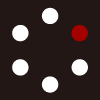
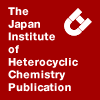
HETEROCYCLES
An International Journal for Reviews and Communications in Heterocyclic ChemistryWeb Edition ISSN: 1881-0942
Published online by The Japan Institute of Heterocyclic Chemistry
e-Journal
Full Text HTML
Received, 29th November, 2009, Accepted, 15th January, 2010, Published online, 18th January, 2010.
DOI: 10.3987/COM-09-11876
■ A Novel Tetra(tetrathiafulvalene-Thiacrown Ether)-Substituted Porphyrazine: Synthesis, Electrochemical, and Control Aggregation in Solution by Complexation of Transition-Metal Ions
Ruibin Hou, Cuiping Jiang, and Bingzhu Yin*
Key Laboratory of Organism Functional Factor of Changbai Mountain, Ministry of Education, Yanbian University, 977, Park Road, Yanji City, Jilin Prov. 133002, China
Abstract
Synthesis of a novel magnesium porphyrazine 6,peripherally substituted with S4 crown ether-TTF macrocycles was described. Molecular structure has been fully characterized by 1H-NMR, IR, MS, and elemental analysis. Crystal structure of the precursor was determined by X-ray crystallography. Their solution electrochemical data showed two reductive and three oxidative processes within a -1.3 V to -1.5 V potential window. UV/Vis spectroscopy and electron paramagnetic resonance (EPR) measurements revealed the formation of a charge-transfer complex between porphyrazine 6 with F4TCNQ. UV/vis spectroscopy showed that addition of the transition-metal ions led to dimerization of the porphyrazine complexes.INTRODUCTION
Porphyrazines (Pzs) is porphyrin macrocycles in which the meso positions contain nitrogens, not CH, and are of potential interest for excellent optical and electrical properties.1-3 These properties may be modulated by central metals and a variety of substitutions attached to the Pz cores.4-10 By controlling such parameters, diverse functionalities can be obtained. For example, Pzs containing macrocycles such as crown ethers show potential applications as ion conducting channel or heavy metal extraction agents.11-16 Of particular interest are porphyrazines fused with four thiacrown ether rings, which were reported independently by the research groups of Nolte and co-workers.17–19 These compounds display solvent and transition-metal ions induce aggregation behavior in CHCl3-MeOH solution. Interestingly, the selective metal complexation of the thiacrowned Pzs can lead to a specific response, e.g. a change in electrical conductivity.
Tetrathiafulvalene (TTF) is a well-known redox-active compound, the derivatives of which have been extensively studied during the last thirty-five years, mainly in the search for new molecular conductors and superconductors. The potential of TTF within the wider context of supramolecular chemistry is now recognised and new, more elaborate TTF and multi-TTF systems have been assembled. In order to make functional Pz-based aggregation, TTF units have been incorporated into a crown-ether-Pz building block to give 6, with the aim of endowing the resulting self-assembled material with potentially interesting electronic and structural properties. Synthesis of target compound 6 was shown in Scheme 1.
RESULTS AND DISCUSSION
Compound 1, obtained according to the reference,20 was deprotected by treatment with cesium hydroxide in a mixture of DMF and MeOH and the derived thiolate ions was reacted with excess 2-bromoethanol to give the compound 2 in 74% yield. Tosylation of 2 afforded 3, latter was converted to then bis-iodo intermediate 4 by a Finkelstein reaction with sodium iodide in acetone. Compound 4 was then reacted with an equivalent amount of cis-1,2-dicyano-1,2-ethylenedithiolate disodium salt in DMF to give the TTF-dicyano-tetrathiacrown ether 5 under argon in good yield (41%). The high-dilution technique accounts for the high yield obtained in the cyclization reaction.21 In CDCl3, the 1H-NMR spectrum of compound 5 displayed well-defined resonance signals in the crown ether and aliphatic units. The presence of C≡N stretching vibrations at 2210.42 cm-1 in the IR spectrum of this compound also confirmed the formation of 5. The MALDI-TOF mass spectrum of compound 5 displayed the [M+, 100%] parent ion peak at m/z 638.0601. The elemental analyses data were also satisfactory.
Red crystals suitable for an X-ray diffraction study of the key precursor 5 was obtained by slowly evaporating a CH2Cl2/pet. ether (60-90 oC) solution of 5 at room temperature. This compound crystallized in the monoclinic space group (P21/c) and an ORTEP plot of the molecule with the atomic numbering as shown in Figure 1 in which four S atoms and eight C atoms formed a 12-membered ring with boat conformation.
Target compound 6 was prepared by a magnesium templated macrocyclization of 5 in n-BuOH in 28% yield (Scheme 1), which was soluble in usual organic solvents except for methanol solvent. Tetramerization of 5 into Pz 6 was confirmed by the disappearance of the sharp C≡N stretching vibration in the IR spectra at 2210.42 cm-1 of the precursor. The MALDI-TOF-MS spectra of 6 featured peak at m/z 2580.8326, corresponding to M+ (2580.7021) of 6. In the 1H-NMR spectrum of 6, the characteristic signals relating to the –CH2S– protons of the crown ether units and alkyl group linked with the TTF skeleton gave significant resonances characteristic of the proposed structure. The UV-vis spectrum of 6 in CH2Cl2 showed a quite broadened Q-band maximum at around 675 nm. In the ultraviolet region, the B-band (or Soret band) of the Mg-Pz ring led to superimposed bands with the absorption of TTF. Broadening of the Q- and B-bands should be attributed to n→π* transitions of nonbonding electrons of peripheral S and N atoms and overlapping of B-band.
To further address the electron donating property of the target compounds, compound 6 was doped with an excess TCNQ in CH2Cl2. But no CT band was observed in the 600–1000 nm region, which might be attributed to the presence of the electron-withdrawing Pz ring. Whereas, upon 4 equiv. of F4TCNQ to a CH2Cl2 solution of 6 (1×10-5 M) resulted in the appearance of two new CT absorption bands, centered on λmax 759 nm and λmax 863 nm in the UV–Vis spectrum (Figure 2a). These new bands corresponded to the SOMO-LUMO transition of the cation radical species of the TTF moieties.22 The formation of the F4TCNQ-/TTF+ charge-transfer complex was also confirmed by the ESR spectra of compound 6 in CH2Cl2 at 25 oC centered around g = 2.0078 and 2.00176, which were in the region characteristic for both a TTF radical cation23 and a F4TCNQ radical anion.24 These results demonstrated that some CT took place between the TTF unit(s) and F4TCNQ in solution (Figure 2b).
The electrochemical characterization of compounds 6 was carried out by using cyclic and differential pulse voltammograms in a mixture of CH2Cl2–CH3CN (9:1, v/v) (Figure 3a and 3b). Compound 6
showed redox processes at E1/2 =-1.177 V (I), -0.937V (II), +0.544 V (III), +0.876 V (IV) and +1.189 V versus SCE (Figure 3b). The five couples observed were assigned to Pz-3/Pz-4 (I), Pz-2/Pz-3 (II), TTF+/TTF (III), TTF+2/ TTF+ (IV) and Pz-1/Pz-2 (V) on the basis of results in the literature.25 Processes I and II were irreversible in terms of the ratio of anodic to Cathodic peak currents. Processes III–V were quasi-reversible because the anodic to cathodic peak currents were near unity even though the ΔE values (for III, ΔE = 0.128 V, for IV, ΔE = 0.123 V and for V, ΔE = 0.093 V, respectively) were greatly beyond the 0.060 V scope.
The presence of tetrathia crown in porphyrazine 6 afforded the possibility of binding transition-metal ions. Addition of silver (I) or mercury (II) perchlorate to solutions of the porphyrazine in a mixtute of CH2Cl2-MeOH caused the absorption spectra of the ligands 6 to change. Some of the spectra that were recorded during its titration with AgClO4 are shown in Figure 4. The complex stoichiometry appeared to be different for the Ag+ (Figure 4, insert). Addition of AgClO4 first caused a decrease and broadening of the Q-band up to a Mg-porphyrazine 6 ratio of ca 2.5. At least four isosbestic points were present during this stage of the titration. Hereafter, a decrease of the Q-band absorption took place up to a metal-porphyrazine ratio of ca. 5. Further addition of Ag+ did not change the spectrum. Hg2+ caused a gradual decrease of the Q-band absorption during titration, Hg(ClO4)2 gave virtually the same results, except that the second peak-shift beyond 4 equivalents was less distinctive. The spectroscopic studies and previous studies11 suggested that dimers were formed. The complexation behavior of the 6 toward silver ions can be explained as following: the silver ion did not fit in the cavity of the tetrathia-12-crown-4 macrocycle and one coordination site of the ion was connected to a neighboring ligand, where the silver ions can form bridges between the two molecules. At the same time the n-π* transition at 496nm disappeared, probably because of complexation of the metal ions to the sulfur atoms.
In summary, we developed an efficient synthetic route to the formation of novel porphyrazine containing tetrathiacrown ether-linked TTF moieties. The ability of compound 6 to function as a donor for F4TCNQ was established, and the formation of TTF+/F4TCNQ- charge-transfer complex was confirmed by the UV-Vis and ESR spectroscopy. The compound 6 exhibited good Ag+ selectivity by forming a stable dimeric structure. The dimerization led to significant changes in the absorption spectral features. It is possible that the size of the cavity between the porphyrazine rings in the dimeric structure can be tuned. The electrical conductivity of the dimers induced by cations is now under investigation.
EXPERIMENTAL
NMR spectra were recorded in CDCl3 with a Bruker AV-300 Spetrometer (300 MHz for 1H and 75 MHz for 13C), and chemical shifts were referenced relative to tetramethylsilane (δH/δC = 0). The UV-VIS spectra were recorded on a Hitachi U-3010 spectrophotometer in CH2Cl2 (c = 1 × 10-5M). The MALDI-TOF-MS data were obtained by a Shimadzu AXIMA-CFRTM plus mass spectrometry, using a 1,8,9-anthra cenetriol (DITH) matrix. Cyclic voltammetry was carried out on a Potentiostat/Galvanostat 273A instrucment in MeCN-CH2Cl2 (1:9, v/v) and 0.1M Bu4PF6 as the supporting electrolyte. Counter and Working electrodes were made of Pt and Glass-Carbon, respectively, and the reference electrode was calomel electrode (SCE). IR spectra were recorded on a Shimadzu IRPrestige-21 FT-IR spectrophotometer (KBr pressed disc method). Starting materials 1 was prepared according to reference.20
The crystal data for C22H26N2S10 (5) was recorded on a Rigaku SCXmini diffractometer. M = 639.05, Monoclinic, P 21/c, a = 13.659(3) Å, b = 9.4722(19) Å, c = 22.875(5) Å, β = 97.61(3)°, V=2933.52 (10) Å3, Z = 4, Dc = 1.447Mg/m3, X-ray source Mo Kα (radiation), λ = 0.71073 Å, F (000) = 1328, T = 293(2) K, R1 = 0.077, wR = 0.258. Crystallographic data (excluding structure factor) for the structure in this paper have been deposited with the Cambridge Crystallographic Data Center as a supplementary publication number CCDC 760920. Copies of the Cambridge Crystallographic Data can be obtained, free of charge, on application to CCDC, 12 Union Road, Cambridge CB2 1EZ, UK (fax: +44(0)-1223-336033 or e-mail:deposit@ccdc.cam.uk).
2,3-Bis(2-hydroxyethylthio)-6,7-bis(butylthio)tetrathiafulvalene (2)
To a solution of 1 (1 g, 1.82 mmol) in DMF (32 mL) was added a solution of CsOH·H2O (0.67 g, 3.64 mmol) in MeOH (5 mL) over a period of 10 min. The mixture was stirred for 15 min and 2-bromoethanol (0.6 g, 4.73 mmol) was added. After stirring for 2 h. the solvent was removed in vacuo. The residue was dissolved in CH2Cl2 (50 mL) and washed with H2O. After drying (MgSO4) the solvent was removed and the product was subjected to column chromatography (silica gel. CH2Cl2/EtOAc, 1:1 to 1:2). The broad orange band was collected to give 2: yield: 0.73 g (75%); mp 72.41 ºC (by DTA). 1H NMR (CDC13): δ 0.93 (t, J = 7.5 Hz, 6H), 1.39-1.51 (m, 4H), 1.59-1.68 (m, 4H), 2.83 (t, J = 7.2 Hz, 4H), 2.92 (s, 2H), 3.01 (t, J = 5.4 Hz, 4H), 3.76 (t, J = 5.4 Hz, 4H); 13C NMR (CDCl3): δ 128.17, 127.80, 112.56, 108.14, 59.96, 39.26, 36.01, 31.75, 21.65, 13.62; IR (cm-1): 3462.22, 2953.02, 2870.08, 1631.78, 1220.94, 1043.46; MALDI-TOF m/z 532.1 (100%, M+); Anal. Calcd for C18H28OS8: C, 40.57; H, 5.30. Found: C, 40.59; H, 5.33.
2,3-Bis(2-tosyloxyethylthio)-6,7-Bis(butylthio)tetrathiafulvalene (3)
To a solution of 2 (0.6 g, 1.13 mmol) in CH2Cl2 (30 mL) was added tosyl chloride (0.65 g, 3.38 mmol). The solution was cooled to 0 oC and Et3N (0.6 mL, excess) was added dropwise. After addition the cooling bath was removed and the mixture was stirred overnight at 20 oC. The solvent was removed in vacuo and the residue subjected to column chromatography on silica gel with CH2Cl2 and pet.ether (60-90 oC) (9/1, v/v). Collection of the orange band afforded 3 as an orange solid: yield: 0.81g (85.2%); mp 75.74 ºC (DTA). 1H NMR (CDC13): δ 0.93 (t, J = 7.5Hz,6H), 1.39-1.51 (m, 4H), 1.59-1.68 (m, 4H), 2.47 (s, 6H), 2.84t, J = 7.2 Hz, 4H), 3.01 (t, J = 6.9 Hz, 4H), 4.15 (t, J = 6.9 Hz, 4H), 7.37 (d, J = 8.10 Hz, 4H), 7.81 (d, J = 8.10 Hz, 4H); 13C NMR (CDCl3): δ 145.19, 132.65, 130.03, 127.98, 67.85, 36.08, 34.41, 31.76, 21.74, 21.63, 13.61; IR (cm-1): 2956.87, 2922.16, 1361.74,1174.65, 964.41; MALDI-TOF m/z 840.6 (100%, M+). Anal. calcd for C32H40O6S10: C, 45.68; H, 4.79. Found: C, 45.72; H, 4.83.
2,3-Bis(2-iodoethylthio)-6,7-bis(butylthio)tetrathiafulvalene (4)
A mixture of 3 (0.35 g, 0.42 mmol) and NaI (1.26 g, 8.4 mmol) acetone was refluxed for 2 h. The solvent was removed in vacuo and the residue was dissolved in CH2Cl2 (20 mL). The CH2Cl2 layer was washed with H2O (25 mL) and dried (MgSO4). The solvent was removed and the residue subjected to column chromatography on silica gel with CH2Cl2 and pet.ether (60-90 oC) (1:1, v/v). Collection of the orange band afforded 3 as an red solid yield: 0.295 g (93%); mp 84.59 ºC (DTA). 1H NMR (CDC13): δ 0.95( t, J = 7.5 Hz, 6H), 1.39-1.51 (m, 4H), 1.59-1.68 (m, 4H), 2.85 (t, J = 7.2 Hz, 4H), 3.25 (t, J = 6.1 Hz, 4H), 3.35 (t, J = 5.4, 6.1 Hz, 4H); 13C NMR (CDCl3): δ 128.42, 127.95, 38.53, 36.88,36.13, 31.82, 21.67, 13.64, 2.03; IR (cm-1): 2922.16, 1432.1, 1211.05; MALDI-TOF m/z: 752.1. Anal. Calcd for C32H40O6S10: C, 28.72; H, 3.48. Found: C, 28.76; H, 3.51.
(Z)-2-(4,5-Bis(butylthio)-1,3-dithiol-2-ylidene)-5,6,11,12-tetrahydro[1,3]dithiolo[4,5-b][1,4,7,10]tetrathiacyclododecine-8,9-dicarbonitrile (5)
A solution of 6,7-Bis(n-butylthio)-2,3-bis(2-iodopropylthio)tetrathiafulvalene 4 (500 mg, 0.664 mmol) and cis-1,2-dicyano-1,2-ethylenedithiolate disodium salt (0.125 g, 0.664 mmol) in purified DMF (220 mL), then the mixture was transferred to a dropping funnel. Under high dilution condition, the solution was introduced into a reaction flask containing DMF (150 mL) under an atmosphere of Ar and heated to 80-90 oC. Dropwise over 3hrs started to form and the mixture was stirred for an additional 24 h and then allowed to cool to rt. The solution of DMF was removed in vacuum. The residue was dissolved in CH2Cl2 (15 mL), washed with water (100 mL), dried (MgSO4), and concentrated under reduced pressure. Purification by column chromatography on silica gel with CH2Cl2 and pet.ether (1:1, v/v) as an eluent to a red oil that solidified on standing in vacuo; yield 174.53 mg (41.1%); mp 127 ºC (by DTA). 1H NMR (CDC13): δ 0.94 (t, J = 7.3 Hz, 6H), 1.39-1.51 (m, 4H), 1.59-1.68 (m, 4H), 2.84 (t, J = 7.2 Hz, 4H), 3.25-3.28 (m, 4H), 3.32-3.34 (m, 4H) 13C NMR (CDCl3): δ 129.03, 127.83, 124.36, 112.56, 38.02, 36.07, 33.68, 31.74, 21.65, 13.62; MALDI-TOF m/z 638.06 (100%, M+), IR (cm-1): 2953.02, 2924.09, 2856.58, 2210.42, 1415.75, 1274.95, 1259.52. Anal. Calcd for C22H26N2S10: C, 41.34; H, 4.10; N, 4.38. Found: C, 41.38; H, 4.15; N, 4.36.
Mg TTF-Crown Ether Porphyrazine (6)
Magnesium (17 mg, 0.71 mmol) in small pieces was added in 3 mL of dry n-BuOH under Argon and the solution was heated to reflux. A small chip of solid I2 used as initiator was added to the solution. After metal magnesium was dissolved in n-BuOH, the compound 5 (90.6 mg, 0.142 mmol) was added and the mixture was refluxed for 12 h (at 110-120 oC) under Argon. The solution color changed from purplish red to deep green. The reaction mixture was cooled to room temperature. The solution was then decanted, CH2Cl2 (20 mL) was added and the organic layer was washed with water (3 x 50 mL). The organic layer was dried over MgSO4 and the solvent was removed. The residue was purified by column chromatography (silica gel, eluent: CH2Cl2-MeOH, 200/1~100/1) to dark green solid; yield 25.65 mg (28%); mp > 250 oC (by DTA). 1H NMR (CDCl3/TMS): δ 0.9 (t, J = 6.9 Hz, 24H), 1.37-1.44 (m, 16H), 1.53-1.62 (m, 16H), 2.65 (br,16H), 3.37 (br,16 H), 4.23 (br, 16H); 13C NMR (CDCl3, 75 MHz): δ 157.81, 141.07, 140.33, 127.67, 111.32, 108.80, 36.70, 35.83, 34.58, 31.64, 21.59, 13.61; IR (cm-1): 2953.02, 2922.16, 1699.29, 1454.33, 1265.30, 1219.01, 1012.63.MALDI-TOF-MS m/z 2580.83 (100%, M+); UV/vis (CH2Cl2): λmax: 678 (ε = 64700), 619 (ε = 23600, sh), 377 (ε = 68000), 332 (ε = 82000). Anal. Calcd for C88H104MgN8S40: C, 40.96; H, 4.06; N,4.34. Found: C, 40.92; H, 4.11; N, 4.31.
ACKNOWLEDGEMENTS
This work was supported by National Science Foundation of China (No.20662010), the Specialized Research Fund for the Doctoral Program of Higher Education (Grant No. 20060184001) and the open project of State Key Laboratory of Supramolecular Structure and Materials, Jilin University.
References
1. T. H. Tran-Thi, Coord. Chem. Rev., 1997, 160, 53. CrossRef
2. T. Toupance, V. Ahsen, and J. Simon, J. Am. Chem. Soc., 1994, 116, 5352. CrossRef
3. K. K. Dailey, G. P. A. Yap, and A. L. Rheingold, Angew. Chem., Int. Ed. Engl., 1996, 35, 1833. CrossRef
4. M. Polat and A. Gül, J. Chem. Res(S)., 1999, 130.
5. P. A. Stuzhin, E. M. Bauer, and C. Ercolani, Inorg. Chem., 1998, 37, 1533. CrossRef
6. E. M. Bauer, D. Cardarilli, and C. Ercolani, Inorg. Chem., 1999, 38, 6114. CrossRef
7. E. M. Bauer, C. Ercolani, and P. Galli, J. Porph. Phthalocyanine., 1999, 3, 371. CrossRef
8. C. S. Wang, M. R. Bryce, A. S. Batsanov, and J. A. K. Howard, Chem. Eur. J., 1997, 3, 1679. CrossRef
9. M. M. Toyama, G. J. F. Demets, and K. Araki, Electrochem. Commun., 2000, 2, 749. CrossRef
10. D. P. Goldberg, S. L. J. Michel, and A. J. P. White, Inorg. Chem., 1998, 37, 2100. CrossRef
11. C. F. Van Nostrum, F. B. G. Bennecker, and H. Brussaard, Inorg. Chem., 1996, 35, 959. CrossRef
12. A. E. Pullen, C. Faulmann, and P. Cassoux, Eur. J. Inorg. Chem., 1999, 38, 269. CrossRef
13. Ö. Salðlam and A. Gül, Polyhedron, 2001, 20, 269. CrossRef
14. B. Ahmet and E. Beytulla, Inorg Chem Commun., 2008 11, 1113. CrossRef
15. J. W. Sibert, S. J. Lange. and C. L. Stern, Angew. Chem., Int. Ed. Engl., 1995, 34, 2020. CrossRef
16. S. J. Lange, J. W. Sibert, and A. G. M. Barret, Tetrahedron, 2000, 56, 7371. CrossRef
17. R. Hendriks, O.E. Sielcken, W. Drenth, and R. J. M. Nolte, J. Chem. Soc., Chem. Commun., 1986, 19, 1464. CrossRef
18. A. R. Koray, V. Ahsen, and O. Bekaroglu, J. Chem. Soc., Chem. Commun., 1986, 13, 932. CrossRef
19. N. Kobayashi and Y. Nishiyama, J. Chem. Soc., Chem. Commun., 1986, 19, 146. CrossRef
20. B. Klaus, N. S. Simonsen, L. Jesper, S. Ole, M. Pernille, J. K. Gitte, and B. Jan, Synthesis, 1996, 407. CrossRef
21. K. Thomas, T. J. Hansen, C. S. Paul, and B. Jan, J. Org. Chem., 1992, 57, 6403. CrossRef
22. J. Sly, P. Kasak, E. Gomar-Nadal, C. Rovira, L. Gorriz, P. Thordarson, D. B. Amabilino, A. E. Rowan, and R. J. M. Nolte, Chem. Commun., 2005, 10, 1255. CrossRef
23. F. Wudl, G. M. Smith, and E. J. Hufnagel, J. Chem. Soc., Chem. Commun., 1970, 21, 1453. CrossRef
24. T. Akutagawa, K. Kakiuchi, T. Hasegawa, T. Nakamura, C.A. Christensen, and J. Becher, Langmuir, 2004, 20, 4187. CrossRef
25. S. Tuncer, A. Koca, A. Gul, and U. Avcıata, Dyes and Pigments, 2009, 81, 144. CrossRef