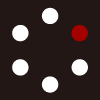
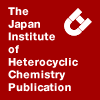
HETEROCYCLES
An International Journal for Reviews and Communications in Heterocyclic ChemistryWeb Edition ISSN: 1881-0942
Published online by The Japan Institute of Heterocyclic Chemistry
e-Journal
Full Text HTML
Received, 9th December, 2009, Accepted, 9th February, 2010, Published online, 10th February, 2010.
DOI: 10.3987/COM-09-11882
■ N-Protected N2-Ethoxymethylenephenylglycine Hydrazides as Precursors of 1,3,4-Oxadiazole Derivatives
Agnieszka Kudelko* and Wojciech Zieliński
Department of Chemical Organic Technology and Petrochemistry, The Silesian University of Technology, Ul. Krzywoustego 4, PL-44100 Gliwice, Poland
Abstract
New derivatives of N2-ethoxymethylene phenylglycine hydrazide protected at the amino group by acetyl and tert-butoxycarbonyl were synthesized with reactions of N-protected phenylglycine hydrazides and triethyl orthoesters. They underwent cyclization to the corresponding N-protected 2-aminomethyl-1,3,4-oxadiazoles in the presence of glacial acetic acid.INTRODUCTION
1,3,4-Oxadiazoles belong to the group of five-membered, non-naturally occurring aromatic heterocycles.1 These compounds were first mentioned in the literature during the late fifties.2,3 They have attracted the attention of many scientists due to a broad spectrum of biological activity. 1,3,4-Oxadiazoles display antibacterial, anticonvulsant, antidepressive, anticancer, and antifungal activities, which makes them potentially useful agents in medicine and agriculture.4-8 Conjugated macrocyclic arrangements based on the 1,3,4-oxadiazole fragment exhibit interesting electron-transfer or luminescent properties and are used in organic light-emitting diodes (OLED), optical brighteners, and laser dyes.9-11
The most popular method to obtain 1,3,4-oxadiazoles is the cyclodehydration of diacylhydrazines in the presence of dehydrating agents such as: polyphosphoric acid,12 phosphorus oxychloride,13 thionyl chloride,14 or boron trifluoride diethyl etherate.15 The starting diacylhydrazines, contain in their structure all the atoms essential for the construction of such a ring, and are usually prepared in reactions of acid hydrazides with synthons, which introduce another carbon atom to the structure. On the other hand, hydrazides could also react with aromatic aldehydes16 or orthoesters17,18 to yield the desired 1,3,4-oxadiazoles. Our earlier studies on the synthesis of 4-acylamino-1,2,4-triazole derivatives in the reaction of α-hydroxyacid hydrazides with triethyl orthoesters showed that the reactions proceed through stable acyclic intermediate, a derivative of N2-ethoxymethylene α-hydroxyacid hydrazide (Scheme 1).19 These compounds contain an imine carbon atom that is susceptible to attack from nucleophiles, which react with another molecule of acid hydrazide to yield the longer chain, the acyclic N,N’-bis(1-hydroxy-1-phenylmethane-carbonylamino)formamidine. This undergoes further cyclization to the desired 1,2,4-triazole in acidic medium at elevated temperature.
Some selected α-hydroxyacid hydrazides and triethyl orthoesters were applied in the one-step synthesis of other heterocyclic arrangements: five-membered 2-hydroxymethyl-1,3,4-oxadiazoles and six-membered 1,3,4-oxadiazin-5(6H)-ones (Scheme 2). The reactions were held in the presence of glacial acetic acid to give a mixture of these two cyclic compounds. The yields of five-membered 1,3,4-oxadiazoles depend on the type of substituent R2 attached to the orthoester. The more bulky the substituent R2 is, the higher the oxadiazole yields. The reversed trend was observed in the case of 1,3,4-oxadiazin-5(6H)-one derivatives. The formation of the latter six-membered compounds resulted from the presence of a highly reactive hydroxy group in hydrazide.20
The reduction of the nucleophilic group situated at the α-position by introducing a protective groupprevents the formation of the six-membered compound and increases the yield of 1,3,4-oxadiazole. Consequently, the derivatives of 2-aminomethyl-1,3,4-oxadiazoles were the only products of the reactions of N-protected phenylglycine hydrazides and triethyl orthoesters (Scheme 2).21
According to our assumed reaction mechanism, the intermediate compound N2-ethoxymethylene hydrazide should play the key role in the building of the heterocyclic products mentioned above. We decided to synthesize such N2-ethoxymethylene acid hydrazides protected at the highly reactive amino group and find proper conditions to obtain the desired 2-aminomethyl-1,3,4-oxadiazoles.
RESULTS AND DISCUSSION
The four enantiomeric phenylglycine hydrazides 2 substituted at the amino group with acetyl (Ac) and tert-butoxycarbonyl group (Boc) were used as starting materials. They were prepared according to a short procedure described in the literature from two enantiomers of phenylglycine and two typical protective agents: acetic anhydride and di-tert-butyl pyrocarbonate.21 The commercially available triethyl orthoesters (R3 = H, Me, Et, Ph), play the dual role of the synthon introducing the methylene carbon atom and the solvent, were applied in the synthesis. The hydrazides and orthoesters were heated to obtain a series of N-protected N2-ethoxymethylene phenylglycine hydrazides 3a-f (Scheme 3).
The best results were obtained when the reactions were conducted in excess orthoester, although in some cases there were problems with separation of products from the post-reaction mixture. The yields of 3a-f fairly vary from 51 to 90% (Table 1) and depend on the kind of substituent R3 from the orthoester molecule. The best result was obtained for the N-Boc-protected N2-ethoxymethylene phenylglycine hydrazide with the imine carbon atom substituted with the phenyl group (3f, entries 11 and12), which seems to be quite reasonable due to the presence of the conjugated system. The rest of the iminoesters with electron-donating substituents resulted in slightly lower yields. The optical rotations of four series of N-protected N2-ethoxymethylene phenylglycine hydrazides 3 obtained from enantiomeric substrates 2 were investigated and showed that they exist as racemic mixtures. These observations were somewhat unexpected because the stereogenic carbon atom situated at the opposite part of the molecule was not involved in the formation of iminoester 3. It is possible that triethyl orthoester, a polar solvent and reagent, could have been responsible for this phenomenon. We decided to decrease the amount of orthoester to the stoichiometric level and conduct the reaction in the presence of xylene, which is a nonpolar solvent with a boiling point similar to orthoester. Unfortunately, even in this case the racemization occurred, proving that an equimolar amount is sufficient for the reaction.
The new compounds were characterised by elemental analysis, IR, NMR spectroscopy, and mass spectrometry. According to 1H-NMR analysis, the N-protected N2-ethoxymethylene phenylglycine hydrazides 3a-f exist in DMSO solution as a mixture of four different forms, due to the quadruple number of peaks for each group of protons (Table 2). Multiplied signals coalesced upon heating the solution to 100 °C. The most characteristic signals in the 1H-NMR spectra come from protons of the ethoxy group that were introduced to molecule 3 by the orthoester, and appears as a triplet (-CH3) at ca. 1.20 ppm and a quartet (-CH2-) from 3.71 to 4.21ppm. The doublets of proton of the parent phenylglycine fragment appear in the broad range from 5.18 to 6.22 ppm. There are also large differences in the chemical shifts of the proton adjacent to the protected amino group. If N2-ethoxymethylene phenylglycine hydrazide is substituted with an acetyl group (3a, 3b), the proton doublet appears at about 8.40-8.63 ppm. For the tert-butoxycarbonyl group (3c-f), it appears in the aromatic range (7.30-7.56 ppm). In the 13C-NMR spectra, the characteristic methylene carbon atom are observed at ca.165 ppm. Two other typical signals of the ethoxy group that was introduced to the N2-ethoxymethylene phenylglycine hydrazide moiety, appear at 15 ppm (-CH3) and 62-67 ppm (-OCH2-). Thus, the formation of the four different structures is the result of both structural and stereo isomerisms.
In our early work on the synthesis of N2-ethoxymethylene benzilic acid hydrazides we found that they exist as a mixture of only two geometric syn and anti isomers relative to the N=C double bond.19 Similar observations were also noted for different heterocyclic hydrazones derived from acidic hydrazides and carbonyl compounds.22,23 However, the presence of the second group of peaks is caused by tautomerism. Phenylglycine derivatives in polar solvents (orthoester, DMSO) exist in two ketone and enol forms, which changes the stereochemistry of the N2-ethoxymethylene hydrazides. They form four different stereoisomers that are in dynamic equilibrium and exhibit nonequivalent spectra (Scheme 4). The optical activity at the stereogenic carbon atom in the two enol structures (enol, syn form, enol, anti form) disappears, and the racemisation is observed.
The new N-Boc protected N2-ethoxymethylene phenylglycine hydrazides (3c-f) were the focus of our study on the cyclization to 1,3,4-oxadiazole derivatives. The initial trials involving the heating of N-Boc protected N2-ethoxymethylene phenylglycine hydrazides (3c-f) in inert solvents such as: benzene, toluene, and xylene were unsuccessful. The cyclization of the compounds proceeded at 50 oC in glacial acetic acid (AcOH) yielding the appropriate 2-(1-N-tert-butoxycarbonylamino-1-phenylmethyl)-1,3,4-oxadiazoles (4a-d). When the mixture of the acyclic N2-ethoxymethylene hydrazide (3c-f) in AcOH was refluxed, the cyclization was accompanied by the exchange of the protection group, which led to the derivatives of 2-(1-N-acetylamino-1-phenylmethyl)-1,3,4-oxadiazole (5a-d).
The yields of two of the series are high, from 75 to 92% (Table 3). Similar to the starting N2-ethoxymethylene phenylglycine hydrazides, the highest values were observed for 1,3,4-oxadiazoles substituted with a phenyl group at position five (4d and 5d).
In contrast, slightly lower yields were obtained from compounds possessing electron-donating groups such as alkyl moieties. They decrease the electrophilicity of the imine carbon atom in 3 and reduce its reactivity in the internal cyclization reaction.
We investigated whether N-Boc substituted 1,3,4-oxadiazoles could be directly transformed into their N-Ac counterparts (Scheme 6). Two 2-(1-N-Boc-amino-1-phenylmethyl)-1,3,4-oxadiazoles possessing methyl (4b) and phenyl (4d) at R3 were refluxed in glacial acetic acid. The progress of the reaction was controlled using TLC analysis. The protecting group was rapidly exchanged to produce the appropriate 2-(1-N-Ac-amino-1-phenylmethyl)-1,3,4-oxadiazoles (5b and 5d) in high yields (82-95%).
It is worth focusing on the structures of two protected 2-(1-amino-1-phenylmethyl)-5-phenyl-1,3,4-oxadiazoles: 4d (-NHBoc) and 5d (-NHAc) (Scheme 7), which can be obtained from the corresponding N2-ethoxymethylene-2-(N-Boc-amino)-2-phenylacethydrazide 3f. Observations show that cyclization makes no measureable changes in the methylene carbon atom shifts. Paraschivescu et al. made similar observations of the synthesis on non-symmetrically 2,5-disubstituted 1,3,4-oxadiazoles containing a benzo[b]thiophene moiety.24 They suggest that such insignificant changes in the chemical shifts of the 1,3,4-oxadiazole carbon atoms in 13C-NMR spectra prove the lack of conjugation between the oxadiazole ring and the aryl moiety. However, that conclusion is questionable if the 1H-NMR spectra of the derivatives 4d and 5d are considered. The two protons H-2” and H-6” of the phenyl group substituted at position five of the 1,3,4-oxadiazole ring are shifted to lower fields, which arise from their proximity to the ring’s nitrogen and oxygen atoms.
Results indicate that both 1,3,4-oxadiazole and the phenyl rings lie untwisted in the same plane and are conjugated. The X-ray analysis of 5d revealed that even in the solid state the rings are nearly coplanar. Such an interaction leads to the exceptional stability in the deprotection of N-protected 2-(1-aminomethyl)-1,3,4-oxadiazoles proceeding in acidic media.21
CONCLUSIONS
New derivatives of N2-ethoxymethylene phenylglycine hydrazide, obtained in the reactions of N-protected phenylglycine hydrazides and triethyl orthoesters, are valuable reagents for the preparation of non-symmetrically substituted N-protected 2-aminomethyl-1,3,4-oxadiazoles. The cyclization of the N2-ethoxymethylene phenylglycine hydrazides carried out in glacial acetic acid appears to be an efficient, rapid, and easy way to obtain five-membered 1,3,4-oxadiazoles. The presented protocol may be especially useful in the synthesis of macrocyclic systems based on the easy-to-bind 2-aminomethyl-1,3,4-oxadiazole moiety.
EXPERIMENTAL
Melting points were measured using an APA II melting point apparatus and are uncorrected. UV spectra were recorded on a Shimadzu UV-2102 spectrophotometer. Elemental analyses were performed with a VarioEL analyser in PAN Zabrze. The 1H- and 13C-NMR spectra were recorded on a Varian Inova 300 spectrometer in DMSO solution using TMS as the internal standard. Thin-layer chromatography was performed on silica gel 60 F254 (Merck) thin-layer chromatography plates using benzene-AcOEt (1:5 v/v) as the mobile phase. Optical rotations were measured on a Perkin Elmer Polarimeter 141 in CHCl3 solutions at approximately 1% concentrations (D line of sodium light, room temperature). IR spectra were recorded between 3800-600 cm-1 on a Zeiss Specord 80 spectrometer in MeCN solutions. Mass spectra were obtained on a Waters HPLC/MS system using the EI technique (70 eV).
Synthesis of α-N-protected phenylglycine hydrazides (2). The appropriate α-N-protected phenylglycine hydrazides were obtained according to a procedure described in the literature from the two enantiomers of phenylglycine.
L-(+)-N-Ac-phenylglycine hydrazide (2a).21
Yield: 85%, mp 210-212 °C, [α]D20 +95.2 (MeOH, C = 1%), IR (MeCN) ν/cm-1: 3645, 3540, 3020, 1690, 1640, 1230, 770, 665.
D-(-)-N-Ac-phenylglycine hydrazide (2b).21
Yield: 90%, mp 196-198 °C, [α]D20 –93.6 (MeOH, C = 1%).
L-(+)-N-Boc-phenylglycine hydrazide (2c).21
Yield: 66%, mp 105-107 °C, [α]D20 +105.3 (CHCl3, C = 1%), IR (MeCN) ν/cm-1: 3650, 3540, 3360, 2980, 2940, 1720, 1690, 1630, 1250, 1170, 710.
D-(-)-N-Boc-phenylglycine hydrazide (2d).21
Yield: 73%, mp 118-121 °C, [α]D20 -101.7 (CHCl3, C = 1%).
General procedure for the preparation of N-protected N2-ethoxymethylene phenylglycine hydrazides (3a-f). The starting α-N-protected phenylglycine hydrazide 2 (0.01 mol) was added to a mixture of the appropriate triethyl orthoester (0.05 mol) and kept under reflux for about 3 h (TLC). After cooling, the excessive orthoester was evaporated under reduced pressure. The crude oils were triturated with Et2O and then purified by crystallization from benzene-hexane mixtures.
N2-Ethoxymethylene-2-(N-Ac-amino)-2-phenylacethydrazide (3a, Entries 1 and 2).
This compound was obtained as a white solid in 63% yield, mp 124-125 °C, Rf 0.10 (benzene-AcOEt, 1:5 v/v). (Anal. Calcd for C13H17N3O3: C, 59.29; H, 6.52; N, 15.95. Found: C, 59.41; H, 6.59; N, 16.03%). UV λmax (MeOH): 202 nm (ε·10-3 13.01 cm-1M-1), 227 (9.15). 13C-NMR (75 MHz, DMSO-d6): δ 14.78, 23.04, 56.16, 63.11, 127.85, 128.02, 128.95, 139.35, 155.68, 166.39, 169.73. MS (EI, 70 eV) m/z (%): 217 (10), 175 (15), 149 (24), 132 (11), 106 (100), 105 (36), 104 (52), 91 (18), 79 (21), 77(42), 71 (19), 60 (25), 57 (18). IR (MeCN) ν/cm-1: 3420, 3380, 3015, 1665, 1520, 1240, 1080, 710.
N2-Ethoxypropylene-2-(N-Ac-amino)-2-phenylacethydrazide (3b, Entries 3 and 4).
This compound was obtained as a white solid in 72% yield, mp 177-178 °C, Rf 0.15 (benzene-AcOEt, 1:5 v/v). (Anal. Calcd for C15H21N3O3: C, 68.42; H, 7.28; N, 14.41. Found: C, 68.59; H, 7.32; N, 14.50%). UV λmax (MeOH): 203 nm (ε·10-3 15.11 cm-1M-1), 228 (8.23). 13C-NMR (75 MHz, DMSO-d6): δ 9.55, 14.07, 21.67, 22.40, 55.05, 61.81, 127.06, 127.44, 128.21, 139.00, 165.49, 168.52, 169.01. MS (EI, 70 eV) m/z (%): 245 ( 12), 202 (23), 175 (10), 149 (28), 143 (19), 132 (16), 115 (12), 106 (100), 105 (24), 104 (71), 94 (48), 91 (32), 89 (12), 79 (26), 77 (45), 57 (41). IR (MeCN) ν/cm-1: 3420, 3380, 3010, 1660, 1510, 1240, 1080, 700.
N2-Ethoxymethylene-2-(N-Boc-amino)-2-phenylacethydrazide (3c, Entries 5 and 6).
This compound was obtained as a white solid in 53% yield, mp 143-144 °C, Rf 0.50 (benzene-AcOEt, 1:5 v/v). (Anal. Calcd for C16H23N3O4: C, 59.80; H, 7.10; N, 13.13. Found: C, 59.71; H, 7.15; N, 13.05%). UV λmax (MeOH): 202 nm (ε·10-3 13.44 cm-1M-1), 233 (11.64). 13C-NMR (75 MHz, DMSO-d6): δ 14.10, 28.16, 58.86, 62.48, 78.42, 127.22, 128.21, 128.29, 138.45, 154.97, 161.08, 171.14. MS (EI, 70 eV) m/z (%): 206 (21), 151 (12), 132 (10), 115 (15), 106 (100), 104 (18), 87 (11), 79 (14), 77 (13), 59 (15), 57 (82). IR (MeCN) ν/cm-1: 3645, 3548, 3362, 2995, 2954, 1710, 1634, 1317, 1243, 1168, 1084, 895.
N2-Ethoxyethylene-2-(N-Boc-amino)-2-phenylacethydrazide (3d, Entries 7 and 8).
This compound was obtained as a white solid in 65% yield, mp 119-121 °C, Rf 0.52 (benzene-AcOEt, 1:5 v/v). (Anal. Calcd for C17H25N3O4: C, 61.12; H, 7.41; N, 12.53. Found: C, 61.21; H, 7.39; N, 12.56%). UV λmax (MeOH): 202 nm (ε·10-3 14.51cm-1M-1), 230 (9.43). 13C-NMR (75 MHz, DMSO-d6): δ 14.61, 15.78, 28.68, 57.11, 62.42, 78.90, 127.16, 128.06, 128.33, 139.19, 155.01, 165.55, 171.00. MS (EI, 70 eV) m/z (%): 206 (10), 151 (12), 150 (63), 132 (11), 129 (53), 106 (100), 101 (27), 87 (10), 79 (13), 77 (12), 59 (18), 57 (92). IR (MeCN) ν/cm-1: 3638, 3544, 3341, 2990, 2947, 1712, 1650, 1307, 1250, 1175, 1074, 900, 710.
N2-Ethoxypropylene-2-(N-Boc-amino)-2-phenylacethydrazide (3e, Entries 9 and 10).
This compound was obtained as a white solid in 76% yield, mp 120-121 °C, Rf 0.55 (benzene-AcOEt, 1:5 v/v). (Calcd. Anal. for C18H27N3O4: C, 62.01; H, 7.72; N, 12.12. Found: C, 62.30; H, 7.77; N, 12.07%). UV λmax (MeOH): 202 nm (ε·10-3 15.26 cm-1M-1), 231 (10.07). 13C-NMR (75 MHz, DMSO-d6): δ 9.52, 14.07, 21.65, 28.17, 56.64, 61.67, 78.41, 127.10, 127.78, 128.23, 138.91, 155.03, 165.68, 168.75. MS (EI, 70 eV) m/z (%): 206 (10), 151 (13), 150 (65), 143 (62), 132 (11), 115 (28), 106 (100), 104 (17), 87 (10), 79 (15), 77 (12), 59 (15), 57 (95). IR (MeCN) ν/cm-1: 3644, 3546, 3381, 2992, 2945, 1708, 1640, 1252, 1175, 1073, 900, 761, 705.
N2-Ethoxybenzylidene-2-(N-Boc-amino)-2-phenylacethydrazide (3f, Entries 11 and 12).
This compound was obtained as a white solid in 89% yield, mp 134-135 °C, Rf 0.56 (benzene-AcOEt, 1:5 v/v). (Anal. Calcd for C22H27N3O4: C, 66.50; H, 6.80; N, 10.58. Found: C, 66.69; H, 6.84; N, 10.69%). UV λmax (MeOH): 201 nm (ε·10-3 18.91cm-1M-1), 269 (11.60). 13C-NMR (75 MHz, DMSO-d6): δ 15.01, 28.14, 56.48, 62.82, 78.47, 127.17, 127.37, 127.85, 128.15, 128.33, 128.64, 128.95, 138.57, 138.83, 155.10, 166.69, 171.25. MS (EI, 70 eV) m/z (%): 206 (12), 191 (40), 177 (21), 163 (15), 150 (51), 132 (10), 106 (100), 105 (78), 104 (51), 103 (22), 87 (11), 79 (23), 77 (62), 59 (15), 57 (79). IR (MeCN) ν/cm-1: 3640, 3558, 3374, 3031, 2986, 1702, 1634, 1322, 1245, 1173, 1081, 1022, 895, 763, 724.
Cyclization of N-Boc protected N2-ethoxymethylene phenylglycine hydrazides to N-Boc protected 2-(1-aminomethyl)-1,3,4-oxadiazoles (4a-d). The appropriate N-protected N2-ethoxymethylene phenylglycine hydrazide (3c-f) (5 mmol) was dissolved in 10 mL of glacial AcOH. The mixture was kept on a water bath at 50 oC for about 4 hours (TLC). Then the solution was concentrated on a rotary evaporator. The crude products (4a-d) were subjected to the column chromatography (silica gel, eluent: benzene-AcOEt, 1:5 mixture) or were crystallized from benzene-hexane mixtures.
2-(1-N-Boc-amino-1-phenylmethyl)-1,3,4-oxadiazole (4a).21
This compound was obtained as a white solid in 75% yield, mp 113-115 °C. 1H NMR (300 MHz, DMSO-d6): δ 1.40 (9H, s, (CH3)3), 6.14 (1H, d, J = 8.1 Hz, CH), 7.32-7.45 (5H, m, Ph), 8.31 (1H, d, J = 8.1 Hz, NH), 9.20 (1H, s, H-C5). 13C-NMR (75 MHz, DMSO-d6): δ 28.09, 50.44, 78.96, 127.56, 128.22, 128.55, 137.00, 154.77 (CO-O-t-Bu), 156.08 (C5), 165.74 (C2).
5-Methyl-2-(1-N-Boc-amino-1-phenylmethyl)-1,3,4-oxadiazole (4b).21
This compound was obtained as a white solid in 81% yield, mp 98-100 °C. 1H NMR (300 MHz, DMSO-d6): δ 1.38 (9H, s, (CH3)3), 2.44 (3H, s, CH3-C5), 6.03 (1H, d, J = 8.1 Hz, CH), 7.32 -7.43 (5H, m, Ph), 8.25 (1H, d, J = 8,1 Hz, NH). 13C-NMR (75 MHz, DMSO-d6): δ 10.55, 28.19, 50.58, 79.00, 127.62, 128.25, 128.63, 137.21, 155.01 (CO-O-t-Bu), 164.25 (C5), 165.93 (C2).
5-Ethyl-2-(1-N-Boc-amino-1-phenylmethyl)-1,3,4-oxadiazole (4c).21
This compound was obtained as a white solid in 85% yield, mp 123-125 °C, 1H NMR (300 MHz, DMSO-d6): δ 1.21 (3H, t, J = 8.1 Hz, CH3-C5), 1.38 (9H, s, (CH3)3), 2.81 (2H, q, J = 8.1 Hz, CH2-C5), 6.03 (1H, d, J = 8.1 Hz, CH), 7.31-7.41 (5H, m, Ph), 8.24 (1H, d, J = 8,1 Hz, NH). 13C-NMR (75 MHz, DMSO-d6): δ 10.45, 18.35, 28.19, 50.63, 78.99, 127.62, 128.24, 128.63, 137.22, 155.03 (CO-O-t-Bu), 165.82 (C2), 168.10 (C5).
2-(1-N-Boc-amino-1-phenylmethyl)-5-phenyl-1,3,4-oxadiazole (4d).21
This compound was obtained as a white solid in 92% yield, mp 131-133 °C, 1H NMR (300 MHz, DMSO-d6): δ 1.38 (9H, s, (CH3)3), 6.19 (1H, d, J = 8.1 Hz, CH), 7.33-7.42 (3H, m, C2 - H-3’, H-4’, H-5’), 7.49 (2H, d, J = 6.9 Hz, C2 - H-2’, H-6’), 7.55-7.62 (3H, m, C5 - H-3”, H-4”, H-5”), 7.95 (2H, d, J = 7.8 Hz, C5 - H-2”, H-6”), 8.38 (1H, s, J = 8.1 Hz, NH). 13C-NMR (75 MHz, DMSO-d6): δ 28.10, 50.63, 79.03, 123.14, 126.48, 127.55, 128.25, 128.62, 129.45, 132.10, 137.06, 155.00 (CO-O-t-Bu), 164.33 (C5), 165.99 (C2). IR (MeCN) ν/cm-1: 3644, 3516, 3362, 2980, 1723, 1648, 1560, 1241, 1165, 1024, 900, 782, 724.
Transformation of N-Boc protected N2-ethoxymethylene phenylglycine hydrazides to N-Ac protected 2-(1-aminomethyl)-1,3,4-oxadiazoles (5a-d). The appropriate N-protected N2-ethoxymethylene phenylglycine hydrazide (3c-f) (5 mmol) was dissolved in 10 mL of glacial AcOH. The mixture was kept under reflux for about 1-2 h (TLC). Then the solution was concentrated on a rotary evaporator. The crude products (5a-d) were crystallized from EtOH.
2-(1-N-Ac-amino-1-phenylmethyl)-1,3,4-oxadiazole (5a).21
This compound was obtained as a white solid in 94% yield, mp 120-121 °C. 1H NMR (300 MHz, DMSO-d6): δ 1.95 (3H, s, CH3), 6.40 (1H, d, J = 7.8 Hz, CH), 7.36-7.42 (5H, m, Ph), 9.18 (1H, d, J = 7.8 Hz, NH), 9.23 (1H, s, H-C5). 13C-NMR (75 MHz, DMSO-d6): δ 22.29, 48.75, 127.59, 128.41, 128.79, 136.76, 154.85 (C5), 165.60 (C2), 169.21 (COCH3).
5-Methyl-2-(1-N-Ac-amino-1-phenylmethyl)-1,3,4-oxadiazole (5b).21
This compound was obtained as a white solid in 95% yield, mp 112-113 °C. 1H NMR (300 MHz, DMSO-d6): δ 1.90 (3H, s, CH3), 2.47 (3H, s, CH3-C5), 6.30 (1H, d, J = 8.1 Hz, CH), 7.33-7.40 (5H, m, Ph), 9.19 (1H, d, J = 8.1 Hz, NH). 13C-NMR (75 MHz, DMSO-d6): δ 10.54, 22.32, 48.72, 127.57, 128.33, 128.75, 136.92, 164.23 (C5), 165.71 (C2), 169.16 (COCH3).
5-Ethyl-2-(1-N-Ac-amino-1-phenylmethyl)-1,3,4-oxadiazole (5c).21
This compound was obtained as a white solid in 95% yield, mp 89-91 °C. 1H NMR (300 MHz, DMSO-d6): δ 1.22 (3H, t, J = 7.5 Hz, CH3-C5), 1.94 (3H, s, CH3), 2.83 (2H, q, J = 7.5 Hz, CH2-C5), 6.33 (1H, d, J = 8.1 Hz, CH), 7.37-7.41 (5H, m, Ph), 9.15 (1H, d, J = 8.1 Hz, NH). 13C-NMR (75 MHz, DMSO-d6): δ 10.34, 18.29, 22.29, 48.73, 127.52, 128.31, 128.74, 136.93, 165.59 (C2), 168.03 (C5), 169.09 (COCH3).
2-(1-N-Ac-amino-1-phenylmethyl)-5-phenyl-1,3,4-oxadiazole (5d).21
This compound was obtained as a white solid in 98% yield, mp 167-168 °C, 1H NMR (300 MHz, DMSO-d6): δ 1.97 (3H, s, CH3), 6.44 (1H, d, J= 8.1 Hz, CH), 7.34-7.41 (3H, m, C2 – H-3’, H-4’, H-5’), 7.48 (2H, d, J = 7.5 Hz, C2 - H-2’, H-6’), 7.56-7.60 (3H, m, C5 – H-3”, H-4”, H-5”), 7.95 (2H, d, J = 7.8 Hz, C5 - H-2”, H-6”) , 9.29 (1H, d, J = 8.1 Hz, NH). 13C-NMR (75 MHz, DMSO-d6): δ 22.34, 48.96, 123.13, 126.57, 127.64, 128.39, 128.79, 129.47, 132.14, 136.81, 164.37 (C5), 165.93 (C2), 169.31 (COCH3). IR (MeCN) ν/cm-1: 3645, 3542, 3361, 3020, 1693, 1632, 1244, 1087, 966, 908.
Transformation of N-Boc protected 2-(1-aminomethyl)-1,3,4-oxadiazoles (4b, 4d) into their N-Ac protected counterparts (5b, 5d). The appropriate 2-(1-N-Boc-amino-1-phenylmethyl)-1,3,4-oxa- diazole (4b, 4d) (3.5 mmol) was dissolved in 10 mL of glacial AcOH. The mixture was kept under reflux for about 1-2 h (TLC). Then the solution was concentrated on a rotary evaporator. The crude products (5b, 5d) were subjected to the column chromatography (silica gel, eluent: MeOH-CHCl3, 1:1 mixture) giving the pure N-Ac protected 1,3,4-oxadiazoles: 5b (yield: 82%, mp 112-113° C), 5d (yield: 95%, mp 166-168 °C).
References
1. A. R. Katritzky, C. A. Ramsden, E. F. V. Scriven, and R. J. K. Taylor, Comprehensive Heterocyclic Chemistry III, Elsevier Science Ltd., Oxford, 2008, vol. 5, 398.
2. C. Ainsworth, J. Am. Chem. Soc., 1955, 77, 1148. CrossRef
3. E. Muller and D. Ludsteck, Chem. Ber., 1955, 88, 921. CrossRef
4. M. Amir and K. Shikha, Eur. J. Med. Chem., 2004, 39, 535. CrossRef
5. A. Almasirad, S. A. Tabatabai, M. Faizi, A. Kebriaeezadeh, N. Mehrabi, A. Dalvandi, and A. Shafiee, Bioorg. Med. Chem. Lett., 2004, 14, 6057. CrossRef
6. H. A. Rajapakse, H. Zhu, M. B. Young, and B. T. Mott, Tetrahedron Lett., 2006, 47, 4827. CrossRef
7. X. Zheng, Z. Li, Y. Wang, W. Chen, Q. Huang, C. Liu, and G. Song, J. Fluorine Chem., 2003, 123, 163. CrossRef
8. X.-J. Zou, L.-H. Lai, G.-Y. Jin, and Z.-X. Zhang, J. Agric. Food Chem., 2002, 50, 3757. CrossRef
9. B. Schulz, I. Orgzall, A. Freydank, and C. Xu, Advances in Colloid and Interface Science, 2005, 116, 143. CrossRef
10. Z. K. Chen, H. Meng, Y. H. Lai, and W. Huang, Macromolecules, 1999, 32, 4351. CrossRef
11. N. Tamoto, C. Adachi, and K. Nagai, Chem. Mater., 1997, 9, 1077. CrossRef
12. C. K. Reddy, P. S. N. Reddy, and C. V. Ratnam, Synthesis, 1983, 842. CrossRef
13. S. Cao, X. Qian, G. Song, and Q. Huang, J. Fluorine Chem., 2002, 117, 63. CrossRef
14. L. El Kain, I. Le Menestrel, and R. Morgentin, Tetrahedron Lett., 1998, 39, 6885. CrossRef
15. V. K. Tandon and R. B. Chhor, Synth. Commun., 2001, 31, 1727. CrossRef
16. M. Dabiri, P. Salehi, M. Baghbanzadeh, and M. Bahramnejad, Tetrahedron Lett., 2006, 47, 6983. CrossRef
17. C. Ainsworth, J. Am. Chem. Soc., 1965, 87, 5800. CrossRef
18. W. R. Tully, C. R. Cardner, R. J. Gillespie, and R. Westwood, J. Med. Chem., 1991, 34, 2060. CrossRef
19. W. Zieliński, A. Kudelko, and W. Czardybon, J. Heterocycl. Chem., 2005, 42, 1393. CrossRef
20. A. Kudelko and W. Zieliński, Heterocycles, 2006, 68, 2269. CrossRef
21. A. Kudelko and W. Zieliński, Tetrahedron, 2009, 65, 1200. CrossRef
22. A. Rutavichyus and S. Valyulene, Khim. Geterotsikl. Soedin.,1998, 12, 1690 (Chem. Heterocycl. Comp., 1998, 34(12), 1436). CrossRef
23. A. Rutavichyus and S.Valyulene, Khim. Geterotsikl. Soedin., 2000, 7, 966 (Chem. Heterocycl. Comp., 2000, 36(7), 851). CrossRef
24. C. C. Paraschivescu, F. Dumitrascu, C. Draghici, L. L. Ruta, M. Matache, I. Baciu, and C. Dobrota, Arkivoc, 2008, xiii, 198.