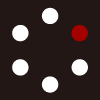
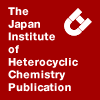
HETEROCYCLES
An International Journal for Reviews and Communications in Heterocyclic ChemistryWeb Edition ISSN: 1881-0942
Published online by The Japan Institute of Heterocyclic Chemistry
e-Journal
Full Text HTML
Received, 13th December, 2009, Accepted, 29th January, 2010, Published online, 1st February, 2010.
DOI: 10.3987/COM-09-11883
■ Absolute Configuration of Sargaol Acetate Using DFT Calculations and Vibrational Circular Dichroism
Marcelo A. Muñoz, Carlos Areche, Juana Rovirosa, Aurelio San-Martín, and Pedro Joseph-Nathan*
Departamento de Química, Centro de Investigación y de Estudios Avanzados del Instituto Politécnico Nacional, A.P. 14-740, Avenida IPN 2508, Esquina Ticoman, C.P. 07000 México, D.F., Mexico
Abstract
The absolute configuration (AC) of sargaol acetate (2), isolated from the brown alga Stypopodium flabelliforme, was determined using vibrational circular dichroism (VCD) measurements and density functional theory (DFT) calculations. Conformational searches using the Monte Carlo stochastic algorithm for 2 and three model compounds with a smaller side chain (diisoprenyl, isoprenyl and ethyl) provided 2172, 596, 82 and 12 conformations, respectively. In the last two cases the moderate number of conformations allowed conformational distributions assessment using single point energy calculations and considering a Boltzmann behavior. In the larger model molecules a prior selection from the original conformational set was done based on structure diversity as implemented in the Spartan`04 modeling software, keeping only 100 conformations in each case. All final conformers where further subjected to geometry optimizations and vibrational calculations providing weighted theoretical IR and VCD spectra which were compared to the experimental spectrum. In all cases only very weak bands are observed above 1300 cm-1, the strong bands around 1200 cm-1 clearly show that the AC of 2 is R, and only models including isoprenyl units allowed good prediction of bands in the 1000 - 1100 cm-1 spectral region.INTRODUCTION
Vibrational circular dichroism (VCD) is developing as a reliable methodology to obtain the absolute configuration (AC) of organic molecules1 which has been applied successfully in the natural products field.2 It allows the AC determination of a single enantiomer and is quite superior than electronic circular dichroism (ECD) since VCD calculations are performed in the ground state,1,2 a situation not holding for ECD calculations. Nevertheless the practical limits imposed by ab initio calculations in terms of molecular size and flexibility regarding costly computer time have not been fully explored.
Chromenes, a class of meroterpenoids having a polyprenyl chain bonded to a hydroquinone or related aromatic systems, have been isolated from many marine3,4 and terrestial5-8 species collected from different parts of the world. In most cases the AC of the obtained compounds has been studied using electronic circular dichroism (ECD), but also anomalous scattering X-ray crystallography of chemically related compounds has been used for conformationally rigid systems, and the absolute configuration of the ring carbon bearing the polyprenyl chain has been assigned either as R or S. In continuation of our studies on the AC determination of meroditerpenoids that include stypotriol triacetate9 and isoepitaondiol diacetate,10
we now turned our attention to sargaol acetate (2).
Sargaol (1) is a chromene substituted with a large unsaturated carbon atoms chain that has shown anti-oxidant activity.11 It was isolated from the brown algae Sargassum tortile12 and Taonia atomaria,13 and also from the steam bark of Dimocarpus fumatus.14 While samples from T. atomaria and S. tortile gave no optical activity, that isolated from D. fumatus showed a dextrorotatory optical rotation ([α]D +2.54º, CHCl3, c 0.55). Nevertheless the natural origin of these samples has been questioned since they may be artifacts derived from the corresponding geranyl-genarylbenzoquinone.12,14 Additionally in the case of D. fumatus the optical activity was considered to be caused by impurities based on an ECD experiment.14
A recent careful isolation15 of sargaol acetate (2) from the brown alga Stypopodium flabelliforme provided a dextrorotatory molecule ([α]D +1.77º, CHCl3, c 0.60) whose VCD study is described herein.
RESULTS AND DISCUSSION
As has been established in the literature, to obtain the absolute configuration of a molecule using VCD it is necessary to consider all significantly populated conformations under the experimental measurement conditions. Nevertheless in the case of sargaol acetate (2) the tris-isoprenyl side chain confers an extremely high conformational flexibility to the molecule which makes it prohibitive to ascertain and consider all conformations. To overcome this limitation it is possible to use a suitable molecular fragment that models the entire molecule by replacing large achiral substituents with
significantly smaller ones thereby drastically reducing the number of low energy conformations that need to be considered,1 as was done for gossypol in a pioneering study.16 As a first approach for a sargaol acetate model we chose an ethyl group to replace the tris-isoprenyl side chain (model compound 3) which yielded 12 conformations in the first 10 kcal/mol of a Monte Carlo conformational search. These conformations were subjected to geometry optimization procedures at the DFT//B3LYP/DGDZVP level of theory to obtain their free energies, showing that 6 conformers account for 99.97% of the entire conformational distribution which are shown superimposed in Figure 1. The vibrational calculations at the same level of theory gave the theoretical VCD spectrum which is compared to the experimental spectrum in Figure 2. It is observed that the sign of the intense bands around 1200 cm-1 in the experimental VCD spectrum are well predicted, while those in the 1000 - 1100 cm-1 range are not well predicted by these calculations, and only very weak bands are observed above 1300 cm-1. Although the sign of the more intense bands clearly suggest calculation of the correct R enantiomer, failure in the relative intensity of these bands along with other bands in the experimental VCD spectrum suggests that further calculations should be performed.
As a second approach, the side chain of 2 was replaced by a single isoprenyl group, as depicted in model compound 4 which increases the conformational flexibility of the model molecule but still allows calculation of all significantly populated conformations. In this case 82 conformations where obtained from the Monte Carlo conformational search which were reduced to 15 after DFT//B3LYP/6-31G(d) single point energy calculations.
These conformations were subjected to geometry reoptimizations at the DFT//B3LYP/DGDZVP level of theory followed by vibrational calculations, allowing comparison of the experimental and weighted VCD spectra as shown in Figure 4. As observed, the same signs and relative intensities of the two bands around 1200 cm-1 obtained for 3 were also obtained using 4 as the model compound. In addition, bands in the 1000-1100 cm-1 spectral range are more accurately predicted than in the previous case, probably due to greater structural similarity of the current model.
While again the sign of the main bands observed in the experimental VCD spectrum are well reproduced by the vibrational calculations, also suggesting the R configuration of the sole asymmetric carbon atom, we decided to use a further different approach to confirm the stereostructure assignment.
Consequently, the next logical step is the use of model compound 5 to get closer to real structure 2. Nevertheless the extension of the side chain with an additional isoprenyl unit dramatically increases the conformational flexibility of the model, making his conformational distribution extremely costly, in terms of computational resources, to determine the complete conformational picture with the same level of confidence reached for the previously discussed two models. Therefore a different approach to obtain a conformational distribution, namely selecting the more diverse set of conformations representing the entire distribution, as implemented in the Spartan 04 software, can be used. This is done by selecting conformations based on structure diversity and keeping only those who are more different from each other. In this approach only 100 conformations are selected and kept for further mathematical treatment as is done for the original distribution in the two previous models.
In the case of model compound 5 the Monte Carlo conformational search found 596 conformations from which 100 where selected as described above. From this set, single point energy calculations at the B3LYP/6-31G(d) level of theory showed that 34 more abundant conformations account for 90.5% of the distribution which are shown superimposed in Figure 5. The 34 selected conformations where further subjected to optimization at the DFT//B3LYP/DGDZVP level of theory and then to vibrational calculations at the same level from which a VCD spectrum was obtained and compared to that of 2 in Figure 6.
In this case a better correlation between the theoretical VCD spectrum of model compound 5 and the experimental VCD spectrum of 2 is obtained, particularly in the low energy portion of the trace. Also the same sign and intensity is observed for the intense bands confirming the same stereostructure assignment made using the simpler models.
Finally, and as the last approach to confirm the absolute configuration of 2 suggested by all used models, a Monte Carlo conformational search for the complete structure was conducted. From this search, 2172 conformations where found from which 100 where keep based on their structure diversity as already described for the biggest used model compound. From this set of conformations single point energy calculation showed that only 14 conformations, shown superimposed in Figure 7, account for 95.8% of the corresponding distribution, and thus they were selected to complete the whole calculation procedure as done in the previous cases to obtain a theoretical VCD spectrum of 2, which in turn could be compared to the corresponding experimental trace in Figure 8.
This approach provided a very similar result than those obtained for model compounds 4 and 5 clearly confirming the absolute configuration of sargaol acetate (2) as R. Nevertheless the relative intensity of the intense bands positioned around 1200 cm-1 is not accurately predicted as in all other calculated model molecules. This seems to own to the restricted conformational space considered in these modeled calculations compared to the very high conformational flexibility of the real molecule in solution, in which much more bands of different intensity or even opposite sign are weighted and originate the shape of the experimental VCD spectrum. In any event the VCD approach for the study of chromenes seems to be quite superior than ECD studies of these molecules since significantly different spectra are observed when comparing published cases.8
EXPERIMENTAL
Optical rotations were measured on a Perkin Elmer 341 polarimeter, NMR spectra were recorded on a Varian Mercury 300 spectrometer operating at 300 MHz for 1H and 75 MHz for 13C using 99.8% atom-D CDCl3 and TMS as the internal standard, IR and VCD spectra were measured on a BioTools ChiralIR FT spectrophotometer equipped with dual photoelastic modulation using 5.9 mg of 2 in 150 µL of CCl4 placed in a BaF2 cell with 100 µm pathlength acquiring data at a resolution of 4 cm-1 during 5 h, the UV spectrum was recorded on a Perkin Elmer Lambda 12 spectrophotometer, the low resolution mass spectrum was measured on a Varian Saturn 2000 spectrometer and the high resolution mass spectrum was measured at the UCR mass spectrometer facility, University of California, Riverside, CA.
Algal Collection. The brown alga Stypopodium flabelliforme was collected intertidally near Hanga Roa, Rapa Nui, Easter Island (South Pacific Ocean), Chile, at 109°25’36.4”W and 27°06’37.3”S in March 2007 at 5-10 m depth by SCUBA diving. A voucher specimen number 2207 is deposited at Museo Nacional de Historia Natural, Santiago, Chile where its identity was confirmed by Prof. M. Eliana Ramirez.
Extraction and Isolation. Fresh algal material (2 kg) was frozen for air transportation (3757 km) to the continent and then extracted with CH2Cl2/MeOH 1:1 (3 x 6 L) at room temperature for 7 days. After filtration, the solvent mixture was concentrated under reduced pressure below 40 °C yielding 50.5 g of a brown residue which was immediately acetylated using Ac2O/Py. After work-up and evaporation, 45 g of residue were obtained which were subjected to flash chromatography on silica gel as recently described15 using n-hexane/EtOAc to produce four fractions. The first fraction, eluted with n-hexane/EtOAc 9/1 gave 15 g which were rechromatographed over silica gel, 200-500 μm eluting with EtOAc-n-hexane (1:9, v/v) to give three fractions. The second of these fractions (2 g) was further purified by CC (silica gel, 63-200 μm) using n-hexane/EtOAc (49:1) to yield 100 mg of sargaol acetate (2) followed by 2-(geranylgeranyl)-6-methyl-1,4-benzohydroquinone diacetate (40 mg), 2-[2′(E)-3′,7′,11′,15′-tetramethyl hexadec-2-en-1′-yl]-6-methyl-1,4-benzohydroquinone diacetate (4 mg) and epitaondiol diacetate (1 g).
Sargaol acetate (2).
Oil; [α]589 +1.77, [α]578 +1.45, [α]546 +0.97, [α]436 -0.64 (c, 0.62 CHCl3); 1H NMR (CDCl3, 300 MHz) δ 6.68 (1H, d, J = 2.5, H-7), 6.55 (1H, d, J = 2.5, H-5), 6.27 (1H, d, J = 9.9, H-4), 5.56 (1H, d, J = 9.9, H-3), 5.09 (3H, m, H-3’, H-7’and H-11’), 2.25 (3H, s, Ac), 2.16 (3H, s, H-18’), 2.12 (2H, m, H-2’), 2.06 (2H, m, H-6’), 2.05 (2H, m, H-10’), 1.97 (4H, m, 2H-5’ and 2H-9’), 1.69 (2H, m, H-1’), 1.68 (3H, brs, H-13’), 1.60 (3H, brs, H-14’), 1.59 (6H, brs, 3H-15’ and 3H-16’), 1.38 (3H, s, H-17’); 13C NMR (CDCl3, 75 MHz) δ 170.0 (CO), 148.6 (C-6), 143.2 (C-8a), 135.3 (C-8’), 134.9 (C-4’), 131.2 (C-12’), 130.2 (C-3), 126.2 (C-8), 124.3 (C-11’), 124.1 (C-7’), 123.9 (C-3’), 122.8 (C-7), 122.5 (C-4), 120.8 (C-4a), 116.4 (C-5), 78.4 (C-2), 41.1. (C-1’), 39.6 (C-5’), 39.6 (C-9’), 26.7 (C-10’), 26.5 (C-6’), 26.3 (C-17’), 25.6 (C-13’), 22.5 (C-2’), 21.0 (Ac), 17.6 (C-14’), 15.9 (C-15’), 15.8 (C-16’), 15.5 (C-18’; UV (EtOH) λmax (ε) 317 (2400), 265 (4400), 227 (26300) nm; IR (CCl4) νmax 1763, 1469, 1368, 1208 cm-1; EM m/z 394 (5.4), 218 (14.4), 217 (100), 175 (21.5); HRESIMS m/z 437.3050 (calc for C29H40O3 + H), 437.3056.
Computational details: Conformational searches were performed using the Monte Carlo stochastic algorithm within the molecular mechanics force field (MMFF94) and considering an energy window of 10 kcal/mol. Automatic conformational selection based on structure diversity was used in conformational distributions having a high amount of conformers. Single point energy calculation were performed using the DFT B3LYP/6-31G(d) level of theory, while geometry optimizations and vibrational calculations were performed using the DFT B3LYP/DGDZVP level of theory from which frequencies, dipole and rotational strengths were obtained. The later data were used to obtain plots using Lorentzian bands with half-widths of 6 cm-1 and considering an anharmonicity factor of 0.97. Final weighted VCD plots were obtained considering a Boltzmann distribution and using free energies derived from the vibrational calculations. Molecular mechanics and single point energy calculations were performed using the Spartan`04 software package,17 while geometry optimizations and vibrational calculations were performed using the Gaussian 03W software package.18 Computation of each conformation of 2-5, including geometry optimization and vibrational calculations, take in average 88.9, 11.0, 33.7, and 68.5 h of CPU time, respectively, when using a personal computer running at 3.0 GHz suited with 2 Gb of RAM. Thus the cost of computer time for this study exceeded 4250 h.
ACKNOWLEDGEMENTS
Financial support came from Proyecto Anillo “ACT-38”, PBCT “PDA-13” and CONACYT-Mexico grant No. 61122.
References
1. T. B. Freedman, X. Cao, R. K. Dukor, and L. A. Nafie, Chirality, 2003, 15, 743. CrossRef
2. L. A. Nafie, Nat. Prod. Commun., 2008, 3, 451.
3. K. H. Jang, B. H. Lee, B. W. Choi, H.-S. Lee, and J. Shing, J. Nat. Prod., 2005, 68, 716. CrossRef
4. P. Reddy and S. Urban, Phytochemistry, 2009, 70, 250. CrossRef
5. D. de L. Moreira, E. F. Guimarães, and M. A. C. Kaplan, Phytochemistry, 1998, 49, 1339. CrossRef
6. Y. Kashiwada, K. Yamazaki, Y. Ikeshiro, T. Yamagishi, T. Fujioka, K. Mihashi, K. Mizuki, L. M. Cosentino, K. Fowke, S. L. Morris-Natschke, and K.-H. Lee, Tetrahedron, 2001, 57, 1559. CrossRef
7. (a) N. Iwata, N. Wang, X. Yao, and S. Kitanaka, J. Nat. Prod., 2004, 67, 1106; CrossRef (b) A.-L. Zhang, T. Xu, J.-M. Gao, M.-S. Bai, G. Zhang, H.-W. Liu, S.-Q. Li, and Y. Konishi, Heterocycles, 2009, 78, 1807. CrossRef
8. (a) L. S. M. Veloso, M. J. P. Ferreira, M. I. S. Santos, D. L. Moreira, V. P. Emerenciano, and M. A. C. Kaplan, Phytochemistry, 2006, 67, 492; CrossRef (b) T. Kikuchi, Y. Mori, T. Yokoi, S. Nakazawa, H. Kuroda, Y. Masada, K. Kitamura, and K. Kuriyama, Chem. Pharm. Bull., 1983, 31, 106.
9. M. A. Muñoz, C. Areche, A. San-Martín, J. Rovirosa, and P. Joseph-Nathan, Nat. Prod. Commun., 2009, 4, 1037.
10. C. Areche, A. San-Martín, J. Rovirosa, M. A. Muñoz, A. Hernández-Barragán, M. A. Bucio, and P. Joseph-Nathan, J. Nat. Prod., 2010, 73, DOI: 10.1021/np900553p (Dec.10, 2009). CrossRef
11. K. Terashima, Y. Takaya, and M. Niwa, Bioorg. Med. Chem., 2002, 10, 1619. CrossRef
12. A. Numata, S. Kanbara, C. Takahashi, R. Fujiki, M. Yoneda, Y. Usami, and E. Fujita, Phytochemistry, 1992, 31, 1209. CrossRef
13. (a) L. Tziveleka, D. Abatis, K. Paulus, R. Bauer, C. Vagias, and V. Roussis, Chem. Biodiversity, 2005, 2, 901; CrossRef (b) R. Nahas, D. Abatis, M. A. Anagnostopoulou, P. Kefalas, C. Vagias, and V. Roussis, Food Chem., 2007, 102, 577. CrossRef
14. L. Voutquenne, C. Lavaud, G. Massiot, T. Sevenet, and H. A. Hadi, Phytochemistry, 1999, 50, 63. CrossRef
15. C. Areche, A. San-Martín, J. Rovirosa, J. Soto-Delgado, and R. Contreras, Phytochemistry, 2009, 70, 1315. CrossRef
16. T. B. Freedman, X. Cao, R. V. Olivera, Q. B. Cass, and L. A. Nafie, Chirality, 2003, 15, 196. CrossRef
17. Spartan’04. Wavefunction: Irvine, CA, 2004.
18. Gaussian 03W. Gaussian Inc.: Pittsburgh, PE, 2003.