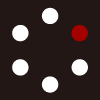
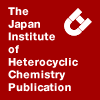
HETEROCYCLES
An International Journal for Reviews and Communications in Heterocyclic ChemistryWeb Edition ISSN: 1881-0942
Published online by The Japan Institute of Heterocyclic Chemistry
e-Journal
Full Text HTML
Received, 23rd October, 2009, Accepted, 30th November, 2009, Published online, 1st December, 2009.
DOI: 10.3987/COM-09-11859
■ S-Methylation of N-Containing Heterocyclic Thiols with Conjugated Acids of Methoxy Groups
Masao Shimizu,* Teruaki Shimazaki, Yoshihiro Kon, and Takeo Konakahara
Research Institute for Innovation in Sustainable Chemistry, National Institute of Advanced Industrial Science and Technology, 1-1-1 Higashi, Tsukuba, Ibaraki 305-8565 Tsukuba, Japan
Abstract
2-Mercaptopyridine-3-carboxylic acid reacts with methanol under acidic conditions to afford the corresponding S-methylated methyl ester, methyl 2-methylthiopyridine-2-carboxylate. Such S-methylation occurred for various N-containing heterocyclic thiols with acidic methanol. The reaction proceeded by the attack of mercapto groups on the conjugated acid of methanol. Therefore, the sulfur atom of pyridine-2-thiol was also methylated with acidic methyl ethers or methyl esters.A convenient method for esterification of carboxylic acids is heating them in alcoholic solvent in the presence of sulfuric acid.1 Methyl thiosalicylate, for example, is synthesized by heating thiosalicylic acid in methanol in the presence of sulfuric acid.2 In the same way, it was reported that methyl 2-mercaptopyridine-3-carboxylate, an aza-analogue of methyl thiosalicylate, is prepared from 2-mercaptopyridine-3-carboxylic acid (1).3 However, this esterification was unexpectedly troublesome according to the later report;4 N-methylation occurred under these conditions to afford 3-methoxycarbonyl-1-methyl-2-pyridinethione. The N-methylation usually occurs under basic conditions with methylating reagents such as methyl halides or dimethyl sulfates.5 Therefore, we were interested in the mechanism for this type of methylation of 1 with methanol under acidic conditions.
We first re-examined esterification of 1. When 1 was refluxed in methanol for 8 h in the presence of sulfuric acid, a product that had two methyl groups, according to 1H and 13C NMR spectra (at δ 2.54 and 3.94 ppm in 1H NMR, δ 14.0 and 52.4 ppm in 13C NMR), was obtained as the main product, and methyl 2-mercaptopyridine-3-carboxylate was not isolated. If this main product was the N-alkylated product, 3-methoxycarbonyl-1-methyl-2-pyridinethione, as reported in the literature,4 the 1H NMR peak at δ 2.54 ppm, assignable to an N-methyl group, appeared rather high upfield. By comparing spectral data of N-methyl and S-methyl pyridinethiol derivatives reported in the literature,6 we deduced the structure of the product as methyl 2-methylthiopyridine-3-carboxylate (2). S-Methylation of 1 occurred in acidic methanol. It was reported that the methyl group on methyl 2-mercaptobenzoate migrates to the mercapto group to form (2-methylthio)benzoic acid.7 Therefore, it is probable that 2 was produced by esterification of 2-methylthiopyridine-3-carboxylic acid which was formed by migration of the methyl group on methyl 2-mercaptopyridine-3-carboxylate. However, the reaction of 6-mercaptopyridine-3-carboxylic acid (3) with sulfuric acid in methanol refluxing for 8 h gave methyl 6-methylthiopyridine-3-carboxylate (4) in 68% yield. This result showed that the carboxyl group neighboring the thiol group did not affect methylation of the mercapto group. These methylations were then applied to pyridine-2-thiol (5), which had no carboxylic acid group.
Pyridine-2-thiol (5) was refluxed in methanol for 8 h in the presence of sulfuric acid. As a result, 2-methylthiopyridine (6, 62%) and 2,2’-dipyridyl sulfide (7, 2%) were isolated from the reaction mixture. Methylation certainly occurred to the sulfur atom. We tried methylation with various acids (Table 1). Methanol in the presence of sulfonic acids methylated 5 (Entries 3 and 4). Hydrochloric acid or a Lewis acid can be substituted for sulfuric acid (Entries 5 and 6); however, acetic acid did not work for this methylation (Entry 7). The formation of 6 in good yield required an excess amount of strong acid.
Other heterocyclic thiols, such as quinoline (8), benzimidazole (10), imidazole (12), and thiazoline derivatives (14), were treated as substrates with acidic methanol under the same reaction conditions for pyridinethiol methylation: the corresponding methylated products were obtained (Table 2). On the contrary, the sulfur atom of 4-t-butylbenzenethiol was not methylated under these conditions, and the starting material was recovered.
Acidic primary alcohol alkylation of 58 or mercapto azoles9 can be found in the literature, but no precise mechanism has been mentioned. The above results were used to formulate the reaction mechanism. Although it is possible that protonated methanol and dimethyl sulfate generated from the reaction of sulfuric acid with methanol were the active electrophilic species, the former is suitable as electrophiles because hydrochloric acid or a Lewis acid could be used in place of sulfuric acid. When pyridine-2-thiol 5 was used as a substrate, the pyridine ring was predominantly protonated, but sufficient nucleophilicity remained on the mercapto group. Actually, methylation of pyridine-2-thiol 5 with iodomethane also occurred under acidic conditions; in the presence of sulfuric acid in acetone and with heating at 80 oC (in a sealed tube) for 5 h, 6 was obtained in 54% yield.10 In this way, protonated 5 can react with electrophiles to produce 2-methylthiopyridine 6.
Although alkyloxonium cations that formed from alcohols under acidic conditions operate as alkylating agents in symmetrical ether or during alkyl halide synthesis,11 these cationic species have been applied only infrequently to other syntheses. Nucleophilic substitution in general was not carried out under acidic conditions, due to deactivation of the nucleophilic center by protonation. Although alcohols, such as 2-methyl-2-propanol, that generate stable carbocation species by dehydration under acidic conditions, can react with thiols to yield alkylthio derivatives,12 there appeared to be another reaction mechanism. It seemed that methylation of heterocyclic thiols by acidic methanol proceeded by an SN2cA mechanism (Scheme 2).
Reasoning from the above reaction mechanism, it is possible that an acidic methoxy group could work as a methylation reagent for heterocyclic thiols. Therefore, we tested the reaction using ethers and esters as electrophiles instead of methanol. Various kinds of methyl ethers and methyl esters were used for reagents in the presence of methanesulfonic acid (Table 3). Since methyl esters are in general more difficult to protonate than methanol,13 the yields of 6 were low (Entries 1 and 2). In contrast, 1,2-dimethoxymethane is easier to protonate than the other ethers,14 and methylation of 5 occurred in 58% yield (Entry 3). Although the yield of S-methylation using acidic methyl acetate was low (Entry 4), owing to difficulty of forming the corresponding conjugated acid,13 the reaction in dimethyl carbonate proceeded in 47% yield (Entry 5). The reaction with methyl methanesulfonate proceeded exceptionally well to afford 6 in good yield (Entry 6).
In conclusion, S-methylation of nitrogen containing heterocyclic thiols proceeds with methanol under acidic conditions. We explain the reaction mechanism as a nucleophilic attack of heterocyclic mercapto groups on the conjugated acid of methanol. This alkylation is applicable to methyl ethers and methyl esters.
EXPERIMENTAL
Melting points were determined on a Mettler FP90 microscopic plate and are uncorrected. 1H and 13C NMR spectra were obtained with a JEOL LA-500 spectrometer, and chemical shifts (δ) are reported in parts per million relative to internal tetramethylsilane and CDCl3, respectively. IR spectra were recorded on a JASCO FT IR-4100 spectrophotometer. Gas chromatographic analyses were performed on Shimadzu GC-14A chromatograph fitted with a Neutrabond-1 column. Silica gel column chromatography was carried out on Merck silica gel 60 (0.063–0.200 mm). Elemental analysis was performed by the Analytical Center at the National Institute of Advanced Industrial Science and Technology.
Typical procedure for the esterification of mercaptopyridine-3-carboxylic acids
To a solution of a mercaptopyridine-3-carboxylic acid (1 or 3, 500 mg, 3.2 mmol) in MeOH (25 mL) was added H2SO4 (98%, 0.5 mL). After reflux for 8 h, aqueous sodium carbonate was added and the product was extracted with CH2Cl2. The organic layer was washed with water and dried over MgSO4. Crude product was obtained after evaporating solvent. Products were isolated by silica gel column chromatography with CH2Cl2: AcOEt (20 : 1) mixture as an eluent.
Methyl 2-methylthiopyridine-3-carboxylate6 (2). Mp 59.0 - 59.3 °C (hexane); 1H NMR (CDCl3, 500 MHz) δ 2.54 (3H, s), 3.94 (3H, s), 7.05 (1H, dd, J = 7.7, 4.7 Hz), 8.21 (1H, dd, J = 7.7, 1.8 Hz), 8.59 (1H, dd, J = 4.7, 1.8 Hz); 13C NMR (CDCl3, 125 MHz) δ 14.0, 52.4, 118.0, 123.0, 138.8, 152.1, 163.0, 165.9; IR (KBr): νmax 1715, 1556, 1397, 1278, 1243, 1130, 1069, 770 cm-1; Anal. Calcd for C8H9NO2S: C, 52.44; H, 4.95; N, 7.64. Found: C, 52.61; H, 4.87; N, 7.57.
Methyl 6-methylthiopyridine-3-carboxylate15 (4). Mp 56.7 - 57.3 °C (hexane); 1H NMR (CDCl3, 500 MHz) δ 2.61 (3H, s), 3.93 (3H, s), 7.23 (1H, dd, J = 8.5, 0.8 Hz), 8.04 (1H, dd, J = 8.5, 2.2 Hz), 9.02 (1H, dd, J = 2.2, 0.8 Hz); 13C NMR (CDCl3, 125 MHz) δ 13.2, 52.2, 120.7, 121.4, 136.2, 150.7, 165.7, 166.0. IR (KBr): νmax 1715, 1590, 1434, 1364, 1292, 1122, 767 cm-1.
Typical procedure for the alkylation of heterocyclic thiols (5, 8, 10, 12, 14)
To a solution of thiol (2.7 mmol) in MeOH (30 mL) was added H2SO4 (98%, 0.3 mL, 5.5 mmol). After reflux for 8 h, aqueous sodium carbonate was added and the product was extracted with CH2Cl2. The organic layer was washed with water and dried over MgSO4. Crude product was obtained after evaporating solvent. A product was isolated by silica gel column chromatography with CH2Cl2: AcOEt (100 : 1) mixture as an eluent.
2-Methylthiopyridine (6). Bp 106 °C (4.0 kPa) [lit.,16 94 – 95 °C (4.0 kPa)]; 1H NMR (500 MHz, CDCl3) δ 2.57 (3H, s), 6.97 (1H, ddd, J = 7.3, 4.9, 1.0 Hz), 7.18 (1H, dt, J = 8.2, 0.9 Hz), 7.48 (1H, ddd, J = 8.2, 7.3, 1.8 Hz), 8.44 (1H, ddd, J = 4.9, 1.8, 0.9 Hz); 13C NMR (125 MHz, CDCl3) δ 13.3, 119.2, 121.6, 135.8, 149.5, 160.1; IR (neat): νmax 1581, 1556, 1455, 1414, 1126, 756, 733 cm-1.
2,2’-Dipyridyl sulfide (7). Bp 192 °C (120 Pa) [lit.,17 148 °C (133 Pa)]; 1H NMR (500 MHz, CDCl3) δ 7.17 (2H, ddd, J = 7.6, 4.9, 0.9 Hz), 7.45 (2H, dt, J = 7.7, 0.9 Hz), 7.62 (2H, td, J = 7.7, 1.9 Hz), 8.54 (2H, ddd, J = 4.9, 1.9, 0.9 Hz); 13C NMR (125 MHz, CDCl3) δ 121.8, 125.9, 137.1, 150.2, 156.8; IR (neat): νmax 1573, 1559, 1448, 1417, 1113, 758 cm-1.
2-Methylthioquinoline (9). Bp 170 °C (227 Pa) [lit.,18 115 – 117 °C (200 Pa)]; 1H NMR (500 MHz, CDCl3) δ 2.71 (3H, s), 7.23 (1H, d, J = 8.5 Hz), 7.42 (1H, ddd, J = 7.9, 7.0, 1.2 Hz), 7.65 (1H, ddd, J = 8.5, 7.0, 1.5 Hz), 7.72 (1H, dd, J = 7.9, 0.9 Hz), 7.88 (1H, d, J = 8.6 Hz), 7.95 (1H, d, J = 8.6 Hz); 13C NMR (125 MHz, CDCl3) δ 12.9, 120.7, 125.2, 125.9, 127.6, 128.0, 129.6, 135.2, 148.4, 159.9; IR (neat): νmax 1612, 1594, 1556, 1497, 1419, 1292, 1138, 1091, 815, 779, 748 cm-1.
2-Methylthiobenzimidazole (11). Mp 203.0 - 204.0 °C (AcOEt) [lit.,19 201 °C (AcOEt)]; 1H NMR (500 MHz, CDCl3) δ 2.72 (3H, s), 7.17 (2H, dd, J = 3.0, 1.5 Hz), 7.44-7.46 (2H, m); 13C NMR (125 MHz, CDCl3) δ 14.5, 54.1, 122.6, 139.6, 152.8; IR (KBr): νmax 1441, 1421, 1394, 1361, 1268, 1221, 986, 740 cm-1.
1-Methyl-2-methylthioimidazole (13). Bp 166 °C (4.0 kPa) [lit.,20 124 – 125 °C (4.0 kPa)]; 1H NMR (500 MHz, CDCl3) δ 2.59 (3H, s), 3.59 (3H, s), 6.91 (1H, d, J = 1.2 Hz), 7.04 (1H, d, J = 1.2 Hz); 13C NMR (125 MHz, CDCl3) δ 16.2, 33.0, 122.0, 129.1, 143.1; IR (neat): νmax 3388, 1462, 1416, 1279, 1125, 741, 685 cm-1.
2-Methylthio-2-thiazoline (15). Bp 174 °C (4.0 kPa) [lit.,21 70 – 71 °C (133 Pa)]; 1H NMR (CDCl3, 500 MHz) δ 2.55 (3H, s), 3.41 (2H, t, J = 7.9 Hz), 4.22 (2H, t, J = 7.9 Hz); 13C NMR (CDCl3, 125 MHz) δ 15.5, 35.8, 64.4, 166.6; IR (neat) νmax 1569, 1000, 979, 958, 917 cm-1.
Typical procedure for the methylation of pyridine-2-thiol with ethers or esters.
To a solution of pyridine-2-thiol (5, 111 mg, 1.0 mmol) in solvent (5 mL) was added methanesulfonic acid (2.0 mmol). After reflux for 5 – 11 h, aqueous sodium carbonate was added and the product was extracted with CH2Cl2. The organic layer was washed with water and dried over MgSO4. Yields of the products were determined by an internal standard technique using the GC method.
References
1. For examples, L. F. Fieser and M. Fieser, “Reagents for Organic Synthesis,” Wiley, New York, 1967, p. 678.
2. F. J. Baerlocher, M. O. Baerlocher, R. F. Langler, S. L. MacQuarrie, and M. E. Marchand, Aust. J. Chem., 2000, 53, 1; CrossRef E. Matsumura, T. Nishinaka, H. Tsujibo, H. Hachiken, Y. Miki, Y. Sakagami, and Y. Inamori, Biol. Pharm. Bull., 2000, 23. 254.
3. L. R. Fibel and P. E. Spoerri, J. Am. Chem. Soc., 1948, 70, 3908. CrossRef
4. L. Katz, W. Schroeder, and M. Cohen, J. Org. Chem., 1954, 19, 711. CrossRef
5. A. Schöberl and A. Wagner, Houben-Weyl, 4th ed., Vol. 9, ed. by E. Müller, Thieme, Stuttgart, 1955, p. 93.
6. M. Gelbcke, R. Grimée, R. Lejeune, L. Thunus, and J. V. Dejardin, Bull. Soc. Chim. Belg., 1983, 92, 39.
7. J. C. Grivas and K. C. Navada, J. Org. Chem., 1971, 36 1520. CrossRef
8. Courtot and Zwilling, In Chem. et Ind. Sonderband 18. Congr. Chim. ind., Nancy, 1938, p. 796 (Beilstein, 4th ed, Vol. 21, p. 374).
9. N. D. Abramova, G. G. Skvortsova, and B. V. Trzhtsinskaya, Zh. Org. Khim., 1984, 20, 2031; N. D. Abramova, G. G. Skvortsova, and B. V. Trzhtsinskaya, J. Org. Chem. USSR, 1984, 20, 1854.
10. Under the same reaction conditions, treatment of 4-t-butylbenzenethiol with iodomethane afforded 4-t-butylphenyl methyl sulfide in 1% yield and bis(4-t-butylphenyl) disulfide in 80% yield..
11. G. W. Brown, “The Chemistry of the Hydroxyl Group,” ed. by S. Patai, Wiley, London, 1971, p. 593; H. Feuer and J. Hooz, “The Chemistry of the Ether Linkage,” ed. by S. Patai, Wiley, London, 1967, p.445.
12. For examples: A. Courtin, H.-R. Tobel, and G. Auerbach, Helv. Chim. Acta, 1980, 63, 1412; CrossRef T. Schaefer and G. H. Penner, Can. J. Chem., 1988, 66, 1641; CrossRef N. C. Cutress, T. B. Grindley, A. R. Katritzky, and R. D. Topsom, J. Chem. Soc., Perkin Trans. 2, 1974, 263. CrossRef
13. G. A. Olah, A. M. White, and D. H. O’Brien, Chem. Rev., 1970, 70, 561. CrossRef
14. O. Tsuge and M. Noguchi, Chem. Lett., 1977, 749. CrossRef
15. M. Gelbcke, R. Grimée, R. Lejeune, and L. Thunus, Spectrochim. Acta, 1985, 41A, 567. CrossRef
16. I. B. Cook, Aust. J. Chem., 1989, 42, 1493. CrossRef
17. Monsanto Co., FR Patent, 1,503,307 (1967) (Chem. Abstr., 1969, 70, 3549).
18. A. Maslankiewicz, Pol. J. Chem., 1980, 54, 2069.
19. E. Hoggarth, J. Chem. Soc., 1949, 3311. CrossRef
20. M. Nishida, H. Kimoto, S. Fujii, Y. Hayakawa, and L. A. Kohen, Bull. Chem. Soc. Jpn., 1991, 64, 2255. CrossRef
21. A. K. Bose, J. L. Fahey, and M. S. Manhas, J. Heterocycl. Chem., 1973, 10, 791. CrossRef