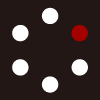
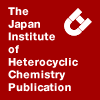
HETEROCYCLES
An International Journal for Reviews and Communications in Heterocyclic ChemistryWeb Edition ISSN: 1881-0942
Published online by The Japan Institute of Heterocyclic Chemistry
e-Journal
Full Text HTML
Received, 9th October, 2009, Accepted, 26th November, 2009, Published online, 26th November, 2009.
DOI: 10.3987/COM-09-11854
■ A [2]Rotaxane Containing N,N-Dialkylammonium Ion and N-Alkylaniline Centers. Translational Isomerism, and Specific N-Acylations
Yuji Tokunaga,* Masanori Kawabata, Yuji Yamauchi, and Naoki Harada
Department of Materials Science and Engineering, Faculty of Engineering, Fukui University, Bunkyo, Fukui 910-8507, Japan
Abstract
This paper describes the molecular shuttling and specific N-acylations of a [2]rotaxane featuring an encircling crown ether unit and both N-alkylaniline and N,N-dialkylamine centers on its dumbbell-shaped component. The crown binds predominantly to the dialkylammonium center under neutral conditions. Reactions with acid anhydride in nonpolar solvents and in the absence or presence of a weak base resulted in the N-acylations occurring mainly at the aniline moiety, and selective N-acylations of the dialkylamine unit occurred in polar solvents in the presence of strong bases.Rotaxanes are stabilized through attractive noncovalent interactions between their axle-like units and the cavities of their wheel-shaped components.1–4 For example, Takata, Kihara, and co-workers reported that the acidities of N,N-dialkylammonium groups in rotaxane systems featuring crown ether units are unusually low—because of stabilizing hydrogen bonds between the components—and that the N-acylation of these NH2+ centers proceeds slowly in the presence of an excess of a tertiary amine.4
Recently, the Loeb group investigated the use of N-alkylanilinium cations as suitable axle components for the formation of [2]pseudorotaxanes with crown ethers.5 They found that the introduction of electron-withdrawing groups on the anilinium benzene ring enhanced [2]pseudorotaxane formation. Because such [2]pseudorotaxanes are pH-sensitive, they prepared a molecular shuttle, driven by acid/base control, featuring a combination of anilinium and 1,2-bis(pyridinium)ethane recognition sites.6 These systems piqued our curiosity: If a [2]rotaxane contained both dialkylamine and alkylaniline moieties, which of these amino groups would predominantly recognize a crown ether through cooperative binding of a proton and which would react preferentially with electrophiles? We design the [2]rotaxane 1 (Scheme 1) to answer these questions (Figure 1).
The 1H NMR spectrum of 1,7 which possessed two different amino groups in the axle component and dibenzo[24]crown-8 (DB24C8) as a macrocyclic component, contains a concentration-independent NH2+ resonance at 7.52 ppm and signals for the pairs of two benzylic protons (Hd and He) at 4.42 and 4.56 ppm, all of which are characteristic of crown ether/dibenzylammonium-type [2]rotaxanes;4,7 in addition, the signal for the benzylic protons Hh appears at 4.24 ppm. Therefore, the N,N-dialkylammonium cationic center acted as the preferentially binding site under neutral conditions (Figure 2a).
An addition of trifluoroacetic acid (TFA) to the solution of the [2]rotaxane 1 in CDCl3 gave the anilinium salt 1-H+ and its translational isomer 2-H+ (Scheme 1). In the 1H NMR spectra of 2-H+ (Figure 2), the anilinium-NH proton resonates at 8.98 ppm and the signal for the anilinium benzylic protons Hh appears at 5.24 ppm; these signals are characteristic of crown ether/anilinium-type [2]rotaxanes.5,6 Additionally, the resonances for the benzylic protons Hd and He in 2-H+ appeared at significantly higher fields than they did in 1, attributable to the loss of deshielding effects associated with DB24C8; the resonances for both Hi and Hk moved to lower fields, a likely result of the formation of the anilinium ion. Shielding effects of DB24C8 are also responsible for the upfield shift in the resonance of methyl (Hj) protons in 2-H+. A 1H NMR spectroscopically monitored titration revealed the distribution of 1-H+/1 and 2-H+ (i.e., 1:0.5, 1:1, 1:2, and 1:5 molar mixtures of 1 and TFA gave 90:10, 82:18, 70:30, and 60:40 mixtures, respectively, of 1-H+/1 and 2-H+). These values suggest that the strengths of the association events for the two ammonium ions with DB24C8 were similar, with the higher basicity of the dialkylamine unit, relative to that of the N-alkylaniline unit, favoring its status as the dominant translational isomer under each set of conditions.
Recently, pH-responsive rotaxanes consisting of ammonium ions and crown ethers have been reported by several groups.6,8 In the most usual cases, deprotonation of the ammonium ion parts weakens the hydrogen-bond between the both components, and molecular shuttle driven by acid-base can be accomplished. In the present study, the positional discrimination is not enough, however we have shown the protonation-controlled translational isomerization using dialkylammonium and alkylanilinium stations.9
Next, we investigated the reactivities of the two nitrogen atoms in the [2]rotaxane toward acid anhydrides (Scheme 2). Treatment of 1 with acetic anhydride in ClCH2CH2Cl in the absence of base gave the N-acylaniline product 3a selectively (Table 1, run 1). This acylation was promoted by the addition of triethylamine (run 2), an excess of which also provided some of the N-acetyldialkylamine product 4a (run 3). Use of polar solvent and/or a strong base was necessary to achieve the selective production of 4a (runs 4–8). We observed similar selectivities for the N-acylations performed using di-tert-butyl dicarbonate (Boc2O) as the electrophile (Table 2): the N-Bocaniline derivative 3b was formed primarily in a nonpolar solvent and in the presence of weak base (runs 1–3), whereas a strong base and a polar solvent increased the production of 4b (runs 4–6). Long reaction times produced some unidentified products, but the addition of 2,6-di-tert-butylphenol improved the yield of 3b (run 7), presumably because it promoted the protonation of the dialkylamine unit or acted as a radical scavenger.
How do the base and solvent dramatically affect the selectivity? The acidity of N,N-dibenzylammonium NH2+ protons located within the cavity of a crown ether is quite low;4,8b therefore, triethylamine (pKa of Et3N+H = 10.8) cannot deprotonate 1 and the crown ether unit kinetically protects the dialkylamine moiety in the form of a dialkylammonium/crown ether–coordinated [2]rotaxane. In contrast, strong bases (pKa of t-BuOH = 18.0; pKa of ArOH = 16.8) deprotonated the dialkylammonium center, causing the DB24C8 unit to interact only weakly with the amino groups; as a result, the more reactive dialkylamine in the deprotonated [2]rotaxane attacked the electrophiles selectively (Figure 3). A polar solvent plays a similar role, cleaving the hydrogen bonds between the axle- and wheel-like components of the [2]rotaxane 1.
In summary, we have investigated the reactivity of two different amines of a [2]rotaxane possessing both N,N-dialkylamine and N-alkylaniline moieties. Under neutral conditions, the dialkylamine unit predominantly recognized the crown ether component in 1 through cooperative binding of a proton; addition of an acid generated the translational isomer 2. Specific N-acylations of 1 are possible in the presence of acetic anhydride and di-tert-butyl dicarbonate; these reactions are influenced by the polarity of the solvent and the strength of any added base. Recently, quantitative active transport of a [2]rotaxane comprising a secondary ammonium ion axle and a crown ether was examined using a one-shot acylation approach.10 Our results suggest the possibility of new transporting methodologies using such systems.
ACKNOWLEDGEMENTS
We thank Dr T. Hoshi of Tohoku University for performing the spectroscopic measurements. This study was supported by University of Fukui.
References
1. M. J. Frampton and H. L. Anderson, Angew. Chem. Int. Ed., 2007, 46, 1028. CrossRef
2. For reviews on rotaxanes, see: a) J.-P. Sauvage and C. Dietrich-Buchecker, 'Molecular Catenanes, Rotaxanes and Knots, Wiley-VCH, Weinheim, 1999; b) V. Balzai, A. Credi, F. M. Raymo, and J. F. Stoddart, Angew. Chem. Int. Ed., 2000, 39, 3348; CrossRef c) R. Ballardini, V. Balzani, A. Credi, M. T. Gandolfi, and M. Venturi, Acc. Chem. Res., 2001, 34, 445; CrossRef d) A. Harada, Acc. Chem. Res., 2001, 34, 456; CrossRef e) C. A. Schalley, K. Beizai, and F. Vögtle, Acc. Chem. Res., 2001, 34, 465; CrossRef f) N. Yui and T. Ooya, Chem. Eur. J., 2006, 12, 6730; CrossRef g) E. R. Kay, D. A. Leigh, and F. Zerbetto, Angew. Chem. Int. Ed., 2007, 46, 72. CrossRef
3. For examples of the stabilization of rotaxanes, see: a) E. Cordova, R. A. Bissell, and A. E. Kaifer, J. Org. Chem., 1995, 60, 1033; CrossRef b) A. H. Parham, B. Windisch, and F. Vögtle, Eur. J. Org. Chem., 1999, 1233; CrossRef c) K. Yoshida, T. Shimomura, K. Ito, and R. Hayakawa, Langmuir, 1999, 15, 910; CrossRef d) M. R. Craig, M. G. Hutchings, T. D. W. Claridge, and H. L. Anderson, Angew. Chem. Int. Ed., 2001, 40, 1071; CrossRef e) J. E. H. Buston, F. Marken, and H. L. Anderson, Chem. Commun., 2001, 1046; CrossRef f) A. Credi, S. Dumas, S. Silvi, M. Venturi, A. Arduini, A. Pochini, and A. Secchi, J. Org. Chem., 2004, 69, 5881; CrossRef g) H. Murakami, A. Kawabuchi, R. Matsumoto, T. Ido, and N. Nakashima, J. Am. Chem. Soc., 2005, 127, 15891; CrossRef h) P. Franchi, M. Fani, E. Mezzina, and M. Lucarini, Org. Lett., 2008, 10, 1901. CrossRef
4. a) N. Kihara, Y. Tachibana, H. Kawasaki, and T. Takata, Chem. Lett., 2000, 506; CrossRef b) Y. Tachibana, H. Kawasaki, N. Kihara, and T. Takata, J. Org. Chem., 2006, 71, 5093. CrossRef
5. S. J. Loeb, J. Tiburcio, and S. J. Vella, Org. Lett., 2005, 7, 4923. CrossRef
6. S. J. Vella, J. Tiburcio, and S. J. Loeb, Chem. Commun., 2007, 4752. CrossRef
7. Rotaxane 1 was prepared using modified our procedure: Y. Tokunaga, S. Nakashima, T. Iwamoto, K. Gambayashi, K. Hisada, and T. Hoshi, Heterocycles, 2010, 80, in press. CrossRef
8. a) P. R. Ashton, R. Ballardini, V. Balzani, I. Baxter, A. Credi, M. C. T. Fyfe, M. T. Gandolfi, M. Gómez-López, M.-V. Martínez-Díaz, A. Piersanti, N. Spencer, J. F. Stoddart, M. Venturi, A. J. P. White, and D. J. Williams, J. Am. Chem. Soc., 1998, 120, 11932; CrossRef b) Y. Tokunaga, T. Nakamura, M. Yoshioka, and Y. Shimomura, Tetrahedron Lett., 2006, 47, 5901; CrossRef c) D. A. Leigh and A. R. Thomson, Org. Lett., 2006, 8, 5377; CrossRef d) C.-L. Lin, C.-C. Lai, Y.-H. Liu, S.-M. Peng, and S.-H. Chiu, Chem. Eur. J., 2007, 13, 4350; CrossRef e) F. Coutrot and E. Busseron, Chem. Eur. J., 2008, 14, 4784; CrossRef f) K, C.-F. Leung, C.-P. Chak, C.-M. Lo, W.-Y. Wong, S. Xuan, and C. H. K. Cheng, Chem. Asian J., 2009, 4, 364.
9. Recently protonation-controlled molecular shuttle was reported: D. A. Leigh, A. R. Thomson, Tetrahedron, 2008, 64, 8411. CrossRef
10. Y. Makita, N. Kihara, and T. Takata, J. Org. Chem., 2008, 73, 9245. CrossRef