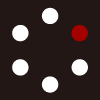
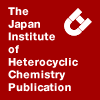
HETEROCYCLES
An International Journal for Reviews and Communications in Heterocyclic ChemistryWeb Edition ISSN: 1881-0942
Published online by The Japan Institute of Heterocyclic Chemistry
e-Journal
Full Text HTML
Received, 13th November, 2009, Accepted, 25th December, 2009, Published online, 29th December, 2009.
DOI: 10.3987/COM-09-11872
■ Reusable Polymer-Supported Terpyridine Copper Complex for [3+2] Huisgen Cycloaddition in Water
Toshimasa Suzuka,* Kazumasa Ooshiro, and Ken’yu Kina
Department of Chemistry, Biology and Marine Sciences, Faculty of Science, University of Ryukyus, Nishihara-cho, Okinawa 903-0213, Japan
Abstract
A polymer-supported terpyridine copper(I) complex was prepared and found to promote the Huisgen [3+2] cycloaddition reaction between azides and alkynes in water to give the corresponding triazoles with up to 87% isolated yield. This catalyst was recovered and reused several times without any loss in catalytic activity.INTRODUCTION
Huisgen 1,3-dipolar cycloaddition of organic azides and alkynes1 is recognized as the most successful method for synthesizing 1,2,3-triazole derivatives, which are important compounds used in agrochemicals and biochemicals.2 Sharpless et al. successfully reported copper-catalyzed Huisgen 1,3-dipolar cycloaddition in 2001; their new concept was named “click chemistry.”3 Since then, considerable research has been conducted on improving the efficiency of the homogeneous conditions and the applications of this reaction.4 One of the major problems with Sharpless et al.’s reaction lies in the reaction conditions where a copper reagent is required to promote the reaction at low temperatures to afford the corresponding 1,4-disubstituted-1,2,3-triazole derivatives with 100% regioselectivity, resulting in the contamination of the coupling products with metal residue. Recently, several studies have been conducted to addressing this problem. For example, the [3+2] cycloaddition reaction has been carried out with solid-supported copper(I) catalysts in organic solvent.5 In addition, Girard et al. examined the Huisgen [3+2] cycloaddition reaction of acetylenes with azides using Amberlyst A-21 supported Cu(I) catalyst in CH2Cl2 to give the corresponding 1,4-disubstitued 1,2,3-triazoles in high yield.6 If azides and acetylenes were reacted in water with recyclable copper catalysts, this would prevent the production of organic solvent wastes as well as metal-contaminated wastes, and the Huisgen [3+2] cycloaddition reaction would meet the green chemical requirements. To meet these requirements, we have developed an amphiphilic polystyrene-poly(ethylene glycol) (PS-PEG)7 resin-supported copper catalyst, which was used for the Huisgen [3+2] cycloaddition reaction in water. In this paper, we report our results demonstrating the Huisgen [3+2] cycloaddition reaction of azides and acetylenes in water in the presence of a copper complex of an amphiphilic PS-PEG resin-bound terpyridine ligand8 (Scheme 1).
RESULTS AND DISCUSSION
The amphiphilic PS-PEG resin-bound terpyridine ligand was prepared from p-hydroxybenzaldehyde (5), propane sultone, 2-acetylpyridine (7), and PS-PEG-N2 resin, and the coordination of the synthesized polymeric terpyridine ligand with copper species proceeded to give the PS-PEG resin-supported terpyridine copper complex 1, which exhibited good catalytic activity for the Huisgen [3+2] cycloaddition reaction. Thus, the reaction of p-hydroxybenzaldehyde (5) with propane sultone in IPA at 100 °C for 3 h under alkaline conditions followed by the treatment of the obtained 3-(4-formylphenoxy)propane-1- sulfonic acid (6) with 2-acetylpyridine (7) and AcONH4 in acetamide at 150 °C for 5 h gave terpyridine ligand 8 in 68% yield in two steps. Immobilization of terpyridine ligand 8 onto an amphiphilic PE-PEG resin through ionic bonds5f to the sulfonate group was performed in DMF at room temperature for 1 h and the complexation of an amphiphilic PS-PEG resin-bound terpyridine ligand and Cu(I) occurred in CH2Cl2 to give PS-PEG-terpyridine-Cu(I) complex 1 as a green solid.
To explore the catalytic activity of the resin-supported complex in water, we studied the copper-catalyzed Huisgen [3+2] cycloaddition reaction of acetylenes and azides in water, which is the first example of Huisgen [3+2] cycloaddition using a reusable heterogeneous catalyst in water. On screening reaction conditions for copper-catalyzed [3+2] cycloaddition with polymeric copper 1, we found that the reaction efficiency was greatly enhanced by the solvent used. Thus, the coupling of benzyl azide (2a) and phenylacetylene (3e) was performed in water with polymeric catalyst 1 (5 mol % to Cu) at 40 °C for 6 h in the solvent. The reaction mixture was filtered and the recovered resin beads were rinsed with a small amount of water and extracted with EtOAc to give 1-benzyl-4-phenyl-1H-1,2,3-triazole (4e). The most effective solvent was water, as seen in the results summarized in Table 1. The coupling in toluene, CH2Cl2, CHCl3, DMSO, and DMF gave 4e in 33%, 19%, 24%, 26%, and 18% yields, respectively. The use of IPA improved the coupling performance to afford 1-benzyl-4-phenyl-1H-1,2,3-triazole (4e) in 71% yield. When water was used as the solvent, the [3+2] cycloaddition reaction proceeded smoothly, with a significant increase in the chemical yield of 4e to 87% (Table 1).9
The scope of azides and acetylenes was examined for the Huisgen [3+2] cycloaddition reaction in water using PS-PEG-terpyridine Cu(I) 1. The representative results are summarized in Table 2. PS-PEG resin-supported terpyridine copper(I) complex 1 efficiently catalyzed the coupling of benzyl azide (2a) and n-nonanyl azide (2b) with dimethyl acetylenedicarboxylate (3a) to afford triazoles 4a and 4f in 82% and 54% yields, respectively (entries 1 and 6). The Huisgen [3+2] cycloaddition reaction of benzyl azide (2a) with various acetylenes 3b–3e proceeded to give the corresponding ethyl 1-benzyl-1H-1,2,3-triazole-4-carboxylate (4b), 1-benzyl-4-(phenoxymethyl)-1H-1,2,3-triazole (4c), (1-benzyl-1H-1,2,3-triazol-4-yl)methyl acetate (4d), and 1-benzyl-4-phenyl-1H-1,2,3-triazole (4e) in 77%, 59%, 71%, and 87% yields, respectively. The coupling reaction of alkyl azide 2b with various acetylenes 3b–3e also proceeded to give the corresponding ethyl 1-nonyl-1H-1,2,3-triazole-4-carboxylate (4g), 1-nonyl-4-phenoxy-1H-1,2,3-triazole (4h), (1-nonyl-1H-1,2,3-triazol-4-yl)methyl acetate (4i), and 1-nonyl-4-phenyl-1H-1,2,3-triazole (4j) in 67%, 45%, 44%, and 56% yields, respectively.
The recyclability of the PS-PEG-Terpyridine Cu(I) 1 was examined for the Huisgen [3+2] cycoaddition reaction of benzyl azide with phenylacetylene. Thus, after the first reaction, which gave 82% of dimethyl 1-benzyl-1H-1,2,3-triazole-4,5-dicarboxylate (4a), the catalyst was recovered by simple filtration, washed with water, dried under vacuum, and reused three times under similar reaction conditions to give 4e in 83%, 85%, and 85% yields (Scheme 2). After recycling experiments, ICP-AES analysis showed that leaching of Cu to the aqueous solution was 6 ppm.
In conclusion, we have developed a novel polymer-supported terpyridine-copper complex through ionic bonds to the sulfonate group, which efficiently catalyzed the Huisgen [3+2] cycoaddition reaction of benzyl or alkyl azides with acetylenes in water under aerobic conditions to give the corresponding triazoles in high yield and high regioselectivity. This catalyst was recovered and reused several times without any loss of catalytic activity. Further extensive scope of the Huisgen [3+2] cycoaddition reaction and application of this catalyst to the other organic transformations are in progress in our lab.
EXPERIMENTAL
General Methods:
All manipulations were carried out under aerobic conditions. Water was deionized with a Millipore system as Milli-Q grade. NMR spectra were recorded on a JEOL JNM-LA 500 spectrometer (500 MHz for 1H and 125 MHz for 13C). 1H and 13C NMR spectra were recorded in CDCl3 or DMSO at 25 °C. Chemical shifts were reported in ppm referenced to an internal tetramethylsilane standard for 1H NMR. Chemical shifts of 13C NMR were given relative to CDCl3 and DMSO as an internal standard (δ 77.0 ppm and 39.7 ppm). Mass spectral data were measured on a Shimadzu Qp2010 MS detector (GC-MS) and a Hitachi M-2500 (GC-MS) and JEOL JMS-T100LP (LC-MS); the abbreviation ‘bp’ is used to denote the base peak. ICP-AES spectral data were measured on a Shimadzu ICPE-9000.
Materials:
PS-PEG-supported catalyst 1 was prepared from PS-PEG amino-resin7 (Tenta Gel S NH2, average diameter 90 mm, 1% divinylbenzene cross-linked, loading value of amino residue 0.31 mmol/g; purchased from RAPP POLYMERE) and terpyridine ligand according to the reported procedures.10
Synthesis:
Preparation of 3-(4-formylphenoxy)propane-1-sulfonic acid (6). To a solution of p-hydroxybenzaldehyde (10.0 g, 82 mmol) in 2-propanol (100 mL) was added KOH (4.6 g, 82 mmol). The resulting mixture was stirred until it became homogeneous under ultrasonic wave irradiation conditions. To a reaction mixture was added propane sultone (10.0 g, 82 mmol) in 2-propanol (50 mL) and the reaction mixture was stirred at 100 °C until it became generating the solid. The generated solid was collected by filtration, washed with 2-propanol, and dried under vaccum to gave compound 6 in 84% yield (19.2 g), which was used directly in the next step.
Preparation of 3-(4-(terpyridin-4'-yl)phenoxy)propane-1-sulfonic acid (8). 3-(4-formylphenoxy)propane-1-sulfonic acid (6) (12.2 g, 50 mmol), acetamide (88.6 g, 1.5 mol), ammonium acetate (57.8 g, 0.75 mol) and 2-acetylpyridine (12.1 g, 0.10 mol) were stirred at 150 °C for 2 h. The reaction mixture was cooled until 40 °C and added NaOH (45 g, 1.1 mol) in H2O (200 mL) at 100 °C. The reaction mixture was stirred for overnight at 100 °C to generate the yellow solid. The solid was corrected by filtration, washed with H2O, and dried under vacuum to give a pale yellow solid. Purification of the pale yellow solid via recrystallization from H2O gave the 3-(4-(terpyridin-4'-yl)phenoxy)propane-1-sulfonic acid (8) in 82% yield (18.3 g, 41 mmol). 1H NMR (DMSO-d6) δ 8.78-8.77 (m, 2 H), 8.68-8.66 (m, 4 H), 8.03 (td, J = 7.5, 1.7 Hz, 2 H), 7.88 (d, J = 8.7 Hz, 2 H), 7.55-7.51 (m, 2 H), 7.13 (d, J = 8.7 Hz, 2 H), 4.17 (t, J = 6.8 Hz, 2 H), 2.64 (t, J = 7.3 Hz, 2 H), 2.52-2.51 (m, 1 H), 2.11-2.06 (m, 2 H): 13C NMR (DMSO-d6) δ 160.0, 155.7 (2C), 155.2 (2C), 149.5 (2C), 149.2, 137.6 (2C), 130.9, 129.5 (2C), 128.3 (2C), 124.6 (2C), 121.0 (2C), 117.4 (2C), 67.1, 48.0, 25.4. ESI-HRMS calcd for C24H21N3O4SNa 470.1150, found 470.1154.
Preparation of immobilized copper complex 1:
A Merrifield vessel was charged with PS-PEG-NH2 (0.80 g, 0.24 mmol), 8 (165 mg, 0.37 mmol), and DMF, and the reaction mixture was shaken on a shaking machine (CM-1000) at 25 °C for 1 h. Complete consumption of the primary amino residue of the resin was monitored by Kaiser negative test. The reaction mixture was filtered and the resin was washed with DMF and CH2Cl2. The resin was dried under reduced pressure to give the polymer-supported 3-(4-(terpyridin-4'-yl)phenoxy)propane-1-sulfonate (total loading: 0.24 mmol), which was used directly in the next step. A Merrifield vessel was charged with resin-supported 3-(4-(terpyridin-4'-yl)phenoxy)propane-1-sulfonate (loading value: 0.24 mmol) and 20 mL of CH2Cl2. To this suspension was added 53.2 mg of copper(I) iodine (0.28 mmol) and the mixture was shaken on a shaking machine (CM-1000) at 25 °C for 10 min. After filtration, the resin was washed with CH2Cl2 and dried under reduced pressure to give polymer-supported copper complex 1 (lording value: 0.26 mmol/g).
Copper-catalyzed Cycloaddition Reaction of benzyl azide with phenylacetylene:
To a mixture of the polymeric catalyst 1 (76 mg, 0.020 mmol) and benzyl azide (2a; 79.8 mg, 0.60 mmol) in H2O (3.0 mL) was added phenylacetylene (3e; 40.8 mg, 0.40 mmol). The reaction mixture was shaken at 40 °C for 6 h and filtered. The recovered resin beads were rinsed with H2O and extracted three times with EtOAc (6 mL). The EtOAc layer was separated and the aqueous layer was extracted with EtOAc (5 mL). The combined EtOAc extracts were washed with brine (2 mL), dried over MgSO4, and concentrated in vacuo. The resulting residue was chromatographed on silica gel (hexane-EtOAc, 10:1) to give 81.6 mg (87% yield) of 1-benzyl-4-phenyl-1H-1,2,3-triazole (4e). 1H NMR (CDCl3) δ 7.81-7.78 (m, 2 H), 7.66 (s, 1 H), 7.41-7.37 (m, 5 H), 7.33-7.30 (m, 3 H), 5.57 (s, 2 H): 13C NMR (CDCl3) δ 148.1, 134.6, 130.4, 129.0 (2C), 128.7, 128.7 (2C), 128.0 (2C), 127.9 (2C), 125.6, 119.4, 54.1. MS (EI): m/z (rel%) 235 (8, M+), 206 (30), 116 (bp), 91 (87).
Dimethyl 1-benzyl-1H-1,2,3-triazole-4,5-dicarboxylate (4a): 1H NMR (CDCl3) δ 7.35-7.32 (m, 3 H), 7.26-7.25 (m, 2 H), 5.81 (s, 2 H), 3.96 (s, 3 H), 3.87 (s, 3 H): 13C NMR (CDCl3) δ 160.3, 158.7, 140.1, 133.1, 129.1 (2C), 128.9 (2C), 128.8, 127.9, 53.9, 53.2, 52.6. MS (EI): m/z (rel%) 216 (2, M+ – COOMe), 214 (6), 174 (6), 156 (10), 130 (12), 91 (bp).
Ethyl 1-benzyl-1H-1,2,3-triazole-4-carboxylate (4b): 1H NMR (CDCl3) δ 8.02 (s, 1 H), 7.39-7.37 (m, 3 H), 7.30-7.28 (m, 2 H), 5.57 (s, 2 H), 4.38 (q, J = 7.0 Hz, 2 H), 1.37 (t, J = 7.0 Hz, 3 H): 13C NMR (CDCl3) δ 160.5, 140.4, 133.6, 129.1 (2C), 128.9 (2C), 128.1, 127.2, 61.1, 54.3, 14.1. MS (EI): m/z (rel%) 231 (1, M+), 202 (2), 174 (18), 130 (25), 91 (bp).
1-Benzyl-4-phenoxy-1H-1,2,3-triazole (4c): 1H NMR (CDCl3) δ 7.53 (s, 1 H), 7.38-7.35 (m, 3 H), 7.30-7.26 (m, 4 H), 6.96-6.95 (m, 3 H), 5.53 (s, 2 H), 5.19 (s, 2 H): 13C NMR (CDCl3) δ 158.1, 144.5, 134.2, 129.5 (2C), 129.1 (2C), 128.8, 128.1 (2C), 122.6, 121.2, 114.7 (2C), 61.9, 54.3. MS (EI): m/z (rel%) 265 (7, M+), 172 (13), 144 (41), 91 (bp).
(1-Benzyl-1H-1,2,3-triazol-4-yl)methyl acetate (4d): 1H NMR (CDCl3) δ 7.53 (s, 1 H), 7.37-7.35 (m, 3 H), 7.28-7.27 (m, 2 H), 5.52 (s, 2 H), 5.18 (s, 2 H), 2.05 (s, 3 H): 13C NMR (CDCl3) δ 170.7, 143.1, 134.3, 129.0 (2C), 128.7 (2C), 128.0, 123.5, 57.5, 54.1, 20.7. MS (EI): m/z (rel%) 231 (2, M+), 188 (18), 161 (10), 144 (6), 91 (bp).
Dimethyl 1-nonyl-1H-1,2,3-triazole-4,5-dicarboxylate (4f): 1H NMR (CDCl3) δ 4.58 (t, J = 7.3 Hz, 2 H), 4.00 (s, 3 H), 3.97 (s, 3 H), 1.92-1.86 (m, 2 H), 1.31-1.25 (m, 12 H), 0.87 (t, J = 6.8 Hz, 3 H): 13C NMR (CDCl3) δ 160.5, 159.0, 139.8, 129.8, 53.3, 52.6, 50.6, 31.7, 30.2, 29.2, 29.0, 28.8, 26.2, 22.5, 14.6. MS (EI): m/z (rel%) 280 (6, M+ – OMe), 252 (bp), 224 (25), 154 (37), 140 (37), 126 (27).
Ethyl 1-nonyl-1H-1,2,3-triazole-4-carboxylate (4g): 1H NMR (CDCl3) δ 8.09 (s, 1 H), 4.46-4.40 (m, 4 H), 2.07-1.90 (m, 2 H), 1.41 (t, J = 7.0 Hz, 3 H), 1.32-1.26 (m, 12 H), 0.87 (t, J = 7.0 Hz, 3 H): 13C NMR (CDCl3) δ 160.7, 140.1, 127.1, 61.1, 50.6, 31.6, 30.0, 29.1, 29.0, 28.8, 26.2, 22.5, 14.2, 13.9. MS (EI): m/z (rel%) 222 (16, M+ – OEt), 210 (14), 168 (45), 154 (62), 152 (75), 130 (50), 96 (83), 83 (bp).
1-Nonyl-4-phenoxy-1H-1,2,3-triazole (4h): 1H NMR (CDCl3) δ 7.59 (s, 1 H), 7.31-7.27 (m, 2 H), 7.00-6.95 (m, 3 H), 5.22 (s, 2 H), 4.34 (t, J = 7.0 Hz, 2 H), 1.91-1.89 (m, 2 H), 1.31-1.25 (m, 12 H), 0.87 (t, J = 7.0 Hz, 3 H): 13C NMR (CDCl3) δ 158.1, 144.0, 129.5 (2C), 122.4, 121.2, 114.7 (2C), 61.9, 50.6, 31.7, 30.2, 29.2, 29.1, 28.9, 26.4, 22.6, 14.0. MS (EI): m/z (rel%) 301 (13, M+), 180 (bp), 94 (51).
(1-Nonyl-1H-1,2,3-triazol-4-yl)methyl acetate (4i): 1H NMR (CDCl3) δ 7.60 (s, 1 H), 5.21 (s, 2 H), 4.34 (t, J = 7.31 Hz, 2 H), 2.08 (s, 3 H), 1.95-1.87 (m, 2 H), 1.32-1.26 (m, 12 H), 0.87 (t, J = 6.5 Hz, 3 H): 13C NMR (CDCl3) δ 170.8, 142.6, 123.4, 57.6, 50.3, 31.7, 30.1, 29.2, 29.0, 28.8, 26.3, 22.5, 20.8, 13.9. MS (EI): m/z (rel%) 267 (4, M+), 224 (37), 168 (35), 154 (87), 126 (41), 98 (39), 84 (bp).
1-Nonyl-4-phenyl-1H-1,2,3-triazole (4j): 1H NMR (CDCl3) δ 7.85-7.81 (m, 2 H), 7.74 (s, 1 H), 7.42 (t, J = 7.8 Hz, 2 H), 7.35-7.32 (m, 1 H), 4.40 (t, J = 7.3 Hz, 2 H), 1.97-1.92 (m, 2 H), 1.35-1.26 (m, 12 H), 0.87 (t, J = 7.0 Hz, 3 H): 13C NMR (CDCl3) δ 147.6, 130.6, 128.8 (2C), 128.0, 125.6 (2C), 119.3, 50.4, 31.7, 30.3, 29.3, 29.1, 28.9, 26.4, 22.6, 14.0. MS (EI): m/z (rel%) 271 (8, M+), 242 (8), 172 (20), 145 (29), 117 (bp), 104 (35).
ACKNOWLEDGEMENTS
We are grateful for financial support from the Young Scientists Program of University of the Ryukyus, and this work was the Joint Study in Cooperative Research Center.
References
1. R. Huisgen, In 1,3-Dipolar Cycloaddition Chemistry; ed. by A. Padwa; Wiley: New York, 1984.
2. For example, see: a) R. Alvarez, S. Velazque, F. San, S. Aquaro, C. De, C. F. Perno, A. Karlsson, J. Balzarini, and M. J. Camarasa, J. Med. Chem., 1994, 37, 4185; CrossRef b) K. J. Genin, D. A. Allwine, D. J. Anderson, M. R. Barbachyn, D. E. Emmert, S. A. Gamon, D. A. Allwine, D. J. Anderson, M. R. Barbachyn, D. E. Emmert, S. A. Garmon, D. R. Graber, K. C. Grega, J. B. Hester, D. K. Hutchinson, J. Morris, R. J. Reischer, C. W. Ford, G. E. Zurenko, J. C. Hamel, R. D. Schaadt, D. Stapert, and B. H. Yagi, J. Med. Chem., 2000, 43, 953; CrossRef c) A. Brik, Y.-C. Lin, J. Elder, and C.-H. Wong, Chem. Biol., 2002, 9, 891. CrossRef
3. For a review, see: H. C. Kolb, M. G. Finn, and K. B. Sharpless, Angew. Chem. Int. Ed., 2001, 40, 2004. CrossRef
4. For homogenously catalyzed [3+2] cycloaddition, see: a) N. Candelon, D. Lastécouères, A. K. Diallo, J. R. Aranzaes, D. Astruc, and J.-M. Vincent, Chem. Commun., 2008, 741; CrossRef b) S. Diez-González, A. Correa, L. Cavallo, and S. P. Nolan, Chem. Eur. J., 2006, 12, 7558; CrossRef c) L. Zhang, X. Chen, P. Xue, H. H. Y. Sun, I. D. Williams, K. B. Shapless, V. V. Fokin, and G. Jia, J. Am. Chem. Soc., 2005, 127, 15998; CrossRef d) P. Appukkuttan, W. Dehaen, V. V. Fokin, and E. van der Eycken, Org. Lett., 2004, 6, 4223; CrossRef e) T. R. Chan, R. Hilgraf, K. B. Sharpless, and V. V. Fokin, Org. Lett., 2004, 6, 2853; CrossRef f) H. S. G. Beckmann and V. Wittmann, Org. Lett., 2007, 9, 1; CrossRef g) B. Sreedhar and P. S. Reddy, Synth. Commun., 2007, 37, 805; CrossRef h) B. Gerard, J. Ryan, A. B. Beeler, and J. A. Porco Jr, Tetrahedron, 2006, 62, 6405; CrossRef i) Y.-B. Zhao, Z.-Y. Yan, and Y.-M. Liang, Tethrahedron Lett., 2006, 47, 1545; CrossRef j) B. -Y. Lee, S. R. Park, H. B. Jeon, and K. S. Kim, Tetrahedron Lett., 2006, 47, 5105; CrossRef k) K. Kamata, Y. Nakagawa, K. Yamaguchi, and N. Mizuno, J. Am. Chem. Soc., 2008, 130, 1530. CrossRef
5. For heterogeneously catalyzed [3+2] cycloaddition, see: a) B. H. Lipshutz and B. R. Taft, Angew. Chem. Int. Ed., 2006, 45, 8235; CrossRef b) I. S. Park, M. S. Kwon, J. S. Lee, and J. Park, Org. Lett., 2008, 10, 497; CrossRef c) M. L. Kantam, V. S. Jaya, B. Sreedhar, M. M. Raoa, and B. M. Choudary, J. Mol. Catal. A., 2006, 256, 273; CrossRef d) K. Rajender Reddy, K. Rajgopal, and M. L. Kantam, Catal. Lett., 2007, 114, 36; CrossRef e) S. Chassaing, M. Kumarraja, A. S. S. Sido, A. Alix, M. Kumarrajya, P. Pale, and J. Sommer, Chem. Eur. J. 2008, 14, 6713; CrossRef f) K. Kamitharan, M. Kumarraja, and K. Pitchumani, Chem. Eur. J., 2009, 15, 2755; CrossRef g) T. Katayama, K. Kamata, K. Yamaguchi, and N. Mizuno, ChemSusChem, 2009, 2, 59; CrossRef h) S. Uthaiwan, B. Y. Jin, L. B. Se, and C. D. Yoon, Synlett, 2008, 2326. CrossRef
6. C. Girard, E. Onen, M. Aufort, S. Beauvienre, E. Samson, and J. Herscovici, Org. Lett., 2006, 8, 1689. CrossRef
7. a) W. Rapp, In Combinatorial Peptide and Nonpeptide Libraries; ed. by G. Jung; VCH, Weilheim, 1996; p 425; CrossRef b) X. Du and R. W. Armstrong, J. Org. Chem., 1997, 62, 5678; CrossRef c) O. Gooding, S. Baudert, T. L. Deegan, K. Heisler, J. W. Labadie, W. S. Newcomb, J. A. Jr. Porco, and P. J. Eikeren, Comb. Chem., 1999, 1, 113. CrossRef
8. For a review, see: I. Eryazici, N. Moorefield, and R. Newkome, Chem. Rev., 2008, 108, 1834 and references therein. CrossRef
9. For a homogeneous system: Effect of the terpyridine ligand on the catalytic activity was studied by the reference reaction under similar reaction conditions in water using the 8-Cu(I) complex to give the corresponding product 4e in 95% isolated yield.
10. W. Spahni and G. Calzaferri, Helv. Chim. Acta, 1984, 67, 450. CrossRef