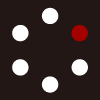
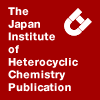
HETEROCYCLES
An International Journal for Reviews and Communications in Heterocyclic ChemistryWeb Edition ISSN: 1881-0942
Published online by The Japan Institute of Heterocyclic Chemistry
e-Journal
Full Text HTML
Received, 24th October, 2009, Accepted, 25th December, 2009, Published online, 29th December, 2009.
DOI: 10.3987/COM-09-11862
■ Synthesis of Metal Complexes with 1-Substituted 3-Hydroxy-2(1H)-pyridinethiones and Their Insulin-Mimetic Activities
Akira Katoh,* Hiroko Yokoyama, Yuriko Matsumura, Yutaka Yoshikawa, Hiroyuki Yasui, and Hiromu Sakurai
Department of Materials and Life Science, Faculty of Science and Technology, Seikei University, Musashino-shi, Tokyo 180-8633, Japan
Abstract
Eight kinds of 1-alkyl-3-hydroxy-2(1H)-pyridinethiones were synthesized from a commercially available 3-methoxy-2(1H)-pyridinone via 3 steps. Zn(II) and vanadyl complexes were synthesized by treatment with Zn(OAc)2 or ZnSO4, and VOSO4, respectively. Vanadyl complexes were found to exist in VO(S2O2) coordination mode by means of ESR spectroscopy. From in vitro evaluation of the inhibitory effect on FFA release from rat adipocytes treated with epinephrine, it was found that IC50 values of Zn(II) complexes with 1-alkyl-3-hydroxy-2(1H)-pyridinethiones, regardless of the methylene-chain length at N-1 position, were in micromolar levels. In other words, these Zn(II) complexes showed higher insulin-mimetic activities than those synthesized previously. On the other hand, the insulin-mimetic activity of vanadyl complexes unfortunately could not be measured owing to their insolubility in KRB buffer which is used in vitro assay.INTRODUCTION
One of the most widespread lifestyle-related diseases in the 21st century is thought to be diabetes mellitus (DM). The number of patients that suffer from DM in the world has been projected to rise approximately 366 million by 2030 from 171 million in 2000.1 DM develops many secondary complications such as atherosclerosis, microangiopathy, renal dysfunction and failure, cardiac abnormalities, diabetic retinopathy, and other ocular disorders.
DM is generally classified into type 1 and type 2 DM. Type 1 DM is caused by destruction of pancreatic β-cells, and so it is characterized by a lack of insulin production. Type 2 DM is caused by aging, obesity, spiritual stress or other environmental factors including high cholesterol and high blood pressure, and so it is characterized by insulin resistance and abnormality of insulin-secretion.2 Type 1 DM can be only treated by daily hypodermic injections of insulin, and type 2 DM is treated by dietary measures, exercise and several types of synthetic therapeutic medicines. Both treatments have some defects that several insulin injections in a day have pain and stress for patients, and synthetic therapeutic medicines have severe side effects. Therefore, the development of orally active compounds in place of physically and mentally painful insulin injections for type 1 DM and of compounds without severe side effects for type 2 DM is still required not only to treat DM but also to improve the quality of life (QOL) in DM patients.
Several metal ions have been reported to show insulin-mimetic activity in vitro.3,4 Hg2+, Se4+, and Cd2+ strongly inhibited free fatty acid (FFA)-release, but these metal ions are highly toxic. Following these ions, V3+, V4+ (VO2+), Zn2+ and Mn2+ also inhibited FFA-release. Since V3+ is readily oxidized to V4+ and V5+ states at physiological pH and V4+ is less toxic than V5+, we exclusively used V4+ in our study. In addition to V4+, we extended our work to Zn2+.
Both metals in the form of inorganic salts such as VOSO4,5 ZnCl2, and ZnSO46-8 could decrease and normalize the blood glucose level in diabetic animals even by an oral dosage, but they are generally poorly absorbed from lipid bilayer cell membrane. High dosage is required to lower the blood glucose level, and it is responsible for emerging some undesirable side-effects. To overcome defects with respect to absorption and dosage, many researchers have focused on the design and synthesis of metal complexes with low-molecular-weight organic bidentate ligands. Zn(II) complexes such as O4,9-12 N4,13 N2O2,14,15 S2O2,16-18 and vanadyl complexes such as O4,9,19-23 N4,24,25 N2O2,26-29 S2O217,18,20,30 coordination modes have been reported to exhibit insulin-mimetic activities both in vitro and in vivo. Further it has been revealed that without exception, complexes with S2O2 coordination mode showed higher insulin-mimetic activities than those with O4 coordination mode.31
As an extensive studies on chemistry and biochemistry32 of insulin-mimetic vanadium and zinc complexes and with the objective of providing a new family of insulin-mimetic complexes with M(S2O2) (M=VO and Zn) coordination modes, we describe here synthesis of novel vanadyl and Zn(II) complexes with 3-hydroxy-2(1H)-pyridinethiones and their insulin-mimetic activities.
RESULTS AND DISCUSSION
Synthesis of metal complexes with 1-alkyl-3-hydroxy-2(1H)-pyridinethiones
A synthetic procedure for metal complexes with 1-alkyl-3-hydroxy-2(1H)-pyridinethiones is depicted in Scheme 1.
A commercially available 3-methoxy-2(1H)-pyridinone was treated with alkyl halides in dry MeOH in the presence of KOH to give 1-alkyl-3-methoxy-2(1H)-pyridinones 1a-h.33 The conversion of the carbonyl into the corresponding thiocarbonyl was carried out using Lawesson’s reagent to give the corresponding 1-alkyl-3-methoxy-2(1H)-pyridinethiones 2a-h. Compounds 2a-h were allowed to react with an equimolar amount of BBr3 to give 1-alkyl-3-hydroxy-2(1H)- pyridinethiones 3a-h.
Bidentate ligands 3a-d were treated with a 0.5 equimolar amount of Zn(OAc)212,34 in H2O:EtOH (1:1) mixture at room temperature to give Zn(II) complexes 4a-d. On the other hand, compounds 3e-h which have a long alkyl chain and a phenyl group were sparingly soluble in organic solvents, and thus the ZnSO4-LiOH method17 was employed. Bidentate ligands 3e-h were treated with ZnSO4·7H2O in the presence of LiOH in H2O:MeOH (1:1) mixture at room temperature to afford Zn(II) complexes 4e-h. The structural assignment of compounds 4a-h was carried out by 1H-NMR, IR and MALDI-TOF MS spectroscopies, and the combustion analysis. Each MALDI-TOF MS spectrum exhibited the peak related to the molecular ion ([M+H]+), strongly indicating the formation of the desired Zn(II) complex. Bidentate ligands 3a-g were treated with a 0.5 equimolar amount of VOSO4·H2O18,19 in H2O at pH 10 at room temperature for compounds 3a-c and with refluxing overnight for compounds 3d-g to give vanadyl complexes 5a-g. The structural assignment of compounds 5a-g was carried out by IR, UV-vis and MALDI-TOF MS spectroscopies, and the combustion analysis. On IR spectra, characteristic absorption bands owing to V=O stretching vibrations were observed around 970 cm-1. Each MALDI-TOF MS spectrum exhibited the peak related to the molecular ion ([M]+), strongly indicating the formation of the desired vanadyl complex.
Structural characteristics of ligands and metal complexes
The pKa value of compound 3b was measured in aqueous solution by the conventional pH titration method and was calculated to be 9.4. The pKa value of 3-hydroxy-2(1H)-pyridinethione 3b was larger than that of 3-hydroxy-2(1H)-pyridinone (pKa = 8.835). Considering the resonance effect, the contribution of –N+=C–S- in resonance hybrid should be larger than that of –N+=C–O-. In addition, higher pKa value may be attributable to an intramolecular hydrogen bonding between –O-H···-S–C=N+–.
It is recognized that the biological activity is affected by the degree of the hydrophobicity of compound. In fact, the partition coefficient is one of the most important factors on oral administration of drugs. Therefore, the partition coefficients of compounds 3a-h and Zn(II) complexes 4a-h were measured using two phases of an aqueous solution buffered at pH 7.4 and 1-octanol. The partition coefficients, logPow = log(Coctanol/Cbuffer), are summarized in Table 1.
Among a series of 1-alkyl-3-hydroxy-2(1H)-pyridinethiones 3a-e, an increase in the alkyl chain length and introduction of phenyl group at N-1 position result in an increase of the solubility in 1-octanol. Unfortunately, the partition coefficients of compounds 3f-h could not be measured because of insolubility in KRB buffer. The partition coefficient of Zn(II) complexes 4a-g also could not be measured, because they were practically insoluble in both solutions.
UV-vis spectra of vanadyl complexes 5a-g were measured in DMSO solution, and the results are summarized in Table 2. On the basis of small molar extinction coefficients (ε), the absorption bands of vanadyl complexes 5a-g around 540 nm can be attributed to d-d transition.
Vanadyl ion has an unpaired electron. ESR spectrum is useful tool for investigation of electronic structure of vanadyl complexes. ESR spectra of vanadyl complexes 5a-g were measured. When vanadyl is VO2+ state, the nuclear spin quantum number (I) of vanadium (IV) is 7/2. The number (n) of hyperfine signal of vanadium(IV) becomes eight according to the following equation; n = 2I + 1. Eight resonance line signals characteristic to VO2+ state were detected in DMSO at room and liquid nitrogen temperatures. ESR parameters such as universal constants (g-values) and hyperfine coupling constants (A-values) were calculated. (see Experimental section) The calculated g// and A// values are consistent with those of vanadyl complexes with S2O2 coordination mode36 reported previously.
Evaluation of insulin-mimetic activities of metal complexes
Previously, Sakurai and co-workers have demonstrated that the FFA release from rat adipocytes is a good in vitro evaluation system to find a compound exhibiting an insulin-mimetic action.5,37 The results on Zn(II) complexes are compiled in Figure 1, together with ZnSO4 as the positive control. As seen in Figure 1, all Zn(II) complexes 4a-h inhibited FFA release in a dose-dependent manner from the epinephrine-stimulated rat adipocytes.
IC50 values which are 50% inhibition concentrations of the FFA release level stimulated by epinephrine were estimated from these graphic data, and the results are summarized in Table 3. All Zn(II) complexes 4a-h showed the insulin-mimetic activity 15 times as much as ZnSO4 as the positive control.
Previously, it was found that the balance of the hydrophilicity/hydrophobicity is one of the most important factors exhibiting the insulin-mimetic activity.10 However, such balance was not observed in this experiment. In the case of vanadyl complexes 5a-g no activity was observed. It may be mainly responsible for the insolubility of vanadyl complexes in the KRB buffer.
In conclusion, Zn(II) and vanadyl complexes with eight kinds of 1-alkyl-3-hydroxy-2(1H)-pyridine- thiones were synthesized. On the basis of in vitro results, Zn(II) complexes 4a-h are expected to become potent insulinomimetic complexes for treating type 2 DM in animals.
EXPERIMENTAL
Melting points were measured on a MELTING POINT APPARATUS SMP3 in open capillaries and are uncorrected. IR and UV-vis spectra were recorded on a JASCO FT/IR-470 infrared and on a JASCO Ubest V-550 spectrophotometers. 1H-NMR spectra were obtained on a JEOL JIM-LA400D NMR spectrometer in an appropriate solvent. Chemical shifts are reported in ppm (δ) downfield from internal tetramethylsilane (TMS). Thin layer chromatography (TLC) was performed on silica gel 60 F254 with 0.2 mm thickness. Column chromatography was carried out with Merck Silica Gel 60 (63-210 mesh). The combustion analysis was performed on a PERKIN-ELMER 2400 CHNS/O analyzer series II. MALDI-TOF MS was performed on a AXIMA-LNR/-CFR/-CFR Plus (S/W Version 2.4).
General synthetic procedure for 1-alkyl-3-methoxy-2(1H)-pyridinones
A typical example: 3-methoxy- 1-methyl-2(1H)-pyridinone (1a). 3-Methoxy-2(1H)-pyridinone (2.5 g, 20 mmol) and KOH (1.5 g, 27 mmol) were dissolved in dry MeOH (125 mL) under Ar atmosphere. To the mixture was slowly added MeI (5.7 g; 2.5 mL, 40 mmol), and then the reaction mixture was refluxed for 24 h. After evaporation of the solvent, the residue was dissolved in H2O (50 mL), and extracted with CHCl3 (50 mL). The aqueous layer was further extracted with CHCl3 (50 mL x 3). The combined organic layer was dried over anhydrous Na2SO4. After evaporation of the solvent, the residue was purified by column chromatography on silica gel with CHCl3:MeOH (10:1) mixture as an eluant to give the product 1a as an orange oil. Yield: 3.12 g (quant.); IR (neat): 3074, 2938, 1654, 1599, 1557, 1457, 1375, 1254, 1034, 750 and 661 cm-1; 1H-NMR (δ, CDCl3, 400 MHz): 3.58 (3H, s), 3.82 (3H, s), 6.10 (1H, dd, J=6.9 and 7.3 Hz), 6.62 (1H, dd, J=1.5 and 7.3 Hz), and 6.91 ppm (1H, dd, J=1.5 and 6.9 Hz). Anal. Calcd for C7H9NO2·1.2H2O: C, 52.30; H, 7.15; N, 8.71%. Found: C, 52.12; H, 6.97; N, 8.59%.
3-Methoxy-1-ethyl-2(1H)-pyridinone (1b): as a yellow oil; yield: 62%; IR (neat): 3063, 2976, 1651, 1596, 1558, 1460, 1236, 1050, 752 and 706 cm-1; 1H-NMR (δ, CDCl3, 400 MHz): 1.35 (3H, t, J=7.3 Hz), 3.82 (3H, s), 4.25 (2H, q, J=7.3 Hz), 6.11 (1H, dd, J=7.1 and 7.3 Hz), 6.59 (1H, dd, J=1.7 and 7.3 Hz), and 6.90 ppm (1H, dd, J=1.7 and 7.1 Hz). Anal. Calcd for C8H11NO2·0.7H2O: C, 57.96; H, 7.54; N, 8.45%. Found: C, 58.04; H, 7.64; N, 8.13%.
3-Methoxy-1-propyl-2(1H)-pyridinone (1c): as an orange oil; yield: 76%; IR (neat): 3065, 2964, 1652, 1600, 1460, 1229, 1059, 751 and 702 cm-1; 1H-NMR (δ, CDCl3, 400 MHz): 0.95 (3H, t, J=7.5 Hz), 1.78 (2H, sext, J=7.3 Hz), 3.81 (3H, s), 3.94 (2H, t, J=7.3 Hz), 6.08 (1H, dd, J=6.8 and 7.3 Hz), 6.59 (1H, dd, J=1.7 and 7.3 Hz), and 6.87 ppm (1H, dd, J=1.7 and 6.8 Hz). Anal. Calcd for C9H13NO2·0.5H2O: C, 61.34; H, 8.01; N, 7.95%. Found: C, 61.30; H, 8.17; N, 7.85%.
3-Methoxy-1-butyl-2(1H)-pyridinone (1d): as an orange oil; yield: 69%; IR (neat): 2958, 2870, 1650, 1595, 1461, 1253, 1060, 751 and 700 cm-1; 1H-NMR (δ, CDCl3, 400 MHz): 0.94 (3H, t, J=7.3 Hz), 1.36 (2H, sext, J=7.6 Hz), 1.73 (2H, quint, J=7.6 Hz), 3.80 (3H, s), 3.97 (2H, t, J=7.3 Hz), 6.08 (1H, dd, J=6.9 and 7.4 Hz), 6.59 (1H, dd, J=1.5 and 7.4 Hz), and 6.87 ppm (1H, dd, J=1.5 and 6.9 Hz). Anal. Calcd for C10H15NO2·0.3H2O: C, 64.35; H, 8.42; N, 7.50%. Found: C, 64.21; H, 8.68; N, 7.44%.
3-Methoxy-1-benzyl-2(1H)-pyridinone (1e): as a yellow oil; yield: 81%; IR (neat): 3062, 2937, 1653, 1602, 1455, 1226, 1062, 747 and 699 cm-1; 1H-NMR (δ, CDCl3, 400 MHz): 3.80 (3H, s), 5.91 (2H, s), 6.08 (1H, dd, J=6.8 and 7.3 Hz), 6.59 (1H, dd, J=1.7 and 7.3 Hz), 6.89 (1H, dd, J=1.7 and 6.8 Hz), and 7.27-7.33 ppm (5H, m). Anal. Calcd for C13H13NO2·0.4H2O: C, 70.19; H, 6.25; N, 6.30%. Found: C, 70.20; H, 6.22; N, 6.17%.
3-Methoxy-1-phenethyl-2(1H)-pyridinone (1f): as an orange oil; yield: 85%; IR (neat): 3060, 2940, 1653, 1601, 1455, 1251, 1065, 749 and 701 cm-1; 1H-NMR (δ, CDCl3, 400 MHz): 3.06 (2H, t, J=7.2 Hz), 3.82 (3H, s), 4.18 (2H, t, J=7.2 Hz), 5.95 (1H, t, J=7.2 Hz), 6.55 (1H, dd, J=1.6 and 7.2 Hz), 6.58 (1H, dd, J=1.6 and 7.2 Hz), and 7.15-7.30 ppm (5H, m). Anal. Calcd for C14H15NO2·0.4H2O: C, 71.11; H, 6.73; N, 5.92%. Found: C, 70.92; H, 6.87; N, 5.98%.
3-Methoxy-1-phenylpropyl-2(1H)-pyridinone (1g): as an orange oil; yield: 75%; IR (neat): 3062, 2938, 1652, 1601, 1456, 1252, 1068, 748 and 700 cm-1; 1H-NMR (δ, CDCl3, 400 MHz): 2.10 (2H, quint, J=7.6 Hz), 2.68 (2H, t, J=7.8 Hz), 3.81 (3H, s), 3.99 (2H, t, J=7.3 Hz), 6.08 (1H, t, J=7.2 Hz), 6.59 (1H, dd, J=1.6 and 7.2 Hz), 6.82 (1H, dd, J=1.6 and 7.2 Hz), and 7.17-7.30 ppm (5H, m). Anal. Calcd for C15H17NO2: C, 74.05; H, 7.04; N, 5.76%. Found: C, 73.76; H, 7.19; N, 5.64%.
3-Methoxy-1-dodecyl-2(1H)-pyridinone (1h): as an orange oil; yield: 64%; IR (neat): 3056, 2938, 1650, 1610, 1542, 1445, 1260, 1057, 783 and 718 cm-1; 1H-NMR (δ, CDCl3, 400 MHz):0.88 (3H, t, J=7.3 Hz), 1.25 (20H, m), 3.81 (3H, s), 3.95 (3H, t, J=7.3 Hz), 6.09 (1H, dd, J=7.1 and 7.6 Hz), 6.58 (1H, dd, J=1.6 and 7.6 Hz), and 6.87 ppm (1H, dd, J=1.6 and 7.1 Hz). Anal. Calcd for C18H31NO2: C, 73.67; H, 10.65; N, 4.77%. Found: C, 73.66; H, 10.96; N, 4.91%.
General synthetic procedure for 1-alkyl-3-methoxy-2(1H)-pyridinethiones
A typical example: 3-methoxy-1-methyl-2(1H)-pyridinethione (2a). A solution of compound 1a (1.40 g, 10.1 mmol) and Lawesson’s reagent (2.24 g, 5.54 mmol) in toluene (100 mL) was refluxed for 5.5 h. After cooling to rt, the solvent was removed under reduced pressure. The residue was purified by column chromatography on silica gel with CHCl3:acetone:EtOH (100:5:1) mixture as an eluant to give the product 2a as yellow solids. Yield: 1.42 g (91%); mp 127.2-130.2 °C; IR (KBr): 3069, 2934, 1617, 1502, 1468, 1393, 1260 and 1057, 1137, 769 and 710 cm-1; 1H-NMR (δ, CDCl3, 400 MHz): 3.93 (3H, s), 4.07 (3H, s), 6.61 (1H, dd, J= 6.5 and 7.8 Hz), 6.71 (1H, dd, J=1.3 and 7.8 Hz), and 7.43 ppm (1H, dd, J=1.3 and 6.5 Hz). Anal. Calcd for C7H9NOS: C, 54.17; H, 5.84; N, 9.02%. Found: C, 54.10; H, 5.63; N, 8.72%.
3-Methoxy-1-ethyl-2(1H)-pyridinethione (2b): as yellow solids; yield: 68%; mp 140.0-141.2 °C; IR (KBr): 3016, 2969, 1616, 1539, 1444, 1188, 1045, 1135, 773 and 717 cm-1; 1H-NMR (δ, CDCl3, 400 MHz): 1.48 (3H, t, J=7.3 Hz), 3.92 (3H, s), 4.70 (2H, q, J=7.1 Hz), 6.63 (1H, dd, J=6.4 and 7.6 Hz), 6.68 (1H, dd, J=1.1 and 7.6 Hz), and 7.39 ppm (1H, dd, J=1.1 and 6.4 Hz). Anal. Calcd for C8H11NOS: C, 56.77; H, 6.55; N, 8.28%. Found: C, 56.50; H, 6.73; N, 8.14%.
3-Methoxy-1-propyl-2(1H)-pyridinethione (2c): as yellow solids; yield: 78%; mp 75.4-76.1 °C; IR (KBr): 3017, 2955, 1617, 1537, 1461, 1051, 1139, 781 and 718 cm-1; 1H-NMR (δ, CDCl3, 400 MHz): 1.00 (3H, t, J=7.3 Hz), 1.96 (2H, sext, J=7.6 Hz), 3.92 (3H, s), 4.58 (2H, t, J= 7.7 Hz), 6.60 (1H, dd, J=6.3 and 7.5 Hz), 6.68 (1H, dd, J=1.0 and 7.5 Hz), and 7.37 ppm (1H, dd, J=1.3 and 6.3 Hz). Anal. Calcd for C9H13NOS: C, 58.98; H, 7.51; N, 7.64%. Found: C, 58.96; H, 7.22; N, 7.56%.
3-Methoxy-1-butyl-2(1H)-pyridinethione (2d): as a brown oil; yield: 98%; IR (neat): 3052, 2956, 1617, 1540, 1451, 1181, 1052, 1135, 770 and 714 cm-1; 1H-NMR (δ, CDCl3, 400 MHz): 0.98 (3H, t, J=7.3 Hz), 1.42 (2H, sext, J=7.6 Hz), 1.90 (2H, quint, J=7.7 Hz), 3.92 (3H, s), 4.62 (2H, t, J=7.7 Hz), 6.60 (1H, dd, J=6.6 and 7.8 Hz), 6.67 (1H, dd, J=1.1 and 7.8 Hz), and 7.37 ppm (1H, dd, J=1.1 and 6.6 Hz). Anal. Calcd for C10H15NOS·0.5H2O: C, 58.22; H, 7.82; N, 6.79%. Found: C, 58.08; H, 7.85; N, 6.64%.
3-Methoxy-1-benzyl-2(1H)-pyridinethione (2e): as yellow solids; yield: 86%; mp 123.4-124.3 °C; IR (KBr): 3060, 2973, 1617, 1539, 1452, 1131, 1062, 770 and 695 cm-1; 1H-NMR (δ, CDCl3, 400 MHz): 3.94 (3H, s), 5.94 (2H, s), 6.58 (1H, dd, J=6.8 and 7.6 Hz), 6.68 (1H, dd, J= 1.0 and 7.6 Hz), 7.30-7.37 (5H, m), and 7.35 ppm (1H, dd, J=1.0 and 6.8 Hz). Anal. Calcd for C13H13NOS·0.1H2O: C, 66.98; H, 5.71; N, 6.01%. Found: C, 66.73; H, 5.57; N, 6.02%.
3-Methoxy-1-phenethyl-2(1H)-pyridinethione (2f): as yellow solids; yield 81%; mp 125 °C (decomp.); IR (KBr): 3073, 2993, 1617, 1539, 1450, 1134, 1048, 769 and 700 cm-1; 1H-NMR (δ, CDCl3, 400 MHz): 3.25 (2H, t, J=7.3 Hz), 3.94 (3H, s), 4.84 (2H, t, J=7.3 Hz), 6.49 (1H, t, J=7.0 Hz), 6.69 (1H, d, J=7.0 Hz), 7.08 (1H, d, J=7.0 Hz), and 7.20-7.31 ppm (5H, m). Anal. Calcd for C14H15NOS: C, 68.54; H, 6.16; N, 5.71%. Found: C, 68.66; H, 6.33; N, 5.68%.
3-Methoxy-1-phenylpropyl-2(1H)-pyridinethione (2g): as yellow solids; yield: 88%; mp 92.9-94.4 °C; IR (KBr): 3065, 2945, 1617, 1537, 1445, 1125, 1054, 786 and 698 cm-1; 1H-NMR (δ, CDCl3, 400 MHz): 2.27 (2H, quint, J=7.6 Hz), 2.74 (2H, t, J=7.6 Hz), 3.92 (3H, s), 4.63 (2H, t, J=7.6 Hz), 6.58 (1H, dd, J=6.6 and 7.8 Hz), 6.68 (1H, dd, J=1.0 and 7.8 Hz), and 7.19-7.32 ppm (6H, m). Anal. Calcd for C15H17NOS: C, 69.46; H, 6.61; N, 5.40%. Found: C, 69.63; H, 6.76; N, 5.37%.
Synthesis of 3-methoxy-1-dodecyl-2(1H)-pyridinethione (2h): as yellow amorphous solids; yield: 60%; IR (KBr): 3058, 2956, 1619, 1541, 1444, 1262, 1058, 1136, 777 and 717 cm-1; 1H-NMR (δ, CDCl3, 400 MHz):0.88 (3H, t, J=7.3 Hz), 1.25 (20H, m), 3.92 (3H, s), 4.60 (3H, t, J=7.3 Hz), 6.60 (1H, dd, J=7.1 and 7.6 Hz), 6.67 (1H, dd, J=1.6 and 7.6 Hz), and 7.36 ppm (1H, dd, J=1.6 and 7.1 Hz). Anal. Calcd for C18H31NOS: C, 69.85; H, 10.10; N, 4.53%. Found: C, 70.10; H, 10.25; N, 4.70%.
General synthetic procedure for 1-alkyl-3-hydroxy-2(1H)-pyridinethiones
A typical example: 3-hydroxy-1-methyl-2(1H)-pyridinethione (3a). To a solution of compound 2a (1.47 g, 9.47 mmol) in dry CH2Cl2 (115 mL) was slowly added 1M BBr3 in dry CH2Cl2 (9.5 mL) at -30 °C under Ar atmosphere. The reaction mixture was stirred for 48 h at rt. The mixture was again cooled to -30 °C, and then MeOH (38 mL) was slowly added to the mixture. After evaporation of the solvent, the residue was adjusted to pH 7 with 1M NaOH, and then extracted with CHCl3 (50 mL x 3). The combined organic layer was dried over anhydrous Na2SO4. After evaporation of the solvent, the residue was purified by column chromatography on silica gel (20 g) with CHCl3:acetone:EtOH (100:5:1) mixture as an eluant to give the product 3a as yellow solids. Yield: 1.10 g (82%); mp 117-119 °C; IR (KBr): 3113, 1623, 1550, 1472, 1351, 1420, 1283, 1132, 773 and 710 cm-1; 1H-NMR (δ, CDCl3, 400 MHz): 4.07 (3H, s), 6.65 (1H, dd, J=6.6 and 7.2 Hz), 7.00 (1H, dd, J=1.3 and 7.2 Hz), 7.37 (1H, dd, J=1.3 and 6.6 Hz), and 8.51 ppm (1H, s, D2O exchangeable). Anal. Calcd for C6H7NOS: C, 51.04; H, 5.00; N, 9.92%. Found: C, 51.28; H, 5.05; N, 9.81%.
3-Hydroxy-1-ethyl-2(1H)-pyridinethione (3b): as yellow solids; yield: 98%; mp 50.0-51.2 °C; IR (KBr): 3449, 3046, 2975, 1626, 1546, 1470, 1425, 1272, 1132, 770 and 713 cm-1; 1H-NMR (δ, CDCl3, 400 MHz): 1.52 (3H, t, J=7.1 Hz), 4.61 (2H, q, J=7.3 Hz), 6.68 (1H, dd, J=6.6 and 7.6 Hz), 6.98 (1H, dd, J=1.0 and 7.6 Hz), 7.35 (1H, dd, J=1.0 and 6.6 Hz), and 8.60 ppm (1H, s). Anal. Calcd for C7H9NOS: C, 54.17; H, 5.84; N, 9.02%. Found: C, 54.10; H, 5.64; N, 8.72%.
3-Hydroxy-1-propyl-2(1H)-pyridinethione (3c): as a yellow oil; yield: 93%; IR (neat): 3073, 2965, 2874, 1628, 1546, 1472, 1429, 1283, 1137, 770 and 713 cm-1; 1H-NMR (δ, CDCl3, 400 MHz): 1.02 (3H, t, J=7.4 Hz), 1.97 (2H, sext, J=7.6 Hz), 4.49 (2H, t, J=7.6 Hz), 6.65 (1H, dd, J=6.6 and 7.8 Hz), 6.98 (1H, dd, J=1.2 and 7.8 Hz), 7.32 (1H, dd, J=1.2 and 6.6 Hz), and 8.61 ppm (1H, s). Anal. Calcd for C8H11NOS: C, 56.77; H, 6.55; N, 8.28%. Found: C, 56.76; H, 6.78; N, 8.23%.
3-Hydroxy-1-butyl-2(1H)-pyridinethione (3d): as a brown oil; yield: 91%; IR (neat): 3073, 2958, 2932, 1628, 1545, 1472, 1429, 1283, 1138, 769 and 713 cm-1; 1H-NMR (δ, CDCl3, 400 MHz): 0.99 (3H, t, J=7.4 Hz), 1.43 (2H, sext, J=7.4 Hz), 1.92 (2H, quint, J=7.7 Hz), 4.53 (2H, t, J=7.7 Hz), 6.65 (1H, dd, J=6.6 and 7.6 Hz), 6.98 (1H, dd, J=1.4 and 7.6 Hz), 7.32 (1H, dd, J=1.4 and 6.6 Hz), and 8.62 ppm (1H, s). Anal. Calcd for C9H13NOS·0.1H2O: C, 58.41; H, 7.19; N, 7.57%. Found: C, 58.19; H, 7.19; N, 7.53%.
3-Hydroxy-1-benzyl-2(1H)-pyridinethione (3e): as yellow solids; yield: 92%; mp 90.8-92.0 °C; IR (KBr): 3463, 3049, 2960, 1622, 1544, 1468, 1431, 1287, 1121, 765 and 695 cm-1; 1H-NMR (δ, CDCl3, 400 MHz): 5.81 (2H, s), 6.64 (1H, t, J=7.3 Hz), 6.98 (1H, d, J=7.3 Hz), 7.31-7.40 (6H, m), and 8.58 ppm (1H, s). Anal. Calcd for C12H11NOS: C, 66.33; H, 5.10; N, 6.45%. Found: C, 66.23; H, 4.95; N, 6.32%.
3-Hydroxy-1-phenethyl-2(1H)-pyridinethione (3f): as yellow solids; yield: 93%; mp 104.8-106.1 °C; IR (KBr): 3639, 3076, 3024, 1628, 1599, 1475, 1432, 1283, 1122, 768 and 700 cm-1; 1H-NMR (δ, CDCl3, 400 MHz): 3.26 (2H, t, J=7.3 Hz), 4.74 (2H, t, J=7.3 Hz), 6.51 (1H, t, J=6.7 Hz), 6.99 (1H, d, J=6.7 Hz), 7.17-7.32 (5H, m), and 8.62 ppm (1H, s). Anal. Calcd for C13H13NOS: C, 67.50; H, 5.66; N, 6.06%. Found: C, 67.56; H, 5.47; N, 5.90%.
3-Hydroxy-1-phenylpropyl-2(1H)-pyridinethione (3g): as yellow solids; yield: 75%; mp 56.5-57.3 °C; IR (KBr): 3461, 3056, 2944, 1623, 1547, 1463, 1420, 1282, 1116, 790 and 701 cm-1; 1H-NMR (δ, CDCl3, 400 MHz): 2.29 (2H, quint, J=7.6 Hz), 2.75 (2H, t, J=7.6 Hz), 4.54 (2H, t, J=7.6 Hz), 6.64 (1H, t, J=5.6 Hz), 6.97 (1H, d, J=5.6 Hz), 7.21-7.33 (6H, m), and 8.59 ppm (1H, s). Anal. Calcd for C14H15NOS: C, 68.54; H, 6.16; N, 5.71%. Found: C, 68.43; H, 6.19; N, 5.71%.
3-Hydroxy-1-dodecyl-2(1H)-pyridinethione (3h): as a yellow oil; yield: 72%; IR (neat): 3052, 2923, 2853, 1629, 1524, 1470, 1429, 1284, 1118, 767 and 736 cm-1; 1H-NMR (δ, CDCl3, 400 MHz): 0.88 (3H, t, J = 7.3 Hz), 1.25 (20H, m), 4.51 (3H, t, J=7.3 Hz,), 6.65 (1H, dd, J=7.1 and 7.6 Hz), 6.97 (1H, dd, J=1.6 and 7.6 Hz), 7.32 (1H, dd, J=1.6 and 7.1 Hz), and 8.60 ppm (1H, s). Anal. Calcd for C17H29NOS: C, 69.10; H, 9.89; N, 4.74%. Found: C, 69.21; H, 10.08; N, 4.86%.
General synthetic procedure for zinc(II) Complexes
A typical example: bis(1,2-dihydro-1-methyl-2-thioxo-3-pyridinolato)zinc(II) complex (4a). A solution of Zn(OAc)2 (155 mg, 0.708 mmol) in H2O/EtOH (1:1) mixture (7.2 mL) was added to a solution of 3a (200 mg, 1.41 mmol) in H2O/EtOH (1:2) mixture (20 mL). The reaction mixture was stirred for 5 h at rt, and then the precipitate was filtrated off. Drying in vacuo over anhydrous P2O5 gave the product 4a as white solids. Yield: 240 mg (83%); mp 355 °C (decomp.); IR (KBr): 3100, 3044, 1591, 1547, 1457, 1396, 1123, 760 and 705 cm-1; 1H-NMR (δ, CDCl3, 400 MHz): 4.13 (3H, s), 6.84 (1H, dd, J=6.1 and 8.0 Hz), 7.06 (1H, dd, J=0.7 and 8.0 Hz), and 7.22 ppm (1H, dd, J=0.7 and 6.1 Hz). Anal. Calcd for C12H12N2O2S2Zn·0.8H2O: C, 40.02; H, 3.81; N, 7.78%. Found: C, 39.91; H, 3.92; N, 7.61%. MALDI-TOF MS (m/z): 344.96 [M+H]+.
Bis(1,2-dihydro-1-ethyl-2-thioxo-3-pyridinolato)zinc(II) complex (4b): as pale yellow solids; yield: quant.; mp 205 °C (decomp.); IR (KBr): 3089, 2974, 1590, 1536, 1437, 1127, 769 and 715 cm-1; 1H-NMR (δ, CDCl3, 400 MHz): 1.58 (3H, t, J=7.2 Hz), 4.61 (2H, q, J=7.2 Hz), 6.86 (1H, dd, J=6.1 and 8.3 Hz), 7.06 (1H, dd, J=1.2 and 8.3 Hz), and 7.21 ppm (1H, dd, J=1.2 and 6.1 Hz). Anal. Calcd for C14H16N2O2S2Zn·0.8H2O: C, 43.31; H, 4.57; N, 7.22%. Found: C, 43.18; H, 4.61; N, 7.12%. MALDI-TOF MS (m/z): 373.81 [M+H]+.
Bis(1,2-dihydro-1-propyl-2-thioxo-3-pyridinolato)zinc(II) complex (4c): as white solids; yield: 98%; mp 243°C (decomp.); IR (KBr): 3046, 2872, 1590, 1542, 1437, 1135, 781 and 716 cm-1; 1H-NMR (δ, CDCl3, 400 MHz): 1.02 (3H, t, J=7.4 Hz), 2.06 (2H, sext, J=7.6 Hz), 4.50 (2H, t, J=7.6 Hz), 6.84 (1H, dd, J=6.1 and 8.2 Hz), 7.06 (1H, dd, J=1.2 and 8.2 Hz), and 7.18 ppm (1H, dd, J=1.2 and 6.1 Hz). Anal. Calcd for C16H20N2O2S2Zn·0.5H2O: C, 46.77; H, 5.15; N, 6.82%. Found: C, 46.78; H, 4.97; N, 6.84%. MALDI-TOF MS (m/z): 401.07 [M+H]+.
Bis(1,2-dihydro-1-butyl-2-thioxo-3-pyridinolato)zinc(II) complex (4d): as white solids; yield: 92%; mp 106 °C (decomp.); IR (KBr): 3073, 2958, 2932, 1628, 1545, 1472, 1429, 1283, 1138, 769 and 713 cm-1; 1H-NMR (δ, CDCl3, 400 MHz): 0.99 (3H, t, J=7.3 Hz), 1.43 (2H, sext, J=7.3 Hz), 1.99 (2H, quint, J=7.3 Hz), 4.53 (2H, t, J=7.6 Hz), 6.84 (1H, dd, J=6.2 and 8.2 Hz), 7.04 (1H, dd, J=1.2 and 8.2 Hz), and 7.17 ppm (1H, dd, J=1.2 and 6.2 Hz). Anal. Calcd for C18H24N2O2S2Zn· 0.5H2O: C, 49.26; H, 5.74; N, 6.38%. Found: C, 49.29; H, 5.82; N, 6.32%. MALDI-TOF MS (m/z): 428.98 [M+H]+.
Bis(1,2-dihydro-1-benzyl-2-thioxo-3-pyridinolato)zinc(II) complex (4e): as white solids; yield: 98%; mp 232 °C (decomp.); IR (KBr): 3072, 3032, 1590, 1544, 1438, 1116, 767 and 697 cm-1; 1H-NMR (δ, CDCl3, 400 MHz): 5.79 (2H, s), 6.85 (1H, dd, J=6.0 and 8.2 Hz), 7.07 (1H, dd, J=1.2 and 8.2 Hz), 7.21 (1H, dd, J=1.2 and 6.0 Hz), and 7.27-7.39 ppm (5H, m). Anal. Calcd for C24H20N2O2S2Zn: C, 57.89; H, 4.05; N, 5.63%. Found: C, 57.86; H, 3.90; N, 5.60%. MALDI-TOF MS (m/z): 497.09 [M+H]+.
Bis(1,2-dihydro-1-phenethyl-2-thioxo-3-pyridinolato)zinc(II) complex (4f): as white solids; yield: 97%; mp 247 °C (decomp.); IR (KBr): 3096, 3024, 1592, 1537, 1492, 1113, 773 and 702 cm-1; 1H-NMR (δ, CDCl3, 400 MHz): 3.33 (2H, t, J=7.4 Hz), 4.75 (2H, t, J=7.4 Hz), 6.73 (1H, dd, J=6.1 and 8.3 Hz), 6.89 (1H, dd, J=0.96 and 6.1 Hz), 7.08 (1H, dd, J=0.96 and 8.3 Hz), and 7.17-7.32 ppm (5H, m). Anal. Calcd for C26H24N2O2S2Zn: C, 59.37; H, 4.60; N, 5.33%. Found: C, 59.41; H, 4.35; N, 5.30%. MALDI-TOF MS (m/z): 525.18 [M+H]+.
Bis(1,2-dihydro-1-phenylpropyl-2-thioxo-3-pyridinolato)zinc(II) complex (4g): as white solids; yield: 88%; mp 80 °C (decomp.); IR (KBr): 3024, 2926, 1589, 1539, 1494, 1115, 750 and 700 cm-1; 1H-NMR (δ, CDCl3, 400 MHz): 2.36 (2H, quint, J=7.5 Hz), 2.76 (2H, t, J=7.5 Hz), 4.53 (2H, t, J=7.6 Hz), 6.83 (1H, dd, J=6.3 and 7.5 Hz), 7.05 (1H, dd, J=1.0 and 7.5 Hz), 7.11 (1H, dd, J=1.0 and 6.3 Hz), and 7.22-7.33 ppm (6H, m). Anal. Calcd for C28H28N2O2S2Zn·0.5H2O: C; 59.73, H; 5.19, N; 4.98%. Found: C; 59.78, H; 5.09, N; 4.96%. MALDI-TOF MS (m/z): 553.09 [M+H]+.
Bis(1,2-dihydro-1-dodecyl-2-thioxo-3-pyridinolato)zinc(II) complex (4h): as pale yellow solids; yield: 93%; IR (KBr): 3090, 2923, 1590, 1537, 1436, 1123, 769 and 710 cm-1; 1H-NMR (δ, CDCl3, 400 MHz): 0.88 (3H, t, J=7.3 Hz), 1.25 (20H, m), 4.51 (3H, t, J=7.3 Hz), 6.83 (1H, dd, J=7.1 and 7.6 Hz), 7.04 (1H, dd, J=1.6 and 7.6 Hz), and 7.17 ppm (1H, dd, J=1.6 and 7.1 Hz). Anal. Calcd for C34H56N2O2S2 Zn·2.5H2O: C, 58.39; H, 8.79; N, 4.01%. Found: C, 58.24; H, 8.63; N, 4.12%. MALDI-TOF MS (m/z): 651.66 [M]+.
General synthetic procedure for vanadyl complexes
A typical example: bis(1,2-dihydro-1-methyl-2-thioxo-3-pyridinolato)oxovanadium(IV) complex (5a). A solution of 3a (252 mg, 1.78 mmol) in H2O (10 mL) was dissolved by adding 10M KOH. Then a solution of VOSO4·3H2O (194 mg, 0.89 mmol) in H2O (2 mL) was added to the solution. The mixture was adjusted to pH 10 with 6M HCl, and then the reaction mixture was stirred for 48 h at rt. The resulting precipitate was collected by filtration, washed with H2O, and dried over anhydrous P2O5 in vacuo to give the product 5a as brown solids. Yield: 144 mg (47%); IR (KBr): 3092, 1594, 1541, 1456, 1413, 1128, 967, 778 and 671 cm-1. ESR parameters in DMSO at 77K: g0=1.975, g//=1.959, g⊥=1.983, A0=85 x 10-4 cm-1, A//=160 x 10-4 cm-1, A⊥=48 x 10-4 cm-1. Anal. Calcd for C12H12N2O3S2V: C, 41.50; H, 3.48; N, 8.07%. Found: C, 41.67; H, 3.35; N, 8.07%. MALDI- TOF MS (m/z): 346.92 [M]+.
Bis(1,2-dihydro-1-ethyl-2-thioxo-3-pyridinolato)oxovanadium(IV) complex (5b): as brown solids; yield: 53%; IR (KBr): 3093, 1594, 1542, 1427, 1119, 973, 772 and 670 cm-1. ESR parameters in DMSO at 77K: g0=1.977, g//=1.959, g⊥=1.986, A0=83 x 10-4 cm-1, A//=155 x 10-4 cm-1, A⊥=47 x 10-4 cm-1. Anal. Calcd for C14H16N2O3S2V·0.2H2O: C, 44.37; H, 4.36; N, 7.39%. Found: C, 44.29; H, 4.31; N, 7.40%. MALDI- TOF MS (m/z): 374.93 [M]+.
Bis(1,2-dihydro-1-propyl-2-thioxo-3-pyridinolato)oxovanadium(IV) complex (5c): as brown solids; yield: 43%; IR (KBr): 3092, 1592, 1544, 1424, 1112, 971, 791 and 680 cm-1. ESR parameters in DMSO at 77K: g0=1.979, g//=1.913, g⊥=2.001, A0=80 x 10-4 cm-1, A//=83 x 10-4 cm-1, A⊥=79 x 10-4 cm-1. Anal. Calcd for C16H20N2O3S2V: C, 47.64; H, 5.00; N, 6.94 %. Found: C, 47.81; H, 5.06; N, 6.92 %. MALDI-TOF MS (m/z): 403.00 [M]+.
Bis(1,2-dihydro-1-buthyl-2-thioxo-3-pyridinolato)oxovanadium(IV) complex (5d): as brown solids; yield: 73%; IR (KBr): 2955, 2930, 1592, 1543, 1436, 1115, 970, 792 and 720 cm-1. ESR parameters in DMSO at 77K: g0=1.977, g//=1.936, g⊥=1.997, A0=80 x 10-4 cm-1, A//=102 x 10-4 cm-1, A⊥=69 x 10-4 cm-1. Anal. Calcd for C18H24N2O3S2V: C, 50.11; H, 5.61; N, 6.49%. Found: C, 50.21; H, 5.53; N, 6.43%. MALDI-TOF MS (m/z): 431.00 [M]+.
Bis(1,2-dihydro-1-benzyl-2-thioxo-3-pyridinolato)oxovanadium(IV) complex (5e): as brown solids; yield: 82%; IR (KBr): 3026, 2939, 1591, 1542, 1456, 1119, 975, 776, 720 and 679 cm-1. ESR parameters in DMSO at 77K: g0=1.989, g//=1.958, g⊥=2.004, A0=83 x 10-4 cm-1, A//=162 x 10-4 cm-1, A⊥=44 x 10-4 cm-1. Anal. Calcd for C24H20N2O3S2V: C, 57.71; H, 4.04; N, 5.61%. Found: C, 58.05; H, 3.97; N, 5.71%. MALDI- TOF MS (m/z): 498.51 [M]+.
Bis(1,2-dihydro-1-phenethyl-2-thioxo-3-pyridinolato)oxovanadium (IV) complex (5f): as brown solids; yield: 60%; IR (KBr): 3093, 3028, 1595, 1541, 1435, 1117, 974, 750 and 700 cm-1. ESR parameters in DMSO at 77K: g0=1.985, g//=1.963, g⊥=1.997, A0=83 x 10-4 cm-1, A//=155 x 10-4 cm-1, A⊥=47 x 10-4 cm-1. Anal. Calcd for C26H24N2O3S2V: C; 59.19, H; 4.59, N; 5.31%. Found: C; 59.45, H; 4.48, N; 5.31%. MALDI- TOF MS (m/z): 526.94 [M]+.
Bis(1,2-dihydro-1-phenylpropyl-2-thioxo-3-pyridinolato)oxovanadium (IV) complex (5g): as brown solids; yield: 92%; IR (KBr): 3022, 2943, 1593, 1542, 1424, 1118, 970, 773 and 699 cm-1. ESR parameters in DMSO at 77K: g0=1.985, g//=1.958, g⊥=1.999, A0=78 x 10-4 cm-1, A//=159 x 10-4 cm-1, A⊥=37 x 10-4 cm-1. Anal. Calcd for C28H28N2O3S2V: C; 60.53, H; 5.08, N; 5.04%. Found: C; 60.80, H; 5.14, N; 5.02%. MALDI- TOF MS (m/z): 555.12 [M]+.
The pKa measurement of compound 3b
A solution of compound 3b (30 mg, 0.19 mmol) in H2O (25mL) was titrated with 25 mM-NaOH solution at rt under Ar atmosphere. The pKa was calculated to be 9.4 from the pH at midpoint of neutralization.
Measurement of the partition coefficient
To a solution of sample (5 mL) in KRB buffer solution (120 mM NaCl, 1.27 mM CaCl2, 1.2 mM MgSO4, 4.75 mM KCl, 1.2 mM KH2PO4, 24 mM NaHCO3 and 5 mM glucose: pH 7.4) was added octanol (5 mL). The mixture was stirred at 600 rpm for 4 h with a magnetic stirrer, and then centrifuged at 4000 rpm for 20 min with a centrifugal separator. The concentrations of two phases were calculated using UV-vis spectral data. The partition coefficient was calculated from the following equation; logPow= log(Coctanol/Cbuffer).
Evaluation of the inhibitory effect of the metal complexes on FFA release from rat adipocytes
Male Wistar rats, whose the age is 7 weeks, were killed by decapitation under anesthesia with Et2O, and adipocytes were isolated from epididymal fat pads. Fat tissues were incubated for 1 h at 37ºC in KRB buffer (120 mM NaCl, 1.27 mM CaCl2·2H2O, 1.20 mM MgSO4, 4.75 mM KCl, 1.20 mM KH2PO4, and 24 mM NaHCO3: pH 7.4) containing collagenase and 2% bovine serm albumin (BSA). Adipocytes were then separated by filtration through mesh, washed three times with the above buffer, and prepared for 240 µL (2.5 x 106 cells/mL). Isolated adipocyte solutions were preincubated at 37ºC for 0.5 h with various concentrations of the metal complexes in DMSO containing 5 mM glucose. Then 10 µM epinephrine was added to the reaction mixtures and the resulting solutions were incubated at 37ºC for 3 h.
The reactions were stopped by soaking in ice water and the mixtures were centrifuged at 3000 rpm at 4ºC for 10 min. For the outer solution of the cells, FFA levels were determined with an NEFA C-test Wako (Wako Pure Chemicals, Osaka, Japan).
ACKNOWLEDGEMENTS
This work was partially supported by Grants-in-Aid for Scientific Research C (No. 21550068) from the Japan Society for the Promotion of Science.
References
1. S. Wild, G. Roglic, A. Green, R. Sicree, and H. King, Diabetes Care, 2004, 27, 1047. CrossRef
2. WHO, Diabetes Mellitus, Reports of a WHO Study Group, WHO Technical Report Series, 1985, pp. 727 and 876.
3. H. Sakurai, Y. Kojima, Y. Yoshikawa, K. Kawabe, and H. Yasui, Coord. Chem. Rev., 2002, 226, 187. CrossRef
4. H. Sakurai, The Cheical. Record, 2002, 2, 237. CrossRef
5. M. Nakai, H. Watanabe, C. Fujiwara, H. Kagawa, T. Satoh, J. Takada, R. Matsushita, and H. Sakurai, Biol. Pharm. Bull., 1995, 18, 719.
6. L. Coulston and P. Dandona, Diabetes, 1980, 29, 665. CrossRef
7. J. M. May and C. S. Contoreggi, J. Biol. Chem., 1982, 257, 4362.
8. A. Shisheva, D. Gefel, and Y. Shechter, Diabetes, 1992, 41, 982. CrossRef
9. M. Yamaguchi, K. Wakasugi, R. Saito, Y. Adachi, Y. Yoshikawa, H. Sakurai, and A. Katoh, J. Inorg. Biochem., 2006, 100, 260. CrossRef
10. Y. Adachi, J. Yoshida, Y. Kodera, A. Katoh, Y. Yoshikawa, Y. Kojima, and H. Sakurai, J. Biol. Inorg. Chem., 2004, 9, 885. CrossRef
11. Y. Yoshikawa, E. Ueda, K. Kawabe, H. Miyake, H. Sakurai, and Y. Kojima, Chem. Lett., 2000, 29, 874. CrossRef
12. M. Yamane, Y. Adachi, Y. Yoshikawa, and H. Sakurai, Chem. Lett., 2005, 34, 1694. CrossRef
13. Y. Yoshikawa, M. Kondo, H. Sakurai, and Y. Kojima, J. Inorg. Biochem., 2005, 99, 1497. CrossRef
14. Y. Kojima, Y. Yoshikawa, E. Ueda, N. Kishimoto, M. Tadokoro, and H. Sakurai, Bull. Chem. Soc. Jpn., 2005, 78, 451. CrossRef
15. S. Yamamoto, Y. Yoshikawa, E. Ueda, T. Yamashita, N. Kajiwara, H, Sakurai, and Y. Kojima, Biomed. Res. Trace Elm., 2004, 15, 85.
16. Y. Adachi, J. Yoshida, Y. Kodera, and H. Sakurai, Chem. Lett., 2005, 34, 656. CrossRef
17. A. Katoh, T. Tsukahara, R. Saito, K. K. Ghosh, Y. Yoshikawa, Y. Kojima, A. Tamura, and H. Sakurai, Chem. Lett., 2002, 31, 114. CrossRef
18. A. Katoh, M. Yamaguchi, R. Saito, Y. Adachi, and H. Sakurai, Chem. Lett., 2004, 33, 1274; CrossRef M Yamaguchi, R. Saito, Y. Adachi, Y. Yoshikawa, H. Sakurai, and A. Katoh, Heterocycles, 2007, 73, 603. CrossRef
19. A. Katoh, K. Taguchi, H. Okada, M. Harata, Y. Fujisawa, T. Takino, and H. Sakurai., Chem. Lett., 2000, 29, 866. CrossRef
20. H. Sakurai, A. Tamura, J. Fugono, H. Yasui, and T. Kiss, Coord. Chem. Rev., 2003, 245, 31. CrossRef
21. M. Rangel, A. Tamura, C. Fukushima, and H. Sakurai, J. Biol. Inorg. Chem., 2001, 6, 128. CrossRef
22. Y. Adachi, J. Yoshida, Y. Kodera, A. Katoh, J. Takada, and H. Sakurai, J. Med. Chem., 2006, 49, 3251. CrossRef
23. J. H. McNeil, V. G. Yuen, H. R. Hoveyda, and C. Orvig, J. Med. Chem., 1992, 35, 1489. CrossRef
24. T. K. Saha, Y. Yoshikawa, H. Yasui, and H. Sakurai, Bull. Chem. Soc. Jpn., 2006, 79, 1191. CrossRef
25. L. C. Y. Woo, V. G. Yuen, K. H. Thompson, J. H. McNeil, and C. Orvig, J. Inorg. Biochem., 1999, 76, 251. CrossRef
26. H. Sakurai, K. Fujii, H. Watanabe, and H. Tamura, Biochem. Biophys. Res. Commun., 1995, 214, 1095. CrossRef
27. P. Noblía, M. Vieites, M. H. Torre, A. J. Costa-Filho, H. Cerecetto, M. González, M. L. Lavaggi, Y. Adachi, H. Sakurai, and D. Gambio, J. Inorg. Biochem., 2006, 100, 281. CrossRef
28. Y. Adachi and H. Sakurai, Chem. Pharm. Bull., 2004, 52, 428. CrossRef
29. T. Takino, H. Yasui, A. Yoshitake, Y. Hamajima, R. Matsushita, J. Takada, and H. Sakurai, J. Biol. Inorg. Chem., 2001, 6, 133. CrossRef
30. V. Monga, K. H. Thompson, V. G. Yuen, V. Sharma, B. O. Patrick, J. H. McNeil, and C. Orvig, Inorg. Chem., 2005, 44, 2678. CrossRef
31. A. Katoh, Y. Matsumura, Y. Yoshikawa, H. Yasui, and H. Sakurai, J. Inorg. Biochem., 2009, 103, 567. CrossRef
32. H. Sakurai, A. Katoh, and Y. Yoshikawa, Bull. Chem. Soc. Jpn., 2006, 79, 1645. CrossRef
33. M. Streater, P. D. Taylor, R. C. Hider, and J. Porter, J. Med. Chem., 1990, 33, 1749; CrossRef B. L. Ellis, A. K. Duhme, R. C. Hider, M.B. Hossain, S. Rizvi, and D. van der Helm, J. Med. Chem., 1996, 39, 3659. CrossRef
34. M. C. Barret, M. C. Mahon, C. K. Molloy, J. W. Streed, and P. Wright, Inorg. Chem., 2001, 40, 4384. CrossRef
35. R. C. Scarrow, P. E. Riley, K. Abu-Dari, D. L. White, and K. N. Raymond, Inorg. Chem., 1985, 24. 954. CrossRef
36. H. Sakurai, K. Tsuchiya, M. Nukatsuka, M. Sofue, and J. Kawada, J. Endocrinol., 1990, 126, 451. CrossRef
37. Y. Adachi, J. Yoshida, Y. Kodera, T. Kiss, T. Jakushu, E. A. Enyedy, Y. Yoshikawa, and H. Sakurai, Biochem. Biophys. Res. Commun., 2006, 351, 165. CrossRef