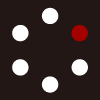
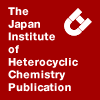
HETEROCYCLES
An International Journal for Reviews and Communications in Heterocyclic ChemistryWeb Edition ISSN: 1881-0942
Published online by The Japan Institute of Heterocyclic Chemistry
e-Journal
Full Text HTML
Received, 14th December, 2009, Accepted, 8th February, 2010, Published online, 9th February, 2010.
DOI: 10.3987/COM-09-11885
■ Gymnastatins I-K, Cancer Cell Growth Inhibitors from a Sponge-Derived Gymnascella dankaliensis
Taro Amagata,* Keiko Takigawa, Katsuhiko Minoura, and Atsushi Numata*
Department of Chemistry and Biochemistry, San Francisco State University, 1600 Holloway Ave., San Francisco, CA 94132, U.S.A.
Abstract
The Halichondria sponge-derived fungus, Gymnascella dankaliensis, was cultured in a malt extract medium containing the bromine source. Three new metabolites, gymnastatins I (5)–K (7), have been isolated from the mycelial MeOH extract. The spectroscopic analyses using 1D and 2D NMR techniques have established the stereostructures of 5–7 in which chlorine atoms in gymnastatins A (1)–C (3) are respectively replaced by bromine atoms. All of the isolated metabolites (5–7) exhibited growth inhibition against the P388 cancer cell line. Furthermore, gymnastatins I (5) and J (6) showed appreciable growth inhibition against the human cancer cell lines.INTRODUCTION
Marine microorganisms are potentially prolific sources of highly bioactive secondary metabolites that might represent useful leads in the development of new pharmaceutical agents. As part of our ongoing search for potential new antitumor materials from marine-derived microorganisms,1-3 we have already reported the structures and cytostatic activities of twelve polyketide-alkaloids, gymnastatins A–C (1–3),4,5 D–G, H (4),4–6 Q and R,7 and dankastatins A and B,7 and six unusual steroids8–10 which were isolated from the fungus Gymnascella dankaliensis OUPS-N134 separated from the sponge Halichondria japonica. All the polyketide-alkaloids except for gymnastatin H (4) have chlorine atoms in the molecule, but no other halogen atoms. It was presumed that this fact was related to halogen sources in culture media because only chlorine ions were used as halogen sources for our previous experiment.4–7 It has actually been reported that the bromo analogues of antibiotics with chlorine atoms were obtained by the substitution of bromide ion for chloride ion in certain media used for the fermentations.11 This consideration prompted us to examine polyketide-alkaloids from this fungal strain in culture conditions using bromine sources, and the fermentation produced polyketide-alkaloids with bromines, designated gymnastatins I–K (5–7). We report herein the isolation and structure elucidation of these compounds together with their growth inhibition against cancer cell lines.12
RESULTS AND DISCUSSION
In the previous experiment,4–7 the fungal strain was cultured at 27 oC for 4 weeks in the malt-glucose-peptone medium in artificial seawater consisting of 2% NaCl, 0.2% KCl, 0.4% MgSO4•7H2O and 0.03% CaCl2•2H2O. In this experiment, the fungal strain was cultured with the same condition in a medium, in which the chlorine ions of artificial seawater were replaced by bromine ions (2% NaBr, 0.2% KBr, 0.4% MgSO4•7H2O and 0.03% CaBr2•2H2O). The MeOH extract of the mycelia was purified by bioassay (P388 cell line)-guided fractionation employing a combination of Sephadex LH-20 and silica gel column chromatography procedures as well as reversed-phase HPLC to afford gymnastatins I (5), J (6) and K (7).
Gymnastatin I (5) had the molecular formula C23H31Br2NO4 established by respective [M – OH]+ and M+ peaks of 5 and its oxidative derivative (8) in HREIMS, and the ratio of relative intensity of isotope peaks (M+ : [M + 2]+ : [M + 4]+ = ca. 1 : 2 : 1) of 8 in EIMS. The UV and IR spectra of 5 exhibited the presence of a hydroxyl group, a conjugated ketone and an amide. The 1H and 13C NMR spectra (Table 1) of 5 suggested that it existed in a 3 : 1 mixture of two stereoisomers (5a and 5b) on a hemiacetal group (δH 5.54, d, 4.4 Hz, and δH 5.62, s; δC 96.5 and δC 103.2). The general features of the 1H and 13C NMR spectra (Table 1) of major isomer 5a closely resembled those of major isomer 1a of gymnastatin A5 except that the signals for H-5, H-9, C-4, C-5, C-6, C-8 and C-9 in 5a revealed a chemical shift difference relative to those of 1a, suggesting that chlorine atoms in 1a were replaced by bromine atoms in 5a. The planar structure of 5a thus deduced from the 1D NMR spectral analysis was confirmed by analysis of 1H-1H COSY and HMBC correlations (H-1/C-4, H-3α/C-5, H-3β/C-4, H-3β/C-9, H-5/C-6, H-5/C-7, H-5/C-9, H-9/C-7, H-9/C-8, H-13/C-11, H-23/C-13) (Table 2).
The relative stereochemistry of the spiro ring in 5a was established by a combination of a coupling constant between H-1 and H-2 (J1,2 4.4 Hz),5 and NOEs from H-2 to H-1, H-9 and H-3α, and from H-3β to H-5 (Table 2). The geometry of the diene and the relative configuration of C-16 in the side chain of 5a were determined by comparison of the NMR data of the side chain of 5a, including 1H and 13C chemical shifts, coupling constants (J12,13 15.3 Hz), and NOEs (H-12/H-23 and H-13/H-15) with those of gymnastatin A (1).5 Based on this evidence, the relative stereostructures for gymnastatin I (5) as a mixture of diasteromers at C-1 were established as 5a and 5b, in which the chlorines in gymnastain A (1) were replaced by bromines. The specific rotation and CD curve of compound 5 were comparable with that of
gymnastatin A (1) {lit,5 [α]D –3.8},13 of which the absolute stereochemistry was previously determined.5 This evidence suggests that the absolute configuration of gymnastatin I (5) is the same as that of gymnastatin A (1).
Gymnastatins J (6) was assigned the molecular formula C24H35Br2NO5 as deduced from a molecular ion peak in HREIMS, and the ratio of relative intensity of isotope peaks in EIMS. The general spectral features of 6 (Table 3) closely resembled those of gymnastatin B (2)5 except that the signals for H-6, H-9, C-4, C-6, C-8 and C-9 in the NMR spectra revealed a chemical-shift difference relative to those of 2. In addition to this evidence, analysis of 1H-1H COSY and HMBC (Table 2) led to planar structure 6 for gymnastatin J.
The observations of a coupling constant between H-1 and H-2 (J1,2 3.7 Hz), and NOEs from H-2 to H-1, H-9 and H-3α, and from H-5 to H-3β and 1-OCH3 in 6 (Table 2) showed that H-1 and H-2 are on the same side as H-9 and H-3α. Also, NOEs from H-5 to H-3β and 1-OCH3 implied that H-5 is oriented to the 1β-methoxy group and hence arranged pseudoequatorially in the half-chair cyclohexene of 6. Furthermore, a NOE from H-6 to 5-OH with a pseudoaxial arrangement indicated that H-6 is arranged
pseudoequatorially and consequently trans to pseudoequatorial H-5 (Figure 1). The stereochemistry of the side chain was determined by comparison of the 1H and 13C NMR data of 6 with those of 5. The above-mentioned evidence allowed assignment of relative stereostructure 6 to gymnastatin J.
Gymnastatin K (7) had the molecular formula C24H37Br2NO6 established by HREIMS. The general spectral features of 7 (Table 3) closely resembled those of gymnastatin C (3) except that the signals for H-6, H-8, C-6, C-7 and C-8 in the NMR spectra revealed a chemical-shift difference relative to those of 3. Analysis of 1H-1H COSY and HMBC (Table 2) led to planar structure of 7. Incidentally, assignments for 5-OH and 9-OH were deduced from their HMBC correlations (5-OH to C-5, and 9-OH to C-4, C-8 and C-9) (Table 2). The observation of an NOE from H-6 to H-8 and the large coupling constant (J8,9 10.9 Hz) between H-8 and H-9 in 7 implied that the cyclohexane ring of 7 exists in a chair conformation with H-6 and H-8 in a coaxial arrangement and with H-8 and H-9 in a trans-axial arrangement. In addition, an NOE from H-5 to 1-OCH3 and the small coupling constant (J5,6 3.4 Hz) between H-5 and H-6 in 7 indicated that H-5 is arranged equatorially, cis to axial H-6 and on the same side as 1-OCH3. Further NOEs from H-2 to H-1 and H-3a implied that these protons are on the same side. The stereochemistry of the side chain was determined by comparison of the 1H and 13C NMR data of 7 with those of 5. The evidence summarized above allowed assignment of relative stereostructure 7 to gymnastatin K.
The absolute configurations of C-16 and C-2 in gymnastatins J (6) and K (7) have not been established independently, but are assumed to be the same as for its co-metabolites, gymnastatins A (1), D, and E, of
which the absolute stereochemistries have already been determined by X-ray crystal structure analyses, modified Moscher’s method, and some chemical transformations.5
The cancer cell growth inhibitory properties of the isolated metabolites were examined using the murine P388 lymphocytic leukemia cell line and a disease-oriented panel of 39 human cancer cell lines (HCC panel) in the Japanese Foundation for Cancer Research.14 All of gymnastatins I–K (5–7) exhibited potent growth inhibition against the P388 cell line (ED50 0.021, 0.021, and 0.21 µg/mL, respectively). In the screening test by HCC panel, the mean log GI50 (MG-MID) of gymnastatins I (5) and J (6) was –5.77 and –5.71 (Table 4), respectively, implying that both the compounds exhibited appreciable growth inhibition. Furthermore, the delta and range values were respectively 0.8 and 1.2 for compound 5, and 0.77 and 1.72 for compound 6, disclosing that both the compounds showed differential activities (effective value: delta ≥ 0.5 as well as range ≥ 1.0). As shown in Table 4, gymnastatins I (5) and J (6) were respectively more effective against HBC-5 (breast), NCI-H522 (lung), OVCAR-3 (ovarian) and MKN1(stomach) cell lines, and against SF-539 (brain), HCT-116 (colon), NCI-H522 (lung), OVCAR-3 (ovarian) and OVCAR-8 (ovarian) cell lines. The above evidence suggested that a conjugated ketone system was important for enhancement of cancer cell growth inhibition in gymnastatins I (5) and J (6), and P388 cell growth inhibition of gymnastatin K (7) resulted from a conjugated ketone which might be derived from itself in the test system.
EXPERIMENTAL
General procedures
Optical rotations were obtained on a JASCO ORD/UV-5 spectropolarimeter. UV spectra were recorded on a Shimadzu spectrophotometer and IR spectra on a Perkin Elmer FT-IR 1720X spectrometer. CD spectra were recorded on a JASCO J-500A spectrometer. 1D and 2D NMR spectra were recorded at 27 °C on a Varian UNITY INOVA-500 spectrometer, operating at 500 and 125.7 MHz for 1H and 13C, respectively, with TMS as an internal reference. EIMS was determined using a Hitachi M-4000H mass spectrometer. Liquid chromatography over silica gel (mesh 230-400) was performed at medium pressure. HPLC was run on a Waters ALC-200 instrument equipped with a differential refractometer (R401) and Shim-pack PREP-ODS (250 mm x 20 mm i.d.). Analytical TLC was performed on precoated Merck aluminum sheets (DC-Alufolien Kieselgel 60 F254, 0.2 mm) with the solvent CH2Cl2-MeOH (19:1), and compounds were observed under a UV lamp and sprayed with 10% H2SO4 followed by heating.
Biological material
The fungal strain (OUPS-N134) was isolated from the sponge Halichondria japonica, collected in the Osaka Bay of Japan, and identified as Gymnascella dankaliensis as previously reported.5–7,9
Culture conditions
The fungal strain was grown in a liquid media (30 L) containing 1% malt extract, 1% glucose, and 0.05% peptone in artificial seawater (1% NaBr, 0.2% KBr, 0.4% MgSO4•7H2O, 0.03% CaBr2•2H2O) adjusted to pH 7.5 for four weeks at 27 °C.
Extraction and isolation
The culture was filtered under suction and the mycelium collected was extracted three times with MeOH.The combined extracts were evaporated in vacuo to give the crude extract (3.8 g). The CH2Cl2–MeOH (1:1)-soluble portion of the crude extract was passed through Sephadex LH-20 using CH2Cl2–MeOH (1:1) as eluent. The second fraction (F1; 2.8 g), in which the activity was concentrated, was chromatographed on a silica gel column with an n-hexane–CH2Cl2–MeOH gradient as eluent. The MeOH–CH2Cl2 (1 : 99) eluate [F2 (250.4 mg)] was further separated by silica gel column chromatography with a CH2Cl2–MeOH gradient as eluent. The MeOH–CH2Cl2 (1 : 99) eluate [F 3 (132.2 mg)] was purified by HPLC (ODS) using acetone–H2O (4 : 1) as eluent to afford 2 fractions [F 4 (21.2mg) and F 5 (50.3 mg)]. Fractions F 4 and F 5 were purified by HPLC using acetone–H2O (7 : 3) and (3 : 1) as eluents, respectively, to afford gymnastatin K (7) (9.2 mg), and gymnastatins J (6) (10.3 mg) and I (5) (27.1 mg), respectively.
Gymnastatin I (5): colorless powder; mp 80.5–81.0 °C; [α]26D –8.5 (c 1.78, CHCl3); UV (EtOH) λmax (log ε) 265 (4.67) nm; CD (EtOH) λmax (Δε) [c 5.22 × 10–5 M] 315 (0), 275 (+2.38), 267 (+2.02), 254 (0), 236 (−2.12), 214 (0); IR (KBr) νmax 3359, 3287 (OH, NH), 1685 (C=C−C=O), 1650 (CONH), 1606 (C=C) cm–1; 1H and 13C NMR data, see Table 1; EIMS m/z 526 [M − OH]+ (1.3), 207 [C14H23O]+ (82.8), 179 [C13H23]+ (94.9), 95 [C5H5NO]+ (100); HREIMS m/z 526.0577 [M − OH]+ (calcd for C23H30Br2NO3, 526.0591).
Gymnastatin J (6): colorless needles; mp 88–89 °C (MeOH); [α]20D –129.5 (c 0.72, CHCl3); UV (EtOH) λmax (log ε) 265 (4.56) nm; CD (EtOH) λmax (Δε) [c 5.03 × 10–5 M] 302 (0), 266 (−13.19), 249 (0), 238 (+4.16), 221 (0), 207 (−3.07); IR (KBr) νmax 3381, 3282 (OH, NH), 1715 (C=C−C=O), 1650 (CONH), 1607 (C=C) cm–1; 1H and 13C NMR data, see Table 3; EIMS m/z 559 [M + 2 – H2O]+ (1.9), 224 [C14H26NO]+ (40.0), 207 [C14H23O]+ (100), 179 [C13H23]+ (85.9), 95 [C5H5NO]+ (76.5); HREIMS m/z 575.0875 [M]+ (calcd for C24H35Br2NO5, 575.0880).
Gymnastatin K (7): colorless powder; mp 116–118 °C; [α]20D –93.9 (c 0.91, CHCl3); UV (EtOH) λmax (log ε) 265 (4.52) nm; CD (EtOH) λmax (Δε) [c 6.00 × 10–5 M] 320 (0), 293 (+0.43), 285 (0), 255 (−3.76), 218 (−2.49), 206 (−2.15); IR (KBr) νmax 3434, 3316 (OH, NH), 1761 (C=O), 1651 (CONH), 1606 (C=C) cm–1; 1H and 13C NMR data, see Table 3; EIMS m/z 595 [M + 2]+ (0.1), 593 [M]+ (0.1), 559 [M + 2 – 2H2O]+ (0.1), 224 [C14H26NO]+ (30.8), 207 [C14H23O]+ (66.7), 179 [C13H23]+ (69.8), 95 [C5H5NO]+ (100); HREIMS m/z 593.0967 [M]+ (calcd for C24H37Br2NO6, 593.0986).
Oxidation of gymnastatin I (5) by a pyridine–CrO3 complex
A solution of gymnastatin I (5) (16 mg) in pyridine (0.3 mL) was added to a pyridine-CrO3 complex prepared from pyridine (0.3 mL) and CrO3 (50 mg), and the reaction mixture was left at room temperature overnight. The mixture was diluted with water and extracted with CH2Cl2. The extract was evaporated under reduced pressure, and the residue was subjected to a silica gel column chromatography [CH2Cl2–MeOH (99 : 1)] followed by HPLC using acetone–H2O (3 : 1) as eluent to afford lactone 8 (2.8 mg) as a colorless powder. Compound 8: mp 73.8–74.5 °C, [α]20D –117.0 (c 0.28, CHCl3); UV (EtOH) λmax (log ε) 265 (4.52) nm; CD (EtOH) λmax (Δε) [c 5.07 × 10–5 M] 303 (0), 266 (−0.95), 245 (0); IR (KBr) νmax 3365, 3288 (OH, NH), 1796 (γ-lacton), 1691 (C=C-CO), 1652 (CONH), 1612 (C=C) cm–1; 1H NMR (CDCl3) δ 0.87 (3H, t, J = 6.9 Hz, H-22), 0.98 (3H, d, J = 6.6 Hz, H-24), 1.21 (2H, m, H-18 or H-19), 1.23 (2H, m, H-21 or H-20), 1.24 (2H, m, H-19 or H-18), 1.26 (1H, m, H-17A), 1.27 (2H, m, H-20 or H-21), 1.35 (1H, m, H-17B), 1.77 (3H, s, H-23), 2.52 (1H, m, H-16), 2.59 (1H, dd, J = 13.6, 10.1 Hz, H-3A), 2.89 (1H, dd, J = 13.6, 10.1 Hz, H-3B), 4.61 (1H, td, J = 10.1, 6.1 Hz, H-2), 5.71 (1H, d, J = 10.0 Hz, H-15), 5.77 (1H, d, J = 15.1 Hz, H-12), 6.15 (1H, d, J = 6.1 Hz, H-10), 7.31 (1H, d, J = 15.1 Hz, H-13), 7.33 (1H, d, J = 2.8 Hz, H-9), 7.53 (1H, d, J = 2.8 Hz, H-5); 13C NMR (CDCl3) δ 12.44 (C-23), 14.08 (C-22), 20.47 (C-24), 22.61 (C-20 or C-21), 27.45 (C-18 or C-19), 29.37 (C-19 or C-18), 31.80 (C-21 or C-20), 33.31 (C-16), 37.18 (C-17), 37.41 (C-3), 49.35 (C-2), 79 16 (C-4), 115.28 (C-12), 123.45 (C-6), 123.69 (C-8), 130.71 (C-14), 145.04 (C-9), 146.78 (C-5), 148.94 (C-13), 149.71 (C-15), 166.89 (C-11), 171.19 (C-7), 172.20 (C-1); EIMS m/z 545 [M + 4]+ (0.1), 543 [M + 2]+ (0.2), 541 [M]+ (0.1).
ACKNOWLEDGEMENTS
This work was supported in part by a Grant-in-Aid for Scientific Research from the Ministry of Education, Science, Sports and Culture, Japan.
References
1. C. Iwamoto, K. Minoura, S. Hagishita, K. Nomoto, and A. Numata, J. Chem. Soc., Perkin Trans. 1, 1998, 449, and references cited therein. CrossRef
2. C. Iwamoto, T. Yamada, Y. Ito, K. Minoura, and A. Numata, Tetrahedron, 2001, 57, 2997, and references cited therein. CrossRef
3. T. Yamada, C. Iwamoto, N. Yamagaki, T. Yamanouchi, K. Minoura, S. Hagishita, and A. Numata, Heterocycles, 2004, 63, 641, and references cited therein. CrossRef
4. A. Numata, T. Amagata, K. Minoura, and T. Ito, Tetrahedron Lett., 1997, 38, 5675. CrossRef
5. T. Amagata, M. Doi, T. Ohta, K. Minoura, and A. Numata, J. Chem. Soc., Perkin Trans. 1, 1998, 3585. CrossRef
6. T. Amagata, K. Minoura, and A. Numata, J. Nat. Prod., 2006, 69, 1384. CrossRef
7. T. Amagata, M. Tanaka, T. Yamada, K. Minoura, and A. Numata, J. Nat. Prod., 2008, 71, 340. CrossRef
8. T. Amagata, K. Minoura, and A. Numata, Tetrahedron Lett., 1998, 39, 3773. CrossRef
9. T. Amagata, M. Tanaka, T. Yamada, M. Doi, K. Minoura, H. Ohishi, T. Yamori, and A. Numata, J. Nat. Prod., 2007, 70, 1731. CrossRef
10. T. Amagata, M. Doi, M. Tohgo, K. Minoura, and A. Numata, Chem. Commun., 1999, 1321. CrossRef
11. E. L. Patterson, W. W. Andres, and L. A. Mitscher, Appl. Microbiol., 1967, 15, 528.
12. A part of this work has been presented orally: T. Amagata, T. Yamada, K. Minoura, and A. Numata, 40th Symposium on the Chemistry of Natural Products, Fukuoka, 1998; Symposium Papers, p 115.
13. In ref 5 unrevised CD data of gymnastatin A (1) were published instead of the following appropriately revised data: CD λ (c 4.40×10-5 M in EtOH)/nm 283 (Δε 0), 271 (−0.76), 259 (0), 246 (+2.41), 234 (0) , 225 (−1.65) and 209 (0).
14. T. Yamori, A. Matsunaga, S. Sato, K. Yamazaki, A. Komi, K. Ishizu, I. Mita, H. Edatsugi, Y. Matsuba, K. Takezawa, O. Nakanishi, H. Kohno, Y. Nakajima, H. Komatsu, T. Andoh, and T. Tsuruo, Cancer Res., 1999, 59, 4042.