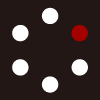
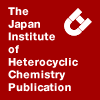
HETEROCYCLES
An International Journal for Reviews and Communications in Heterocyclic ChemistryWeb Edition ISSN: 1881-0942
Published online by The Japan Institute of Heterocyclic Chemistry
e-Journal
Full Text HTML
Received, 2nd October, 2009, Accepted, 22nd December, 2009, Published online, 25th December, 2009.
DOI: 10.3987/COM-09-11849
■ 15N NMR Spectroscopy of Annulated Δ2-Pyrazolines and Δ2-1,2,4-Triazolines
Teresa Recca, Lara De Benassuti, and Giorgio Molteni*
Department of Organic and Industrial Chemistry, University of Milano, Via Golgi 19, 20133 Milano, Italy
Abstract
Enantiopure Δ2-pyrazolines and Δ2-1,2,4-triazolines fused to the 1,4-benzodiazepine moiety, as well as Δ2-pyrazolines annulated to the 1,5-benzoxazocine moiety (racemic) or inserted in a bis-1,3-pyrazolophane skeleton (enantiopure) were investigated through 15N NMR spectroscopy in natural abundance. Nitrogen chemical shifts were determined by (1D)-INEPT experiments, while proton-nitrogen scalar coupling were obtained through 2D-J-HMBC experimentsDue to the occurrence of nitrogen atoms in a huge number of heterocyclic rings, their study through 15N NMR spectroscopy may appear as a logical consequence in order to obtain a better knowledge of their own structures. As a matter of fact, increasing attention have been payed to this interesting topic1 and, in particular, the chemical shifts of some 15N-enriched azoles have been investigated by 15N NMR spectroscopy.2 Several efforts have also been made in studying 15N NMR of azoles in natural abundance.3 Within this field, early papers by Elguero and coworkers4 are concerned to simple Δ2-pyrazoline derivatives, while our recent work5 deals with the spectroscopic data of a series of 1-(4-substituted)phenyl-3-methoxycarbonyl-5-ethoxycarbonyl-Δ2-pyrazolines. Having firmly established the basic spectroscopic features of simple Δ2-pyrazolines within the frame of 15N NMR spectroscopy, we turned our attention to the study of annulated Δ2-pyrazolines and Δ2-1,2,4-triazolines, namely 3,3a-dihydro-pyrazolo[1,5-a][1,4]benzodiazepine-4(6H)-ones 1 and [1,2,4]triazolo[4,3-a][1,4]benzo diazepinones 2 (Figure 1), in natural abundance. These heterocyclic skeletons are particularly attracting since they belong to the class of annulated 1,4-benzodiazepines, which occupy a prominent place among drugs for the treatment of CNS disturbances.6 Some significant representatives are alprazolam, a common anxiolytic agent,7 and flumazenil8 which belongs to the family of cognition enhancers. Other interesting Δ2-pyrazolines annulated to the 1,5-benzoxazocine moiety (compounds 3 and 4, racemic) or inserted in a bis-1,3-pyrazolophane skeleton (compound 5, enantiopure) have also been investigated.
In order to gain deeper insights about the spectroscopic features of compounds 1-5 we undertook their comprehensive 15N NMR analyses.
All the substrates 1-5 submitted to 15N NMR analyses (Figure 1) were synthesised via nitrilimine cycloaddition onto the appropriate dipolarophile. Nitrilimine intermediates were generated in situ by treating the corresponding hydrazonoyl chloride with silver carbonate as the basic agent, according to a procedure developed in our laboratory.9
In the cases where a chiral pendant is present a stereoselective cycloaddition took place giving mixtures of the possible enantiopure diastereoisomers.10 In the present paper we examined the spectral properties of the major diastereoisomers 1a,b, 2a,b and 5 in the enantiopure form, whose absolute configurations are depicted in Figure 1 and were assigned previously on the basis of X-ray diffractometric analyses.11 As far as racemic substrates 3 and 4 are concerned, heir structure were assigned unambiguously by analytical and spectral data.12
All the experiments reported in the present paper were performed in natural abundance thus avoiding the synthesis of 15N-enriched compounds. Futhermore, the lack of 15N labeled atoms did not lead to excessive time-consuming experiments.
The 15N nuclear shielding values of compounds 1-5, which resulted from (1D)-INEPT pulse sequences, are given in Table 1. It is known that nitrogen chemical shift (δN) of some aromatic azoles can be affected by the solvent,13 however these changes occur because of explicit hydrogen bondings which are clearly absent in the present case. Hence, all experiments were performed in CDCl3 as the solvent, while sample concentration was always 0.25 M.
According to Table 1, the chemical shift values of N1 and N2 vary due to their hybridisation. It is apparent that sp2-hybridised N2 falls downfield with respect to sp3-hybridised N1. In other words N2 atoms appear strongly deshielded with respect to N1, a result which agrees with the known chemical shifts of both sp2 and sp3 nitrogens of simple heterocycles.14 Some fluctuation in the N2 chemical shift values appears from Table 1 according to their chemical environment. In particular, sp2-hybridised N2 contained in the 1,2,4-triazoline ring of 2a,b appears shielded with respect to their counterparts in the pyrazoline ring of compounds 1a,b. Such a behaviour is hardly rationalisable on the basis of simple inductive and hybridisation effects. In fact, it may be recalled that some nitrogens experience an upfield shift due to the dominance of the increase in molecular-plane shielding over the decrease in the out-of-plane shielding.15 This latter statement can be applied to the present case since structures of compounds 1a and 2a provided by X-ray crystallographic analysis show better planarity for the 1,2,4-triazoline ring. Compounds 1a,b and 2a,b display both N1 and N3 or N3 and N4, respectively, whose chemical shifts are difficult to assign unambiguosly on the basis of the above-mentioned (1D)-INEPT experiment. In the case of such compounds 2D-HMBC experiments were required to attain this latter purpose. As far as racemic benzoxazocine-annulated pyrazoles 3 and 4 are concerned, their N1 and N2 chemical shifts finds a rationale in the light of the above considerations. The N1 and N2 chemical shifts of enantiopure bis-1,3-pyrazolophane 5 just match with that observed in the case of simple Δ2-pyrazolines.5
Next, we measured the 15Nx-C-C-H scalar couplings of compounds 1-5 by means of 2D-J-HMBC experiments. The 3J values reported in Table 2 encompasses the range 3.3-4.2 Hz in the case of compounds 1-4, which are consistent with literature data.16 As far as bis-1,3-pyrazolophane 5 is concerned, larger values of 3J were observed, namely between 6.0 and 6.2 Hz. It needs to be underlined the perfect agreement between these latter data and those previously found for simple Δ2-pyrazolines.5
Novel 15N NMR spectroscopic data, namely chemical shifts and scalar coupling constants, have been obtained for some enantiopure Δ2-pyrazolines and Δ2-1,2,4-triazolines fused to the 1,4-benzodiazepine moiety, as well as for racemic pyrazolo[1,5-a][5,1]benzoxazocines or enantiopure bis-1,3-pyrazolophane.
EXPERIMENTAL
Compounds 1a,b,11a 2a,b,11b 3,12a 4,12b and 511c are known in the literature.
15N NMR spectroscopic experiments.
NMR spectra were acquired on a Bruker Avance 400 MHz (40.557 MHz for 15N) or on AMX 300 MHz (30.424 MHz for 15N) spectrometer, both equipped with a 5 mm inverse z-gradient probe.
15N chemical shifts were measured via (1D)-INEPT experiments, which were recorded over a range of 300 ppm with a J value of 4 Hz and a relaxation delay of 2 s.
The 2D-HMBC spectra are recorded with a J value of 4 Hz, spectral width of 300 ppm in F1 dimension, a relaxation delay of 1.5 s; data matrices of 1024 x 256 points (eight scans) were zero filled in F1 dimension to 1024 points.
JH–N long range were recorded by means of 2D-J-HMBC experiment of samples in CDCl3 solution, with the following parameters: relaxation delay 4.0 s, scaling factor SF = 23, J = 4 Hz, 16 scans.
In all experiments nitromethane was used as reference of 15N chemical shifts (δ = 0 ppm).
ACKNOWLEDGEMENTS
Thanks are due to PUR for financial support.
References
1. M. Witanowsky and L. Stefaniak, Annu. Rep. NMR Spectrosc., 1986, 18, 1. CrossRef
2. (a) L. Stefaniak, J. D. Roberts, M. Witanowsky, and G. A. Webb, Org. Magn. Reson., 1984, 22, 215; CrossRef (b) T. Axenrod, C. M. Watnick, and M. J. Wieder, Org. Magn. Reson., 1979, 12, 476. CrossRef
3. (a) R. M. Claramunt, D. Sanz, C. López, J. A. Jiménez, M. L. Jimeno, J. Elguero, and A. Fruchier, Magn. Res. Chem., 1997, 35, 35; CrossRef (b) R. M. Claramunt, C. López, M. D. Santa María, D. Sanz, and J. Elguero, Prog. NMR Spectrosc., 2006, 49, 169. CrossRef
4. (a) J. Elguero, R. Faure, and J. Llinares, Spectrosc. Lett., 1987, 20, 149; CrossRef (b) I. Alkorta, J. Elguero, A. Fruchier, N. Jagerovic, and G. P. A. Yap, J. Mol. Struct., 2004, 689, 251. CrossRef
5. L. De Benassuti, T. Recca, and G. Molteni, Tetrahedron, 2007, 63, 3302. CrossRef
6. J. A. Vida, 'Medicinal Chemistry'; ed. by M. W. Wolf and A. Burger, Wiley, New York, 1981, p. 787.
7. The Merck Index, 12th edition, ed. by S. Budavari, Merck & Co., Whitehouse Station, New Jersey, 1996.
8. W. Fröstl and L. Maître, Pharmacopsych., 1989, 22, 54. CrossRef
9. G. Molteni, ARKIVOC, 2007, (ii), 224.
10. G. Molteni, Heterocycles, 2006, 65, 2513. CrossRef
11. (a) Compounds 1a,b: G. Broggini, G. Casalone, L. Garanti, G. Molteni, T. Pilati, and G. Zecchi, Tetrahedron: Asymmetry, 1999, 10, 4447; CrossRef (b) Compound 2a: G. Molteni, G. Broggini, and T. Pilati, Tetrahedron: Asymmetry, 2002, 13, 2491; CrossRef (c) Compound 5: G. Broggini, G. Molteni, and T. Pilati, Tetrahedron: Asymmetry, 2000, 11, 1975. CrossRef
12. (a) Compound 3: G. Broggini, L. Bruché, L. Garanti, and G. Zecchi, J. Chem Soc., Perkin Trans 1, 1994, 433; CrossRef (b) Compound 4: G. Broggini, L. Garanti, G. Molteni, and G. Zecchi, Heterocycles, 1994, 38, 1601. CrossRef
13. M. Witanowsky, Z. Biedrzycka, W. Sicninska, and Z. Grabowsky, J. Magn Reson., 1998, 131, 54. CrossRef
14. A. R. Katritzky and J. M. Lagowski, 'Comprehensive Heterocyclic Chemistry', ed. by K. T. Potts, Pergamon, Oxford, 1985, Vol. 5, Chapter 4.01, p 1.
15. C. Gatti, A. Ponti, A. Gamba, and G. Pagani, J. Am. Chem. Soc., 1992, 114, 8634. CrossRef
16. K. Wüthrich, 'NMR of Proteins and Nucleic Acids', Wiley-Interscience, New York, 1986, p. 167.