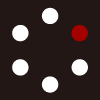
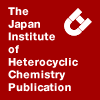
HETEROCYCLES
An International Journal for Reviews and Communications in Heterocyclic ChemistryWeb Edition ISSN: 1881-0942
Published online by The Japan Institute of Heterocyclic Chemistry
e-Journal
Full Text HTML
Received, 4th November, 2009, Accepted, 10th December, 2009, Published online, 10th December, 2009.
DOI: 10.3987/COM-09-11870
■ Cephastigiamide A, and Antiplasmodial Activity of Cephalotaxus Alkaloids from Cephalotaxus harringtonia Forma Fastigiata
Hiroshi Morita,* Yuta Nagakura, Takahiro Hosoya, Wiwied Ekasari, Aty Widyawaruyanti, Kanami Mori-Yasumoto, Setsuko Sekita, and Yusuke Hirasawa
Faculty of Pharmaceutical Sciences, Hoshi University, 2-4-41 Ebara, Shinagawa-ku, Tokyo 142-8501, Japan
Abstract
A new Cephalotaxus alkaloid, cephastigiamide A (1), has been isolated from the leaves of Cephalotaxus harringtonia forma fastigiata and the structure was elucidated by 2D NMR analysis and chemical degradation. Harringtonine, deoxyharringtonine, and homodeoxyharringtonine showed pronounced antiplasmodial activity against Plasmodium falciparum 3D7 but not against Leishmania major. Structure-activity relationship for antiplasmodial activity of isolated Cephalotaxus alkaloids was also discussed.Malaria caused by parasites of the genus Plasmodium is a major parasitic infection disease and continues to cause morbidity and mortality on a large scale in tropical countries.1 A major contributor to malarial morbidity and mortality is almost certainly the increasing resistance of malaria parasites to available drugs.2 Such a situation has heralded the need for alternative antiplasmodial therapy. On the other hand, leishmaniasis is also one of a major tropical disease,3 which affects populations of the developing countries like in the case of malaria. Current treatment against leishmaniasis is based on toxic chemotherapeutic agents with serious side effects.4 These situations have prompted us to find new natural products for possible antimalarial and antileishmanial actions.
Cephalotaxus alkaloids such as cephalotaxine and homoharringtonine are a family of cytotoxic heterocyclic natural products with the unique ring system and elaborated by trees of the genus Cephalotaxus (Cephalotaxaceae), some of which showed antileukemic activity by intraperitoneal injection to mice.5 Clinical studies of homoharringtonine in U.S.A. have shown that it has a certain effect on two strains of chloroquine-resistant Plasmodium falciparum malaria.6 The rational search for bioactive natural products in higher plants is a promising strategy for antiplasmodial and antileishmanicidal drug discovery. Our screening program for antiplasmodial and leishmanicidal plants resulted in the isolation of cephastigiamide A (1), a new alkaloid, from the leaves of C. harringtonia forma fastigiata together with known related alkaloids. Activities of these isolated Cephalotaxus alkaloids were evaluated against Plasmodium falciparum 3D7 and Leishmania major. Here, we describe the isolation and structure elucidation of 1 and parasitic antiprotozoa activity of Cephalotaxus alkaloids. Structure-activity relationship for antiplasmodial activity of Cephalotaxus alkaloids was also discussed.
The CHCl3-soluble materials prepared from the leaves of C. harringtonia forma fastigiata were purified by column chromatography to afford cephastigiamide A (1, 8.3 mg, 0.0002%) as colorless solid together with known related alkaloids, cephalotaxine (2),7 cephalotaxinamide (3),8 isoharringtonic acid (4),8 isoharringtonine (5),9 harringtonine (6),9 deoxyharringtonine (7),9 homoharringtonine (8),9 homodeoxyharringtonine (9),9 homoharringtonine-β-N-oxide (10),10 homoharringtonine-α-N-oxide (11),10 cephalezomine B (12),11 cephalezomine E (13),11 cephalezomine F (14),11 cephalocyclidin A (15),12 and bis-cephalezomine A (16).13
Cephastigiamide A (1), colorless solid, [α]D20 –55°(c 0.1, MeOH), showed pseudomolecular ion at m/z 546 (M+H)+ in the ESITOFMS, and the molecular formula, C28H35NO10, was established by HRESITOFMS [m/z 546.2339, (M+H)+, Δ ±0 mmu]. The IR absorption bands were characteristic of hydroxyl (3440 cm-1), ester carbonyl (1740 cm-1), and amide carbonyl (1680 cm-1) groups. Analysis of 1H and 13C NMR data (Table 1) and the HSQC spectrum provided evidence that 1 possessed 28 carbon signals including 10 quaternary carbons (sp2 × 8 and sp3 × 2), 7 methines (sp2 × 3 and sp3 × 4), 7 methylenes, and 4 methyls. Among them, two quaternary carbons (δC 161.8 and 80.8), two methines (δC 75.1 and 75.8), and one mehylene (δC 102.3) were ascribed to those bearing an oxygen atom, the carbon at δC 177.7 was assigned to amide carbonyl, and the carbons at δC 173.0 and 173.4 were assigned to ester carbonyls. The 1H-1H COSY spectrum revealed the connectivities of C-3 to C-4, C-6 to C-7, C-10 to C-11, and C-1'' to C-5''.
In the HMBC spectrum, these spectral evidences suggested that 1 was a congener of isoharringtonine (5) with a ketone at C-8. The HMBC correlations of H2-7 to C-8, characteristic for cephalotaxinamide-type skeleton with a side chain at C-3, were observed.8 HMBC cross-peaks of H-3' to C-2' (δC 80.8), C-4' (δC 173.0), and C-1'' (δC 34.9), and H3-OMe (5') to C-4' revealed the presence of the methyl ester of 2-hydroxy-2-isopentyl-3-hydroxy-butanedioic acid in isoharringtonine (5). The connectivity between C-3 and C-1', was indicated by HMBC correlations of H-3 and H-3' to C-1' (δC 173.4). Thus, the structure of cephastigiamide A was assigned as 1.
The relative stereochemistry of the backbone skeleton was deduced from NOESY correlations as shown in Fig. 1, which was the same as that of cephalotaxinamide (3).8 The CD spectrum ([θ]218 –11400, [θ]259 –390, and [θ]285 –1795) of 1 showed Cotton effects similar to those ([θ]220 –11700, [θ]259 –50, and [θ]295 –1880) of 3. Furthermore, the CD spectrum for the molybdate complex of the dicarboxylic acid moiety (C-1' ~ C-4' and C-1'' ~ C-5'') derived from the acid hydrolysate showed a negative Cotton effect at 270 nm, indicating that the absolute configurations at C-2' was R. 14 To determine the absolute configuration at C-3', 1 was converted into (S)- and (R)-2-methoxy-2- trifluoromethylphenylacetic acid (MTPA) esters. The values of Δδ [δ (S–MTPA ester) – δ (R–MTPA ester)] obtained from the 1H NMR spectra of the MTPA esters suggested that the absolute configurations at C-3' of 1 was S (Figure 2).15 Therefore the absolute stereochemistry of 1 was elucidated to be 3S, 4S, 5R, 2'R, and 3'S.16
Activities of these Cephalotaxus alkaloids (1-16) against the promastigote from Plasmodial falciparum 3D7 and Leishmania major were evaluated and some of them showed pronounced in vitro activity against P. falciparum 3D7. Cytotoxicity was tested against human lung adenocarcinoma epithelial cell line (A549). All alkaloids possessing Cephalotaxus common skeleton except for cephlocyclidin A (15) exhibited antiplamodial acitivity with IC50 values in the range of 0.0014 – 17.82 µM, whereas they did not show promising antileishmanial activity on L. major (Table 1). Harringtonine (6), deoxyharringtonine (7), and homodeoxyharringtonine (9) showed more effective antiplasmodial activity (IC50 6: 0.0043 µM; 7: 0.0014 µM; 9: 0.0018 µM) than homoharringtonine (IC50 8: 0.012 µM). Selectivity index (SI) of 6, 7, and 9 (SI 32.6, 15.0, and 37.8, respectively), which is the values of the IC50 (cytotoxicity) obtained in A549 divided by the IC50 in P. falciparum 3D7, also showed more effective than that of 8 (SI 7.0). It should be noted that the alkaloids with a side chain were significantly toxic to A549 more than Leishmania major. In the P. falciparum assay, compounds 5 and 13 with 3'S-hydroxy functionality showed potent activity (IC50 5: 0.045 µM; 13: 0.071 µM) and good selectivity (SI 5: 86.2; 13: 67.5). The presence of a side chain at C-3 might be closely related to antiplasmodial activity in these Cephalotaxus alkaloids. Amide-type alkaloids such as cephastigiamide A (1), cephalotaxinamide (3), and two N-oxide derivatives 10 and 11 did not show potent activity even if they possess a side chain at C-3. Dimeric bis-cephalozomine A (16) did not show antiplasmodial activity, although drupacine-type alkaloid with a side chain, cephalezomine B (12) showed relatively potent activity and good selectivity (IC50 0.040 µM; SI 44.5). Further analysis is needed to elucidate the structural and pharmacological features that may be important for the biological properties of this class of molecules.
EXPERIMENTAL
General Experimental Procedures. Optical rotations were measured on a JASCO DIP-1000 automatic digital polarimeter. CD spectra were measured on a JASCO J-820 spectropolarimeter, and IR spectra were recorded on a JASCO FT/IR-4100 spectrophotometer. 1H and 2D NMR spectra were recorded on a JEOL ECA600 and Bruker AV 400 spectrometers, and chemical shifts were referenced to the residual solvent peaks (δH 3.31 and δC 49.0 for CD3OD). Standard pulse sequences were employed for the 2D NMR experiments. 1H–1H COSY and NOESY spectra were measured with spectral widths of both dimensions of 4800 Hz, and 32 scans with two dummy scans were accumulated into 1K data points for each of 256 t1 increments. NOESY spectra in the phase-sensitive mode were measured with a mixing time of 800 ms. For HSQC spectra in the phase sensitive mode and HMBC spectra, a total of 256 increments of 1K data points were collected. For HMBC spectra with Z-axis PFG, a 50 ms delay time was used for long-range C–H coupling. Zero-filling to 1K for F1 and multiplication with squared cosine-bell windows shifted in both dimensions were performed prior to 2D Fourier transformation. High-resolution ESI MS were obtained on a LTQ Orbitrap XL (Thermo Scientific).
Plant Material. The leaves of Cephalotaxus harringtonia forma fastigiata were collected at Chiba, Japan in 2006. The botanical identification was made by Mr. M. Daitoku, School of Pharmacy, Tokyo University of Pharmacy & Life Sciences. A voucher specimen has been deposited in the herbarium of Hoshi University.
Extraction and Isolation. The leaves of C. harringtonia forma fastigiata (500 g) were crashed and extracted with MeOH, the MeOH extract was partitioned with EtOAc and 3% tartaric acid. Water-soluble materials were adjusted at pH 9 with sat. Na2CO3 aq. and partitioned with CHCl3. CHCl3-soluble materials were subjected to a LH-20 column with CHCl3/MeOH (1:1) followed by C18 HPLC (40% MeCN/0.1%TFA) to afford cephastigiamide A (1, 8.3 mg, 0.0002%) as colorless solid together with known related alkaloids, cephalotaxine (2),7 cephalotaxinamide (3),8 isoharringtonic acid (4),8 isoharringtonine (5),9 harringtonine (6),9 deoxyharringtonine (7),9 homoharringtonine (8),9 homodeoxyharringtonine (9),9 homoharringtonine-β-N-oxide (10),10 homoharringtonine-α-N-oxide (11),10 cephalezomine B (12),11 cephalezomine E (13),11 cephalezomine F (14),11 cephalocyclidin A (15),12 and bis-cephalezomine A (16).13
Cephastigiamide A (1): colorless amorphous solid, [α]D20 –55° (c 1.0, MeOH); IR (KBr) νmax 3440, 2960, 1740, 1680, and 1650 cm-1; 1H and 13C NMR (Table 1); ESIMS (pos.) m/z 546 (M+H)+; HRESITOFMS (pos.) m/z 546.2339 (M+H)+, calcd. for C28H36NO10. CD (MeOH) [θ]218 –11400, [θ]259 –390, and [θ]285 –1795.
(R)- and (S)-MTPA esters of Cephastigiamide A (1). To a solution of 1 (0.2 mg) in CHCl3 (50 µL) was added (–)- or (+)-MTPACl (1.1 µL), triethylamine (1.3 µL) and 4-N,N-dimethylaminopyridine (0.2 mg). The mixture was allowed to stand at room temperature for 2 h. N,N-Dimethylamino- 1,3-propandiamine (1.0 µL) was added, and after evaporation of solvent, the residue was applied to a silica gel column (CHCl3-MeOH, 20:1) to give the (S)-MTPA ester of 1 (0.2 mg). The (R)-MTPA ester was prepared according to the same procedure as described above.
(S)-MTPA ester of 1: ESIMS m/z 762 (M+H)+; 1H NMR (CD3OD) δ 5.04 (s, H-1), 5.96 (d, 9.6, H-3), 4.08 (d, 9.6, H-4), 2.15 (m, H-6), 1.25 and 2.22 (m, H-7), 3.10 and 3.92 (m, H-10), 2.52 and 3.26 (m, H-11), 6.37 (s, H-14), 6.68 (s, H-17), 5.82 and 5.87 (s, H-18), 3.80 (s, 2-OMe), 3.60 (s, H-3'), 3.52 (s, 4'-OMe), 1.51 (m, H-1''), 0.95 (m, H-2''), 1.38 (m, H-3''), 0.86 (d, 6.5, H-4''), 0.87 (d, 6.5, H-5'').
(R)-MTPA ester of 1: ESIMS m/z 762 (M+H)+; 1H NMR (CD3OD) δ 5.06 (s, H-1), 6.12 (d, 9.6, H-3), 4.06 (d, 9.6, H-4), 2.14 (m, H-6), 1.25 and 2.20 (m, H-7), 3.10 and 3.93 (m, H-10), 2.54 and 3.27 (m, H-11), 6.71 (s, H-14), 6.69 (s, H-17), 5.81 and 5.87 (s, H-18), 3.77 (s, 2-OMe), 3.69 (s, H-3'), 3.42 (s, 4'-OMe), 1.55 (m, H-1''), 1.03 (m, H-2''), 1.48 (m, H-3''), 0.87 (d, 6.5, H-4''), 0.88 (d, 6.5, H-5'').
Molybdate complexes of hydrolysates of cephastigiamide A (1). Cephastigiamide A (1, 1 mg) was hydrolyzed with 3 N HCl (1 mL) under reflux for 4 days. After cooling, 3M NH4OH was added and the alkaline phase was extracted with CHCl3. Excess NH4OH was neutralized and the solvent was evaporated under reduced pressure. The residue was used directly in the preparation of solution for CD measurement, which contained 3 mM each hydrolysates of 1 and 2.7 mM sodium molybdate. HCl and NaOH solution were added until pH 2.9 - 3.1 was reached. Measurements of CD spectra were carried out in a 2 mm cell 5 days after the solution had been prepared.
Antiplasmodial Activity Human malaria parasites were cultured according to the method of Trager et al. 17 The antimalarial activity of the isolated compounds was determined by the procedure described by Budimulja et al.18 In brief, stock solutions of the samples were prepared in DMSO (final DMSO concentrations of < 0.5%) and were diluted to the required concentration with complete medium (RPMI 1640 supplemented with 10% human plasma, 25 mM HEPES and 25 mM NaHCO3 ) until the final concentrations of samples in culture plate wells were 10; 1; 0.1; 0.01; 0.001 µg/mL. The malarial parasite P. falciparum 3D7 clone was propagated in a 24-well culture plates. Growth of the parasite was monitored by making a blood smear fixed with MeOH and stained with Geimsa stain. The antimalarial activity of each compound was expressed as an IC50 value, defined as the concentration of the compound causing 50 % inhibition of parasite growth relative to an untreated control.
The percentage of growth inhibition was expressed according to following equation : Growth inhibition % = 100- [(test parasitaemia/control parasitemia) × 100. Chloroqine: IC50 0.011 µM.
Leishmanicidal Activity Assay. Cultivation of Leishmania promastigotes and leishmanicidal activity assay were carried out as we previously described.19
Cytotoxic Activity A549 (human lung adenocarcinoma epithelial cell line) was seeded onto 96-well microtiter plates at 5 × 103 cells per well. Cells were preincubated for 24 h at 37 °C in a humidified atmosphere of 5% CO2. Different concentrations of each compound (10 µL) were added to the cultures, and then the cells were incubated at 37 °C for 48 h. On the third day, 15 µL MTT solution (5 mg/mL) was added into each well of the cultured medium. After further 2 hours of incubation, 100 μL of 10% SDS-0.01N HCl solution was added to each well and the formazan crystals in each well were dissolved by stirring with a pipette. The optical density measurements were made using a micropipette reader (Benchmark Plus microplate spectrometer, BIO-RAD) equipped with a two wavelengths system (550 and 700 nm). In each experiment, three replicate of wells were prepared for each sample. The ratio of the living cells was determined based on the difference of the absorbance between those of samples and controls. These differences are expressed in percentage and cytotoxic activity was indicated as an IC50 value. Taxol: IC50 0.0014 µM.
ACKNOWLEDGMENTS
This work was partly supported by a Grant-in-Aid for Scientific Research from the Ministry of Education, Culture, Sports, Science, and Technology of Japan, and a grant from the Open Research Center Project. We acknowledge the Faculty of Pharmacy, Airlangga University for antimalarial activity and financial support.
References
1. (a) J. Wiesner, R. Ortmann, H. Jomaa, and M. Schlitzer, Angew. Chem. Int. Ed., 2003, 42, 5274; CrossRef (b) B. Greenwood and T. Mutabingwa, Nature, 2002, 415, 670; CrossRef (c) D. J. Wyler, Clinical Infectious Diseases, 1993, 16, 449.
2. (a) M. H. Gelb and W. G. Hol, Science, 2002, 297, 343; CrossRef (b) P. A. G. N. De Smet, Drugs, 1997, 54, 801. CrossRef
3. (a) R. W. Ashford, P. Desjeux, and P. deRaadt, Parasital. Today, 1992, 8, 104; CrossRef (b) C. A. C. Araujo, L. V. Alegrio, and L. L. Leon, Phytochemistry, 1998, 49, 751. CrossRef
4. R. Balana-Fouce, R. M. Reguera, J. C. Cubrîa, and D. Ordonez, Gen. Pharmacol., 1998, 30, 435. CrossRef
5. For reviews of the Cephalotaxus alkaloids, see: (a) M. A. J. Miah, T. Hudlicky, and J. W. Reed, In The Alkaloids, ed. by G. A. Cordell; Academic Press, New York, 1998; Vol. 51, p 199; (b) L. Huang and Z. Xue, The Alkaloids; ed. by A. Brossi; Academic Press, New York, 1984, Vol. 23, p 157.
6. J. M. Whaun and N. D. Brown, Ann. Trop. Med. Parasitol., 1990, 84, 229.
7. W. W. Paudler, G. I. Kerley, and J. McKay, J. Org. Chem., 1963, 28, 2194. CrossRef
8. X. Zhi, X. Lizhen, C. Dihua, and H. Liang, Acta Pharm. Sinica, 1981, 16, 752.
9. (a) R. G. Powell, D. Weisleder, C. R. Smith Jr., and W. K. Rohwedder, Tetrahedron Lett., 1970, 11, 815; CrossRef (b) K. L. Mikolajczak, R. G. Powell, and C. R. Smith, Jr., Tetrahedron, 1972, 28, 1995. CrossRef
10. I. Takano, I. Yasuda, M. Nishijima, Y. Hitotsuyanagi, K. Takeya, and H. Itokawa, J. Nat. Prod., 1996, 59, 965. CrossRef
11. H. Morita, M. Arisaka, N. Yoshida, and J. Kobayashi, Tetrahedron, 2000, 56, 2929. CrossRef
12. J. Kobayashi, M. Yoshinaga, N. Yoshida, M. Shiro, and H. Morita, J. Org. Chem., 2002, 67, 2283. CrossRef
13. M. Yoshinaga, H. Morita, T. Dota, and J. Kobayashi, Tetrahedron, 2004, 60, 7861. CrossRef
14. (a) S. Brandange, S. Josephson, and S. Vallen, Acta Chem. Scand., 1974, B28, 153; CrossRef (b) S. Brandange, S. Josephson, S. Vallen, and R. G. Powell, Acta Chem. Scand., 1974, B28, 1237. CrossRef
15. I. Ohtani, T. Kusumi, Y. Kashman, and H. Kakisawa, J. Am. Chem. Soc., 1991, 113, 4092. CrossRef
16. J. M. Cassady, P. Z. Cong, R. G. Cooks, R. Roush, C. J. Chang, and R. G. Powell, Yaoxue Xuebao, 1988, 23, 315; This structure has already been proposed by Cassady et al. only by MS spectrum in the crude fraction from Cephalotaxus harringtonia var. harringtonia, however isolation, structure elucidation, and any physicochemical and spectroscopic data of this compound have not been published yet.
17. W. Trager and J. B. Jensen, Science, 1976, 193, 673. CrossRef
18. A. S. Budimulja, T. P. Syafruddin, P. Wilairat, and S. Marzuki, Mol. Biochem. Parasitol, 1997, 84, 137. CrossRef
19. M. Takahashi, H. Fuchino, S. Sekita, M. Satake, and F. Kiuchi, Chem. Pharm. Bull., 2006, 54, 915. CrossRef