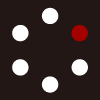
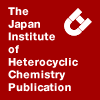
HETEROCYCLES
An International Journal for Reviews and Communications in Heterocyclic ChemistryWeb Edition ISSN: 1881-0942
Published online by The Japan Institute of Heterocyclic Chemistry
e-Journal
Full Text HTML
Received, 26th November, 2009, Accepted, 22nd January, 2010, Published online, 25th January, 2010.
DOI: 10.3987/COM-09-S(E)1
■ Nucleophilic Phosphine-Catalyzed Iodocyclization of Isoprenoids Bearing an Oxygen Terminal Group
Akira Sakakura, Gakujun Shomi, Atsushi Ukai, and Kazuaki Ishihara*
Graduate School of Engineering, Nagoya University, Chikusa, Nagoya, Aichi 464-8601,
Abstract
The nucleophilic phosphine-catalyzed diastereoselective iodocyclization of linear isoprenoids bearing an oxygen terminal group was investigated. TBDMS ether of homogeraniol and TBDMS ester of homogeranic acid were successfully converted to the corresponding iodopolycyclic products in the presence of a catalytic amount of triphenylphosphine with complete diastereoselectivity.Diastereoselective halocyclizations induced by the electrophilic addition of a halonium ion to an unactivated alkene are powerful tools in organic synthesis.1,2 Since these halocyclizations are still conducted under harsh reaction conditions with more than stoichiometric amounts of reagents, the development of effective catalysts that can promote halocyclizations under mild conditions is in high demand. In this context, we recently developed a catalytic diastereoselective iodocyclization of isoprenoids bearing an aryl terminal group.3 Catalytic amounts of nucleophilic phosphorous(III) compounds activate N-iodosuccinimide (NIS) and react with the terminal carbon-carbon double bond of 4-homogeranyltoluene (1), which induces diastereoselective cyclization to give 2 in excellent yield (Scheme 1).4 Highly nucleophilic phosphorous(III) compounds such as tributylphosphine (PBu3) show good catalytic activities in CH2Cl2. During the course of our study, we found that the reactivity of nucleophilic phosphine-catalyzed iodocyclization significantly depended on the structure and nucleophilicity of the terminal functional group. We report here the nucleophilic phosphine-catalyzed iodocyclization of isoprenoids bearing an oxygen terminal group.
We chose 4-substituted 4-pentenoic acids 3 as model substrates for iodolactonization.5 As a terminal group, tert-butyldimethylsilyl (TBDMS) ester would be suitable. As shown in Scheme 2, the TBDMS ester group of 3a would react with the iodonium ion moiety of 5a, and the TBDMS group would be spontaneously trapped by the succinimide anion to give iodolactone 4a. According to our working hypothesis, we first examined the catalytic activity of PPh3 toward the 5-exo-trig iodolactonization of 3a (Table 1). The reaction was conducted with 1.2 equiv. of NIS in toluene at –40 °C. The catalytic activity highly depended on the amount of triphenylphosphine (PPh3). Very interestingly, the best result (54% yield) was obtained when 30 mol% of PPh3 was used (entry 4). The use of larger amounts (40–100 mol%) of PPh3 decreased the yield of 4a (entries 5–7). Interestingly, the catalytic activities of highly nucleophilic PBu3 and tri(cyclohexyl)phosphine [P(c-C6H11)3] were quite low (entries 8 and 9) in contrast to the iodocyclization of 4-homogeranyltoluene (Scheme 1). Triphenyl phosphite [P(OPh)3] showed a slightly higher activity than PPh3 (entry 10).
ased on our preliminary work, it is conceivable that the reactivity of 3 depends on the terminal functional group. The reactivity of tert-butyl ester 3b was slightly lower than that of TBDMS ester 3a. The reaction of 3b proceeded at –20 °C and gave 4b in 49% yield (entry 11). We next examined the catalytic iodolactonization of TBDMS ester of 4-substituted 4-pentenoic acids 3c–3f. The reaction was conducted with NIS (1.2 equiv) in the presence of PPh3 (30 mol%) in toluene. Compound 3c (R = H), which has a monosubstituted carbon–carbon double bond, showed low reactivity and gave 4b in 34% yield, even though the reaction was conducted at 0 °C (entry 12). In contrast, compounds 3d–3f, which have 1,1-disubstituted carbon–carbon double bonds, were successfully converted to 4d–4f at –40 °C (entries 13–15). In particular, compound 3d bearing a less bulky methyl group at the 4-position showed good reactivity (entry 13). The high nucleophilicity of the carbon–carbon double bond in 3 would be important for obtaining 4 in high yield.
Although it is unclear why the use of large amounts of PPh3 decreased their activity, we can propose the explanation in Scheme 3. When the reaction was conducted in the presence of 40–100 mol% of PPh3, PPh3 might attack the iodonium ion moiety of 5a to give phosphonium ion intermediate 6a, which would be converted back to 3a by aqueous workup (path a).6,7 Alternatively, the equilibrium between 3a and 5a might strongly favor the regeneration of 3a in the presence of large amounts of PPh3 (path c). In contrast, the intramolecular cyclization of 5a successfully proceeded in the presence of 30 mol% of PPh3 (path b), as shown in Scheme 2. The finding that highly nucleophilic phosphorous compounds showed low catalytic activities might also be explained by this mechanism.
Many halogenated polycyclic terpenoids possessing a cyclic ether structure have been isolated from various marine organisms.8 For example, the cytotoxic substance aplysistatin was isolated from the South Pacific Ocean sea hare Aplysia angasi.9 Luzonenone and luzofuran were isolated from the red alga Laurencia luzonensis.10 The biosynthesis of these natural products appears to include the halonium ion-induced polycyclization of linear isoprenoids bearing terminal oxygen groups.11 Catalytic diastereoselective iodocyclization is one of the most promising methods for the chemical synthesis of these polycyclic natural compounds, since polycyclic iodoterpenoids can be converted to other halogenated derivatives by stereospecific transhalogenation.3 Therefore, we next examined the nucleophilic phosphine-catalyzed iodocyclization of 9 and 10 (Scheme 4).
First, the catalytic iodoetherification of O-protected homogeraniol 9 was examined (Table 2). The reaction was conducted with NIS in the presence of PPh3 (30 mol%) in CH2Cl2.12 When the reaction of TBDMS ether 9a was conducted with 1.2 equiv. of NIS, the desired trans-fused dicyclic product 7 was obtained in 35% yield as a single diastereomer (entry 1). To improve the yield of 7, the reaction was conducted with 2.0 equiv. of NIS. As a result, 7 was successfully obtained in 68% yield along with a small amount of monocyclic product 11a (15%) (entry 2). The reaction of p-methoxyphenyl (PMP) ether 9b also proceeded to give monocyclic product 11b, which was easily converted to 7 (52%, 3 steps from 9b) by oxidative deprotection of the PMP group [Ce(NH4)2(NO3)6] followed by Brønsted acid-promoted cyclization (SnCl4, ClSO3H) (entry 3). The iodolactonization of homogeranic acid esters 10 was also catalyzed by triphenylphosphine to give the corresponding iodocyclic product 8 as a single diastereomer. As in the case of 3, TBDMS ester 10a was more reactive than tert-butyl ester 10c and gave 8 in 47% yield along with monocyclic product 12a (11%) (entries 4 and 5).
In conclusion, we investigated nucleophilic phosphine-catalyzed diastereoselective iodocyclization with oxygen terminal groups.13 For the 5-exo-trig iodolactonization of TBDMS esters of 4-substituted 4-pentenoic acids 3, 30 mol% of triphenylphosphine showed moderate catalytic activity under mild reaction conditions (toluene, –40 °C). TBDMS ether of homogeraniol and TBDMS ester of homogeranic acid were successfully converted to the corresponding iodopolycyclic products in the presence of a catalytic amount of triphenylphosphine with complete diastereoselectivity.
ACKNOWLEDGEMENTS
Financial support for this project was provided by MEXT.KAKENHI (20245022), the Toray Science Foundation, the Global COE Program of MEXT, and JSPS Research Fellowships for Young Scientists (A.U.).
References
1. For recent reviews, see: (a) M. S. Laya, A. K. Banerjee, and E. V. Cabrera, Curr. Org. Chem., 2009, 13, 720; CrossRef (b) A. N. French, S. Bissmire, and T. Wirth, Chem. Soc. Rev., 2004, 33, 354; CrossRef (c) S. Robin and G. Rousseau, Tetrahedron, 1998, 54, 13681. CrossRef
2. For selected recent reports of catalytic halolactonization and haloetherification, see: (a) Z. Ning, R. Jin, J. Ding, and L. Gao, Synlett, 2009, 2291; CrossRef (b) T. A. Doroski, M. R. Cox, and J. B. Morgan, Tetrahedron Lett., 2009, 50, 5162; CrossRef (c) H. Y. Kwon, C. M. Park, S. B. Lee, J.-H. Youn, and S. H. Kang, Chem. Eur. J., 2008, 14, 1023; CrossRef (d) S. M. Ahmad, D. C. Braddock, G. Cansell, and S. A. Hermitage, Tetrahedron Lett., 2007, 48, 915. CrossRef
3. (a) A. Sakakura, A. Ukai, and K. Ishihara, Nature, 2007, 445, 900; CrossRef (b) A. Sakakura and K. Ishihara, Chimica Oggi–Chemistry Today, 2007, 25, 9.
4. For a recent review of Lewis base catalysis, see: S. E. Denmark and G. L. Beutner, Angew. Chem. Int. Ed., 2008, 47, 1560. CrossRef
5. (a) J. M. Gernier, S. Robin, and G. Rousseau, Eur. J. Org. Chem., 2007, 3281; CrossRef (b) J. Haas, S. Piguel, and T. Wirth, Org. Lett., 2002, 4, 297; CrossRef (c) R. B. Grossman and R. J. Trupp, Can. J. Chem., 1998, 76, 1233. CrossRef
6. Compound 3a might be regenerated from 6a through the nucleophilic attack on the iodine atom by OH–, S2O32– or something else.
7. 31P NMR analysis of a 1:1:1 mixture of 3a, NIS and PPh3 did not show the generation of 6a but only small amount (ca. 10%) of phosphonium salt I–PPh3+. The intermediate 6a might be too moisture sensitive to be detected by NMR analysis.
8. J. W. Blunt, B. R. Copp, W.-P. Hu, M. H. G. Munro, P. T. Northcote, and M. R. Prinsep, Nat. Prod. Rep., 2009, 26, 170. CrossRef
9. G. R. Pettit, C. L. Herald, M. S. Allen, R. B. Von Dreele, L. D. Vanell, J. P. Y. Kao, and W. Blake, J. Am. Chem. Soc., 1976, 99, 262. CrossRef
10. M. Kuniyoshi, P. G. Wahome, T. Miono, T. Hashimoto, M. Yokoyama, K. L. Shrestha, and T. Higa, J. Nat. Prod., 2005, 68, 1314. CrossRef
11. A. Butler and J. N. Carter-Franklin, Nat. Prod. Rep., 2004, 21, 180. CrossRef
12. General procedure for the iodocyclization of homogeraniol derivatives 9 and homogeranic acid derivative 10: To a solution of triphenylphosphine (30 mol%) and 9 or 10 (0.10 mmol) in CH2Cl2 (1 mL) was added NIS (2.0 equiv.) at –78 °C, and the mixture was stirred at –78 °C for 0.5 h and then at –40 °C for 24 h. The reaction mixture was quenched with Na2S2O3 (3 mL) and extracted with EtOAc (5 mL × 3). The combined organic layer was dried over Mg2SO4, filtered and concentrated in vacuo. The crude product was purified by column chromatography on silica gel with a 30:1 mixture of hexane–EtOAc. 7: oil; IR (neat) 2872, 1455, 1376, 1140, 1064, 1008 cm–1; 1H NMR (300 MHz, CDCl3) δ 0.97 (s, 3H), 1.04 (s, 3H), 1.13 (s, 3H), 1.42–1.62 (m, 2H), 1.73 (m, 1H), 1.8–2.0 (m, 2H), 2.14–2.31 (m, 1H), 2.37–2.45 (m, 1H), 3.52–3.66 (m, 2H), 4.08 (dd, J = 4.2, 12.9 Hz, 1H); 13C NMR (100 MHz, CDCl3) δ 19.6, 20.0, 25.7, 32.5, 35.5, 38.7, 40.9, 48.9, 54.3, 64.6, 78.9; HRMS (EI) calcd for C11H19IO (M+) 294.0481, found 294.0499. 8: oil; IR (neat) 1772, 1457, 1387, 1188, 1132 cm–1; 1H NMR (300 MHz, CDCl3) δ 1.04 (s, 6H), 1.39 (s, 3H), 1.78–1.92 (m, 2H), 2.09–2.59 (m, 5H), 4.08 (dd, J = 4.8, 12.9 Hz, 1H); 13C NMR (100 MHz, CDCl3) δ 19.6, 20.4, 30.8, 32.0, 34.8, 38.2, 39.9, 44.7, 52.8, 84.8, 175.2; HRMS (EI) calcd for C11H17IO3 (M+) 308.0273, found 308.0285.
13. Triphenylphosphine could not be recovered because it was oxidized to triphenylphosphine oxide by unreacted NIS through aqueous workup.