmaco, 1990, 45, 167.
98. C. B. Barrett, R. J. S. Beer, G. M. Dodd, and A. Robertson, J. Chem. Soc., 1957, 4810. CrossRef
99. V. P. Gorbunova and N. N. Suvorov, Chem. Heterocycl. Compd. (N. Y., NY, U. S), 1972, 9, 1374. CrossRef
100. J. M. Fevig, J. Cacciola, J. Buriak, K. A. Rossi, R. M. Knabb, J. M. Luettgen, P. C. Wong, S. A. Bai, R. R.Wexler, and P. Y. S. Lam, Bioorg. Med. Chem. Lett., 2006, 16, 3755. CrossRef
101. D. Bierowska-Charytonowicz and M. Konieczny, Arch. Immun. Therap. Exp., 1976, 24, 871 (Chem. Abstr., 1977, 87, 53221).
102. A. Amer, N. Rashed, M. M. Abdel Rahman, and H. Zimmer, Heterocycles, 1987, 26, 1277. CrossRef
103. A. K. Roy and S. Batra, Synthesis, 2003, 2325. CrossRef
104. G. Biagi, I. Giorgi, O. Livi, V. Scartoni, L. Betti, G. Giannaccini, and M. L. Trincavelli, Eur. J. Med. Chem., 2002, 37, 565. CrossRef
105. P. G. Baraldi, A. Barco, S. Benetti, S. Manfredini, G. P. Pollini, and D. Simoni Tetrahedron Lett., 1984, 25, 4313. CrossRef
106. N. K. Kochetkov and S. D. Sokolov, "Advances in Heterocyclic Chemistry", ed. by A. R. Katritzky, Academic Press, New York, 1963.
107. P. A. Pieper, D. Yang, H. Zhou, and H. Liu, J. Am. Chem. Soc., 1997, 119, 1809. CrossRef
108. H. Henecka, Chem. Ber., 1949, 82, 36. CrossRef
109. Y. Oka, K. Omura, A. Miyake, K. Itoh, M. Tomimoto, N. Tada, and S. Yuruga, Chem. Pharm. Bull., 1975, 23, 2239.
110. J. Suh and Y. Hong, Arch. Pharm. Res., 1990, 13, 257. CrossRef
111. A. Palani, S. Shapiro, J. W. Clader, W. J. Greenlee, S. Vice, S. McCombie, K. Cox, J. Strizki, and B. M. Baroudy, Bioorg. Med. Chem. Lett., 2003, 13, 709. CrossRef
112. L. Valgimigli, G. Brigati, G. F. Pedulli, G. A. DiLabio, M. Mastragostino, C. Arbizzani, and D. A. Pratt, Chem. Eur. J., 2003, 9, 4997. CrossRef
113. D. V. Sevenard, O. G. Khomutov, O. V. Koryakova, V. V. Sattarova, M. I. Kodess J. Stelten, I. Loop, E. Lork, K. I. Pashkevich, and G.-V. Roschenthaler, Synthesis, 2000, 1738. CrossRef
114. C. R. Hauser and R. M. Manyik, J. Org. Chem., 1953, 18, 588. CrossRef
115. A. H. Abd-El-Rahman, F. A. Amer, E. M. Kandeel, and S. I. El-Desoky, Egypt J. Chem., 1990, 31, 59.
116. J. Lee, H. J. Kim, S. Choi, H. G. Choi, S. Yoon, J.-H. Kim, K. Jo, S. Kim, S.-Y. Koo, M.-H. Kim, J. I. Kim, S.-Y. Hong, M. S. Kim, S. Ahn, H.-S. Yoon, and H.-S. Cho, PCT Int Appl WO 2004/080979 (2004) (Chem. Abstr., 2004, 141, 277637).
117. M. M. Eid, M. A. Badawy, M. A. H. Ghazala, and Y. A. Ibrahim, J. Heterocycl. Chem., 1983, 20, 1709. CrossRef
118. V. L. Gein, N. N. Kasimova, M. A. Panina, and E. V. Voronina, Pharm. Chem. J., 2006, 40, 410. CrossRef
119. V. L. Gein, L. F. Gein, and I. A. Shevchenko, Russ. J. Gen. Chem., 2003, 73, 661. CrossRef
120. S. A. Abdel-Hady, M. A. Badawy, A. M. Kadry, and Y. A. Ibrahim, Sulfur Lett., 1988, 8, 153.
121. Y. A. Ibrahim, S. A. L. Abdel-Hady, M. A. Badawy, and M. A. H. Ghazala, J. Heterocycl. Chem., 1982, 19, 913. CrossRef
122. Y. S. Andreichikov, S. V. Kol’tsova, I. A. Zhikina, and D. D. Nekrasov, Russ. J. Org. Chem., 1999, 35, 1538.
123. H. Sheibani, M. R. Islami, H. Khabazzadeh, and K. Saidi, Tetrahedron, 2004, 60, 5931. CrossRef
124. C. Cano, P. Goya, J. A. Paez, R. Giron, and E. Sanchez, Bioorg. Med. Chem., 2007, 15, 7480. CrossRef
125. H. K. Ibrahim, S. H. El-Tamany, R. F. El-Shaarawy, and I. M. El-Deen, Macedonian J. Chem. Eng., 2008, 27, 65.
126. O. Migliara, S. Petruso, and V. Sprio, J. Heterocycl. Chem., 1979, 16, 577. CrossRef
127. G. Auzzi, L. Cecchi, A. Costanzo, and L. Pecori Vettori, Farmaco, 1979, 34, 898.
128. G. Auzzi, L. Cecchi, A. Costanzo, L. Pecori Vettori, F. Bruni, R. Pirisino, and G. B. Ciottoli, Farmaco, 1979, 34, 478.
129. R. Balicki, Polish J. Chem., 1983, 57, 1251 (Chem. Abstr., 1985, 103, 87802).
130. O. Migliara, S. Petruso, and V. Sprio, J. Heterocycl. Chem., 1979, 16, 1105. CrossRef
131. M. P. Giovannoni, C. Vergelli, C. Ghelardini, N. Galeotti, A. Bartolini, and V. Dal Piaz, J. Med. Chem., 2003, 46, 1055. CrossRef
132. T. Mulamba, R. El Boukili-Garre, D. Seraphin, E. Noe, C. Charlet-Fagnere, J. Henin, J. Laronze, J. Sapi, and R. Barret, Heterocycles, 1995, 41, 29. CrossRef
133. I. M. El-Deen and F. F. Mahmoud, Phosphorus, Sulfur and Silicon Relat. Elem., 2000, 165, 205. CrossRef
134. D. G. Markees, J. Heterocycl. Chem., 1989, 26, 29. CrossRef
135. R. B. Palkar and H. E.Master, Indian J. Heterocycl. Chem., 2000, 10, 37.
136. I. V. Mashevskaya, R. R. Makhmudov, G. A. Aleksandrova, O. V. Golovnina, A. V. Duvalov, and A. N. Maslivets, Pharm. Chem. J., 2001, 35, 196. CrossRef
137. K. S. Bozdyreva, I. V. Smirnova, and A. N. Maslivets, Russ. J. Org. Chem., 2005, 41, 1081. CrossRef
138. N. Rashed, A. M. El-Massry, E. H. El-Ashry, and A. Amer, J. Heterocyl. Chem., 1990, 27, 691.
139. M. Xia, B. Wu, and G.-F. Xiang, Synth. Commun., 2008, 38, 1268. CrossRef
140. D. G. Orphanos and A. Taurins, Can .J. Chem., 1966, 44, 1795. CrossRef
141. Z. G. Aliev, O. P. Krasnykh, A. N. Maslivets, and L. O. Atovmyan, Russ. Chem. Bull., 2000, 49, 2045. CrossRef
142. V. L. Gein, N. A. Rassudikhina, and E. V. Voronina, Pharm. Chem. J., 2006, 40, 554. CrossRef
143. A. Atfah and J. Hill, J. Chem. Soc., Perkin Trans. 1, 1989, 221. CrossRef
144. G. A. M. Giardina, M. Artico, S. Cavagnera, A. Cerri, E. Consolandi, S. Gagliardi, D. Graziani, M. Grugni, D. W. P. Hay, M. A. Luttmann, R. Mena, L. F. Raveglia, R. Rigolio, H. M. Sarau, D. B. Schmidt, G. Zanoni, and C. Farina, Farmaco, 1999, 54, 364. CrossRef
145. M. C. Venuti, Synthesis, 1982, 61. CrossRef
146. S. A. L. Abdel-Hady, M. A. Badawy, M. A. N. Mosselhi, and Y. A. Ibrahim, J. Heterocycl. Chem., 1985, 22, 801. CrossRef
147. A. Zayed and J. Metri, Egypt. J. Chem., 1981, 24, 481 (Chem. Abstr., 1983, 99, 70679).
148. N. V. Kolotova, V. O. Kozminykh, E. V. Dolbilkina, and E. N. Kozminykh, Russ. Chem. Bull., 1998, 47, 2246. CrossRef
149. H. J. Lee, T. Y. Kim, and J. N. Kim, Synth. Commun., 1999, 29, 4375. CrossRef
150. J. N. Kim, H. J. Lee, K. Y. Lee, H. R. Kim, and E. K. Ryu, Bull. Korean Chem. Soc., 1999, 20, 1375 (Chem. Abstr., 2000, 133, 73900).
151. W. R. Mallory, R. W. Morrison, and V. L. Styles, J. Org. Chem., 1982, 47, 667. CrossRef
152. M. T. Barros, C. F. G. C. Geraldes, C. D. Maycock, and M. I. Silva, Tetrahedron, 1988, 44, 2283. CrossRef
153. H. Meyer and J. Kurz, Liebigs Ann. Chem., 1978, 1491.
154. V. A. Chuiguk, G. N. Poshtaruk, and V. A. Goroshko, Ukrainskii Khim. Zh. (Russ. Ed.), 1981, 47, 76 (Chem. Abstr., 1981, 94, 175002).
155. G. B. Barlin and W. Pfleiderer, Chem. Ber., 1969, 102, 4032 (Chem. Abstr., 1970, 72, 43613). CrossRef
156. I. Yavary and A. R. Samzadeh-Kermani, Tetrahedron Lett., 1998, 39, 6343. CrossRef
157. F. V. Bagrov, T. V. Vasil'eva, and D. F. Bagrov, Zh. Obsh. Khim., 1994, 64, 1475 (Chem. Abstr., 1995, 123, 9523).