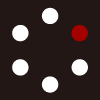
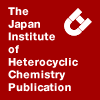
HETEROCYCLES
An International Journal for Reviews and Communications in Heterocyclic ChemistryWeb Edition ISSN: 1881-0942
Published online by The Japan Institute of Heterocyclic Chemistry
e-Journal
Full Text HTML
Received, 20th October, 2009, Accepted, 8th January, 2010, Published online, 15th January, 2010.
DOI: 10.3987/REV-09-662-2
■ Recent Development in Palladium-Mediated Synthesis of Nitrogen Heterocycles
Krishna C. Majumdar,* Buddhadeb Chattopadhyay, Pradip K. Maji, Sudip K. Chattopadhyay, and Srikanta Samanta
Department of Chemistry, University of Kalyani, Kalyani 741 235, West Bengal, India
Abstract
This review describes the synthesis of nitrogen heterocycles by palladium-mediated cyclization. This report covers literature published during 2003 to 2007.CONTENTS
1 Introduction
2 Intramolecular reactions of alkynes, alkenes, allenes, arenes and heteroarenes: Heck, Suzuki, Stille and Sonogashira type reactions
2. 1 Reactions of aryl halides
2. 2 Reactions of enolates
2. 2. 1 Reaction of aryl halides
2. 2. 2 Reactions of vinyl halides
3 Cyclization via C-H bond functionalization reactions
3. 1 Functionalization of alkane C-H bonds
3. 2 Functionalization of aromatic C-H bonds
3. 2. 1 Direct functionalization reactions
3. 2. 2 Functionalization via 1,4-palladium migration
4 Cyclization of 1,n-unsaturated systems: Cycloisomerization and cascade addition-cyclization reactions
4. 1 Cycloisomerization reactions
4. 2 Cascade addition-cyclization reactions
5 Cycloaddition reactions
5. 1 [3+2] Cycloaddition reactions
5. 2 [3+3] Cycloaddition reactions
5. 3 [4+2] Cycloaddition (Diels-Alder reactions)
5. 4 [2+2+1] Cycloaddition reactions
6 Heterocyclization reactions: Cyclization via carbon-heteroatom bond formation
6. 1 Intramolecular addition of N-H and C=N bonds across alkene, allene and alkyne
6. 1. 1 Addition to alkene
6. 1. 2 Addition to allene
6. 1. 3 Addition to alkyne
6. 2 Intra- and intermolecular coupling of NH with vinyl and aryl halides or triflates
6. 2. 1 Aryl halides or triflates
6. 2. 2 Vinyl halides or triflates
6. 3 Cyclocarbonylation reactions
6. 4 Reductive N-heterocyclization of nitroarene
6. 5 Amino Heck reaction
7 Cyclization via cascade carbon-carbon/carbon-heteroatom bond formation: Heterocyclization reactions
7. 1 Heterocyclization reactions with alkynes
7. 1. 1 Reactions with terminal alkynes: Sonogashira coupling-cyclization reactions
7. 1. 2 Reactions with internal alkynes
7. 2 Heterocyclization reactions with alkenes
7. 3 Heterocyclization reactions with dienes
7. 4 Heterocyclization reactions with carbon-heteroatom unsaturated bond
7. 5 Heterocyclization with arenes
8 Palladium-catalyzed multi-component reaction
8. 1 Palladium-catalyzed three-component reaction
8. 2 Palladium-catalyzed four-component reaction
9 Miscellaneous
9. 1 Cyanoamidation reaction
9. 2 Ring-opening cyclization reaction
10 Conclusion
11 Acknowledgements
12 References
1. INTRODUCTION
Recently, there is flurry of activities in the synthesis of heterocyclic compounds due to their pharmacological and biological activities. Synthetic organic chemists are continuously after the development of novel and more effective synthetic methodologies. Many of the methodologies involve the formation of carbon-heteroatom bond from the corresponding acyclic precursors. Among newly developed protocols, the application of palladium catalysis in the synthesis of heterocycles is extremely useful and are now routinely considered in planning of complex target molecules.
A good number of books1 and reviews2 covering general, particular and limited aspects of the chemistry of organopalladium are available. Organopalladium chemistry has already resulted in a large number of name reactions for the formation of C-C, C-O, and C-N bonds under relatively mild conditions. The catalysts are generally air stable, tolerant of a wide range of functionalities which avoids protection-deprotection processes. The use of chiral ligands3 in asymmetric transformation for the synthesis of heterocycles and the palladium catalyzed domino reactions4 are quite significant in organopalladium chemistry.
Palladium in three oxidation states, Pd(0), Pd(II) and Pd(IV) can exist in palladium complexes. Fortunately, there is facile interconversion between Pd(0) and Pd(II) oxidation states due to small energy difference between them. The ability of interconversion between the aforesaid two oxidation states is responsible for wide use of palladium in organic synthesis. Palladium(0) oxidation state is synthetically most useful and is also very important for the synthesis of heterocyclic compounds. Palladium(II) complexes are very important in organopalladium chemistry. They are stable in air, soluble in most common organic solvents and electrophilic in character. Electron-rich olefins, alkynes and arenes are the common substrates for Palladium(II). Some useful Pd(II) complexes are PdCl2(PPh3)2, Pd(OAc)2, PdCl2(RCN)2, Pd(PPh3)4. Usually Pd(II) complex is added to the reaction mixture which is readily reduced by various species to Pd(0) and catalyzes the reaction.
The synthesis of various heterocycles and new protocols involving palladium has been the central goal in recent years. Therefore, our objective is to provide a complete and updated summary of palladium-catalyzed protocols for the preparation of nitrogen heterocycles developed from 2003 onwards with the emphasis on the underlying principle following each synthetic procedure, the results and the appropriate choice of reaction conditions. We have also avoided a thorough review of the patent literature which is beyond the scope of this review.
2. INTRAMOLECULAR REACTIONS WITH ALKYNES, ALKENES, ALLENES, ARENES AND HETEROARENES: HECK, SUZUKI, STILLE AND SONOGASHIRA TYPE REACTIONS
The potentiality of C-C unsaturated bond as carbon source to get coupled with aryl- and vinyl halides or organometallics in a palladium mediated reaction is well documented.2,5 Heck reaction1a,2a,6 is significant in the synthesis of heterocycles. The intramolecular version of this reaction with aryl or vinyl halides generally proceeds through a sequence of oxidative addition to C-X (X = halogen) bond, insertion and β-elimination (for olefins) or protonolysis (for alkynes) to generate the heterocycles ranging from small to macrocyclic compounds. The observed rate of oxidative addition with C-X bonds decreases according to the following order: C-I> C-Br> C-Cl> C-F.7 The reactivity of aryl triflates is in between that of aryl iodides and aryl bromides. Additives also play a important role in controlling the results of palladium-catalyzed reactions.8 Recently intramolecular reactions have been developed involving related carbopalladation reactions followed by trapping with nucleophilic reagents.9
For macrocyclization via C-C bond formation with olefin or alkyne, the well-known Heck, Suzuki, Sonogashira and Stille reactions are now routinely considered.10 A different approach to C-C bond formation involving the palladium-catalyzed intramolecular reaction of enolate derived from ketones, esters and amides with aryl or vinyl halide11 or oxidative coupling with olefin12 has been utilized for the synthesis of five- and six-membered heterocyclic compounds.
2.1. REACTIONS OF ARYL HALIDES
In palladium-catalyzed Heck reaction, aryl iodide has been found to be the most commonly used halide source.13,14 In an investigation, Liu et al. reported the use of aryl bromide and chloride 1a-d and 3a-f for the synthesis of a number of 1,2,3,4-tetrahydroisoquinolines 2a-d, indolines 4a-f under ligand free Pd(OAc)2–catalyzed reductive Heck15 cyclization (Scheme 1).16 The reaction was robust in the presence of water as up to 5% water. Although the ligand free condition was efficient for bromobenzene, chlorobenzene showed low reactivity in the absence of an activating group on the aromatic ring. However, an excellent improvement in the yield of 4e (87%) was observed under the Buchwald condition.17 By employing various palladium complexes [(t-Bu)2P(OH)]2-PdCl2, [(t-Bu)2P(OH)(t-Bu)]2-PdCl2 and [(t-Bu)2P(OH)-PdCl2]2 developed by Li,18 a 99% yield of 4f was obtained from 3f.
The compound 5 when subjected to intramolecular Heck reaction using Pd(OAc)2 as catalyst, afforded the cyclized product 6 in 34% yield along with sizable amount of 2,4-dimethylisoquinolin-1(2H)-one 7 and 4-formyl-2-methylisoquinolin-1(2H)-one 8 (Scheme 2).19
Stephenson et al. extensively investigated the intramolecular asymmetric Heck reaction3,20 of o-iodoanilide 10 for the synthesis of oxindoles 11 by employing a wide range of conditions (Scheme 3).21
The conversion rate and ee data clearly indicated a general tendency for high ee’s at high temperature and relatively low ee’s at low temperature. The limiting ee’s at 100 oC indicated the generation of catalytically active Pd(0) particles by thermal decomposition of Pd2(dba)3 [(R)-BINAP] intermediates resulting in a competing racemic cyclization over time.
The X-ray crystallographic study of the substrate 22a revealed the presence of enantiomerically pure (P)- and (M)-helical structures (Scheme 4). Here it is important to note that Curran and co-workers22 also synthesized 2-oxindole by radical-mediated intramolecular cyclization and found that the stable amide atropisomers play a significant role in the control of intramolecular Heck reaction. The dynamic kinetic resolution of interconverting helical iodide (P)- and (M)-12a must favor the oxidative addition of (M)-helix to promote the pro-(R) pathway to product 13a. However, in the case of Ag3PO4 protocol, the rationalization for the opposite stereochemical preference is due to the equilibrium between palladium bound oxidative addition complexes of the (P)- and (M)-helices [(P)/(M)-14a]. The (S)-(-)-13a product was formed through the pro-(S) catalytic intermediate, the access of which has been enhanced by Ag3PO4.
The equilibrium between the two atropisomeric forms of 12a is crucial for an efficient asymmetric induction in the Heck cyclization which is evident from the formation of product 16 with an ee of only 10% from the imide substrate 15 containing two 2-methylbut-2-enyl groups (Scheme 5).
Intramolecular Heck reaction of 2'-bromo-N-(2-methyl-1,3-dioxo-2,3,3a,4,7,7a-hexahydro-1H-isoindol-4-yl)benzamide 17 was investigated under two different conditions.23 In both the conditions the imide 17 underwent 6-exo-trig cyclization. In dioxane, 2-methyl-3b,9b,10,11-tetrahydro-1H-pyrrolo[3,4-c]-phenanthridine-1,3,5-(2H,4H)-trione 18, was obtained. Whereas the reaction in DMF afforded 2-methyl-4,10,11,11a-tetrahydro-1H-pyrrolo[3,4-c]phenanthridine-1,3,5(2H,3H)-trione 19 (Scheme 6).
The intramolecular Heck reaction of α,β-unsaturated 2-haloanilides derived from azatricyclo[4.4.0.02,8]decanone was used to introduce the congested spirooxindole functionality of gelsemine.24 Depending upon the reaction conditions, two epimeric products 21 and 22 were obtained from anilides 20a and 20b possibly via migratory insertion from the α-face (TS I) and β-face (TS II) (Figure 1) respectively (Scheme 7). The extra stability of TS-II is due to the coordination with angular vinyl group. An examination of the catalytic conditions revealed that anilides 20a and 20b when subjected to catalytic system Pd(PPh3)4/Et3N/MeCN afforded spiro oxindoles 21 and 22 in 66:34 and 60:40 ratios. However, cyclization of α,β-unsaturated anilide 20b catalyzed by tris(dibenzylideneacetone)dipalladium [Pd2(dba)3] in the absence of phosphine ligands was more selective. In contrast, Heck cyclization of the precursor 20b conducted in the presence of Ag3PO4 and in the absence of phosphine ligands occurred with virtually complete selectivity to give the epimeric oxindole 22.
The intramolecular Heck reaction of β-methylthio-α,β-unsaturated anilide 25 and β-methoxy-α,β-unsaturated anilide 28 was also examined. Under forcing conditions (150 °C) using Pd2(dba)3.CHCl3 as the catalyst, the intramolecular Heck reaction of 25 produced a 1:2 mixture of stereoisomeric tetracyclic products 26 and 27 in 69% yield. (Scheme 8). However, in the case of 28, a high preference for the formation of 29 was observed using 35 mol% Pd2(dba)3 and Ag3PO4 in refluxing THF.
The intramolecular Heck reaction of the amide 31 under microwave irradiation and employing the same combination of catalysts gave benzoazepine 32 in excellent yield. In contrast, the intramolecular Heck reaction of resin bound amide 33, performed under microwave and standard heating conditions, afforded the benzolactam 34 in moderate yield after washing of the resin followed by methylation (Scheme 9).25
Weinreb and Artman26 reported a halogen-selective domino intramolecular Heck/carbonylation reaction for the construction of an indole moiety with quaternary stereocenter, found in metabolite perophoramidine. The Pd(OAc)2-catalyzed process worked efficiently and compounds 36a,b were obtained from the precursors 35a,b as single diastereomers (Scheme 10).
Fuwa et al. recently reported the first application of acyclic α-phosphoryloxy enecarbamates to the synthesis of indole-2,3-quinodimethanes and 2-(N-alkoxycarbonylamino)-1,3-dienes and demonstrated their efficiency in the Heck reaction.27 The same group, in a recent investigation, has reported the use of α-phosphoryloxy enecarbamates 37 in Pd(0)-catalyzed intramolecular Heck2,28 reaction for the preparation of indole derivatives 40. Thus, N-(o-bromophenyl) enecarbamates 39, prepared from the acyclic α-phosphoryloxy enecarbamates 37 by Suzuki-Miyaura cross coupling afforded 2-phenylindole derivatives 39 when subjected to Pd(PPh3)4, Et3N in DMF (Scheme 11).29
Regioselective construction of medium-sized benzolactams has been successfully achieved by intramolecular Heck reaction.30 The regiochemistry of the reaction is highly dependent on the catalytic condition as both exo30a,b and endo30c products can be formed. An interesting example of ligand and temperature effect on the regioselectivity of the intramolecular Heck reaction was reported by Hii et al.31 The 7-exo-trig cyclized product benzazepine 42 was obtained exclusively when the amides 41a,b were subjected to Pd(OAc)2 or Pd2(dba)3.CHCl3 as catalyst at 140 oC employing ligands PPh3 or dppp or dppf (Scheme 12). A competitive formation of 8-endo-trig cyclized products 43 and 44 as an inseperable mixture along with compound 42 was observed by employing 3 equiv of PPh3 (51-60%). Replacement of PPh3 with the P(o-tol)3 ligand led to an improvement in the yield of 42 (80%). An exclusive formation of 42 in excellent yield was observed by using 4 equiv of PPh3 at 130 oC (88%).
Similarly, compounds 45a-c in the presence of Pd2(dba)3.CHCl3 as catalyst and PPh3 as ligand in a ratio 1:4 underwent cyclization in an exo-manner to afford quinolinone 46, 1-benzazepinone 47 and 1-benzazocinone 48 (Scheme 13).32 However, under ligand free Jeffery’s condition, compound 45b afforded an inseparable mixture of benzapinone and its double bond isomer 47 in a 1:1 ratio.
The intramolecular Heck reaction for the synthesis of a series of a novel benzazepines 50 (Scheme 14)33 was carried with Pd(OAc)2 catalyst and K2CO3 in the absence of any posphine ligand. The amine 49 afforded the benzazepine 50. Interestingly, PEG 3400 acted both as solvent as well as a stabilizer for in situ generation of nanoparticles, thus influencing the outcome of the reaction in terms of selectivity.
The intramolecular Heck reaction of indole derivative 51a in the presence of Pd(OAc)2, P(o-tol)3, Et3N, afforded both 9-endo-53 and 8-exo-52a products.34 The substrate 51b under the same reaction conditions afforded 8-exo-52b as the isolable product. Moreover, the intramolecular Heck reaction of compound 54 employing Pd(OAc)2, P(o-tol)3, DBU afforded 10-membered trans-macrocycle 55 (Scheme 15).
N-Aryl and N-ethynyl-2-iodobenzamides 57a,b underwent intramolecular hydroarylation reaction on treatment with a catalytic amount of Pd(OAc)2 and PPh3 to afford 3-methyleneisoindolin-1-ones 59a,b via the intermediate 58 (Scheme 16).35,36 Here ammonium formate (HCO2NH4) acts as the reducing agent in order to regenerate the Pd(0) catalyst from the intermediate 58. Similarly, by the application of domino-coupling37 the same type of products with terminally aryl substitution may be obtained.
An efficient Pd(OAc)2-PPh3-catalyzed method for the domino reaction of alkynamides 60 was demonstrated by Takemoto et al.38 The efficiency of the catalytic system was explored in the synthesis of various (E)-, (Z)-, and disubstituted 3-alkylideneoxindoles 61 and 62 in excellent yields (Scheme 17).
Using a small screening set of different catalysts Player et al.39 demonstrated that domino carbocyclization/Suzuki coupling of alkynylamide 63 under both microwave and ambient temperature afforded the desired (E)-3,3-(diarylmethylene)indolines 65 in 48-78% yields. More activating carbene ligand precursor 6640 and Buchwald’s ligand, 2-dicyclohexylphosphinobiphenyl ligand 6741 were found to be less effective to promote the reaction even under the microwave condition (Scheme 18).
A new synthetic strategy based on the microwave-assisted intramolecular hydroarylation was developed for the synthesis of medium-sized nitrogen heterocycles.42 Thus seven-membered heterocycles 69 were synthesized in moderate-to-good yields starting from propynoic acid amides 68 using Pd(PPh3)4 and HCO2Na (Scheme 19).
Pd(OAc)2-PPh3-catalyzed intramolecular carbopalladation of 1-(2-bromobenzyl)-1H-imidazole 70a was carried out to afford fused heteroaromatic compounds, 5H-imidazo[5,1-a]isoindole 71a.43 Attempted reaction at higher temperature afforded better yield of 71a (Scheme 20). Similarly, 5H-imidazolo[2,1-a]-isoindoles44 may also be synthesized by intramolecular Heck reaction sequence.
We have utilized the Jeffery’s two-phase protocol for the construction of a number of tetracycyclic coumarin-annulated nitrogen heterocycles from 72a-f (Scheme 21).45 By using Pd(OAc)2 as catalyst, KOAc as base, and n-Bu4NBr as additive in DMF a series of tetrahydropyrido[2,3-c]coumarin derivatives 73a-f were synthesized.
An efficient route for the construction of complex heterocycle 75 containing benzazepine moiety has been achieved via an intramolecular biaryl coupling reaction at position 2 of the heterocyclic systems such as 74 (Scheme 22).46
Grigg and co-workers recently reported47 an interesting observation during the course of cyclization of the substrates cis-76a and trans-76b by the palladium-catalyzed intramolecular Heck reaction. When the cis-isomer 76a was treated with a catalytic system comprising Pd(OAc)2, PPh3, n-Et4NCl and K2CO3, the corresponding exo-cyclized product 77 was obtained with exclusively (Z)-configuration due to the cis-addition of ArPdI to the styryl moiety followed by cis-β-hydride elimination. The possible explanation is that the conformational differences in the pyrazolidine moiety of the chelated oxidative addition product 78 may destabilize trans-isomer to the extent that the cyclization is disfavored (Scheme 23).
Similarly, tripeptides having 3-bromobenzylamino group at the C-termini and an acryloyl group at the N-termini 79 underwent intramolecular Heck reaction utilizing Pd(OAc)2/P(o-tol)3/EtN(i-Pr)2 to afford the corresponding cyclic peptide 80 (Scheme 24).48
Sonogashira10 coupling was exploited for the solid-phase synthesis of a cyclic peptide containing 21-residue epitope present in the A-B loop of the C-3 domain of human immunoglobulin E.49 The key step to construct the 65-membered ring 82 was achieved in 13% yield from the corresponding acyclic precursor 81 on treatment with Pd(PPh3)4-CuI-PPh3-Et3N catalytic system in DMF (Scheme 25).
Copper-free Sonogashira coupling was utilized for the macrocylization of di- and tri-peptides 84 to give cyclic peptides 85 with rigid linkers leading to unusual peptidometrics50 (Scheme 26).
An efficient route for the stereocontrolled synthesis of bicyclic β-lactams via palladium-catalyzed intramolecular Heck reaction51 was best performed using Pd(OAc)2, PPh3 and K2CO3 in DMF. The regiochemistry of the reaction was observed to be highly influenced by the reaction temperature (Scheme 27). At lower temperature exclusive formation of 8-endo-trig cyclized product 87 along with very little or no formation of 7-exo-trig cyclized product 89 was obtained from the precursors 86. At higher temperature, the reaction afforded isomeric bicycle 88 along with 89.
Ohno and Tanaka demonstrated that bromoenyne 90 when treated with Pd(OAc)2 and Cs2CO3 in EtOH underwent tandem cyclization to give the tricyclic heterocycles 91 in good yields (Scheme 28).52
2.2 REACTIONS OF ENOLATES
Palladium-catalyzed intramolecular coupling reaction of tethered vinyl or aryl halides and ketone enolates provides a useful route to a wide variety of heterocycles.11,53
2.2.1 REACTIONS OF ARYL HALIDES
α,β-Unsaturated-γ-lactam 92 containing a haloaryl moiety tethered to nitrogen on treatment with Pd(OAc)2 in DBU underwent cyclization to afford five- and six-membered ring conpounds 93 (Scheme 29).54 A plausible mechanism55 for this reaction involves the formation of the palladium complex 94 followed by transmetallation to give 95 and reductive elimination to afford 93.
Intramolecular Heck reaction of aryl iodide 96a and 96b on to β,γ-unsaturated nitro-derivative in with Pd(PPh3)4 as catalyst gave different results depending on the nature of the base used (Scheme 30).56 While KOPh gave the cyclized compounds 97a, 97 and 97b in good yields KOt-Bu proved completely ineffective, giving either complex reaction mixtures or the dehalogenation product 98 instead of the cyclized product 97b.
2.2.2 REACTIONS OF VINYL HALIDES
β,γ-Unsaturated nitro-derivatives57 as enolate type nucleophile were found to be efficient terminators in the palladium-catalyzed intramolecular coupling with amino-tethered vinyl halide 99. Pd(PPh3)4-catalyzed transformation afforded four different products 100-103. The product formation is dependent on the nature and amount of the base used.56 Potassium phenoxide (KOPh) is the base of choice in these reactions as it gives exclusively the desired 2-azabicyclo[3.3.1]nonane moiety 101. Tertiary butoxide (KOt-Bu) and Cs2CO3 afforded the bridged 1,4-elimination product 100 along with normal Heck product hydroindole 102. The dimer 103 was obtained when 2.5 equiv of KOt-Bu was used as a base (Scheme 31).
The formation of bridged compounds 101 clearly indicated the involvement of sequential nucleophilic substitution on palladium by the nitronate, reductive elimination and base promoted isomerization. However, in the presence of Pd(0), compound 101 may generate a π-allyl complex by oxidative addition which via 1,4-elimination pathway58 may give the compound 100.
Bonjoch et al. reported the synthesis of 4-azatricyclo[5.2.2.04,8]undecan-10-ene core of the natural product calyciphylline A where palladium-catalyzed intramolecular enolate alkenylation was utilized as the key step.59 Thus compound 104 containing an amino-tethered vinyl bromide with a ketone when subjected to Pd(PPh3)4 and KOPh afforded the tricylic core system 105. The compound 105 was converted in several steps to the target azatricyclic ketone 106 (Scheme 32).
Regio-and stereoselective formation of γ-lactam could be achieved via the intramolecular 5-exo reaction between stabilized acetamide enolate anion with properly tethered η3-allyl-palladium appendage.60 An investigation on the free enolate alkylation by phase transfer catalysis61 compatible with the palladium catalysis62 revealed that the use of n-Bu4NBr as catalyst, [Pd(C3H5)Cl]2 as palladium source, dppe as ligand, and KOH as base, in a biphasic CH2Cl2/H2O (1/1; v/v) system, resulted in the formation of hexahydro indole derivatives in high yield.63 The cyclization of 109 with crown ether 15-C-5 (1.2 equiv) under the same reaction conditions {NaH/DMF/Pd(OAc)2/dppe-based} afforded the hexahydroindole derivatives 110 in moderate-to-good yields (Scheme 33). Remarkable efficiency of these new cyclization conditions may be due to the formation of highly reactive zwitterionic64 enolates/η3-allyl intermediates 111 under either biphasic PTC conditions or Na-sequestered homogeneous conditions (Scheme 34).65
The oxidative cyclization of various N-alkenyl β-keto amides 113 utilizing Pd(II) catalyst and Yb(OTf)3 as lewis acid has been reported.66 The reaction underwent through enol formation and intramolecular attack of neucleophilic enol towards Pd(II)-activated olefin67 (Scheme 35).
3. CYCLIZATION VIA C-H BOND FUNCTIONALIZATION REACTIONS
Palladium-catalyzed functionalization of C-H bond68 has undergone a rapid development over the past decade.69 The potentiality of this excellent protocol has proven to be extremely useful for the synthesis of a wide variery of N-heterocycles under mild conditions.70 In general the cyclization via aromatic C-H functionalization proceeds through Pd(IV) intermediate generated by electrophilic palladation on second aromatic or heteroaromatic ring followed by reductive elimination of palladium to afford the heterocycles.71
3.1 FUNCTIONALIZATION OF ALKANE C-H BONDS
The development of catalytic system for direct functionalization of alkane sp3 C-H bond is of considerable interest.72 The sp3 C-H bond adjacent to amines are realatively activated and can be functionalized under special condition.73 Shi et al. reported an unusual formation of pyrrole 116 in the multiple deprotonations and deaminations of phenethylamines 115.74 The reaction was carried out in the presence of PdCl2, Cu(OAc)2 in refluxing PhMe. The efficiency of the transformation seemed to be highly dependent on the electronic effects of the substituents on the aromatic ring and the trisubstituted pyrrole derivatives were produced (Scheme 36).
3.2 FUNCTIONALIZATION OF AROMATIC C-H BONDS
3.2.1 DIRECT FUNCTINALIZATION REACTIONS
The palladium-catalyzed direct functionalization of aromatic or heteroaromatic C-H bond75 via C-H activation is a versatile way to generate a wide variety of N- and O-heterocycles under mild conditions.76 Pd(II)-catalyzed C–H bond activation followed by oxidative cyclization of diaryl amine77 117 was utilized for the construction of the 9H-carbazole skeleton 118. The reaction was carried out by utilizing Pd(OAc)2 and an excess of Cu(OAc)2 in AcOH to afford the carbazole derivative (Scheme 37).
It was observed that by employing a single catalytic system Pd(OAc)2/P(t-Bu)3-HBF4/K2CO3 in the presence of styrene, compound 119a,b underwent domino intramolecular Heck reaction and direct arylation reaction to give the products 121a,b in one pot78 (Scheme 38).
The intramolecular arylation of 3-(2-bromophenylamino)quinoline 122 employing Pd(PPh3)2Cl2 as catalyst and NaOAc.3H2O as base was tested both under heating and microwave irradiation.79 Although, in both the cases 7H-indolo[2,3-c]quinoline 123 was obtained as the major product, microwave irradiation process was found to be superior to normal refluxing condition (Scheme 39).
An extensive investigation80 of the effect of catalyst, base and temperature on the palladium-catalyzed intramolecular arylation to give 5-methylfuro[3,2-c]quinolin-4(5H)-one has recently been reported. The condition developed by Kuroda and Suzuki81 was found efficient as the desired compound 125 was obtained in 83% yield from the amide precursor 124 (Scheme 40). When the reaction was performed using PdO catalyst and potassium acetate as base in polar solvent DMA at 150 oC the desired tricyclic compound was obtained in 89% yield.
Lautens et al. developed a new and straightforward Pd(OAc)2-catalyzed, norbornene-mediated one step approach to highly substituted six- and seven-membered annulated indoles 128a,b using readily available starting materials N-brormoalkylindoles 126a,b and aryl iodide 127.82 The basic strategy of the synthesis was based on domino aromatic alkylation/aryl-heteroaryl coupling and also involved an aromatic sp2 C-H activation to generate heterocycles 128 in moderate-to-excellent yields (Scheme 41).
Similarly, this domino aromatic alkylation/aryl-heteroaryl coupling strategy was also applied to the synthesis of a number of pyrrole-annulated six- and seven-membered heterocycles83 (Scheme 42).
Substituent effect on the regioselectivity of domino annulation of pyrrole with norbornene was also reported.84 With unsubstituted pyrrole 132, the annulation in the presence of Pd(OAc)2 as catalyst, PPh3 and Cs2CO3 proceeded efficiently to afford the polycyclic heterocycle 133 (Scheme 43).
The norbornene-mediated and Pd(OAc)2-catalyzed reaction of 135 and 134 underwent annulation via three C-H activation in the presence of PPh3 as ligand to furnish the pentacyclic indole-annulated heterocycles 136 (Scheme 44).85
A highly efficient palladium-catalyzed synthesis of unsymmetrically substituted 3-(diarylmethylenyl)indolines 139 has been developed by the reaction between N-protected-N-aryl-3-phenylpropiolamide 137 and 2-iodonitrobenzenes (Scheme 45).86 Under aforesaid reaction conditions the cyclization of differently substituted diaryl alkynyl amide occurred preferably at the electron poor benzene ring which is incompatible with the SEAr mechanism for the C-H activation step.87,88
The intermolecular carboannulation of 1-(2-bromophenyl)-1H-indoles 140 with o-trimethylsilyl phenyltriflate in the presence of Pd2(dba)3.CHCl3 as catalyst and dppp as ligand using mixed solvent MeCN-PhMe (1:1) afforded indolo[1,2-f]phenanthridine 142 (Scheme 46).89 The reaction is assumed to proceed via the formation of benzyne intermediate, generated in situ via elimination of o-trimethylsilyl phenyltriflate by CsF. The Pd(0) complex may undergo the oxidative addition90 with 1-(2-bromophenyl)-1H-indole to give the arylpalladium intermediate which may then get converted to intermediate 143 via a palladacycle intermediate. Reductive elimination from intermediate 143 may generate the product 142.
A short synthesis of rhazinilam 149,91 a potent anticancer agent, was developed by Trauner et al.92 The key cyclization step for the construction of the nine-membered lactam ring was achieved by heating 144 with Pd(OAc)2 and Buchwald’s ‘davephos’93 ligand 145 (Scheme 47).
The cyclization reaction may proceed through intramolecular nucleophilic attack of the pyrrole moiety onto the Pd(II) center in 146 followed by deprotonation94 and reductive elimination of Pd(0) from the complex 147 leading to the formation of aryl-heteroaryl bond. Finally, rhazinilam 149 was obtained from 148 in few steps.
Tanaka et al. reported direct construction of fused aromatic ring systems by palladium-catalyzed “zipper-mode”95 double C-H bond activation process. Treatment of 150 with a catalytic amount of Pd(OAc)2 and PCy3.HBF4 in the presence of Cs2CO3 in dioxane afforded96 4,5-naphtho[3,2,1-cd]indole derivatives 151 (Scheme 48).
3.2.2 CYCLIZATION VIA 1,4-PALLADIUM MIGRATION
Excellent ability of palladium to insert into unactivated C-H bond is of great interest as it affords wide variety of useful synthetic processes.69 The through-space palladium rearrangement with simultaneous C-H activation provides a novel way to introduce palladium into a specific location within organic molecules. Recently, Larock et al. have applied the nitrogen directed vinylic to aryl palladium migration strategy involving domino C-H activation process for the synthesis of carbazole derivatives.97 The synthetic studies were carried out in the presence of Pd(OAc)2, bis(diphenylphosphino)methane (dppm) and CsO2CCMe3 (CsPiv) in DMF.98 The reaction with symmetrical alkynes afforded the carbazole 154a and 154b exclusively from the starting materials 152a and 152b respectively. However, with an asymmetrical alkyne the formation of two regioisomeric compounds 155a+156a in a 10:1 ratio was obtained (Scheme 49).
The same group also showed that the reaction between N-allyl-3-iodoaniline 157 under the same reaction conditions furnished the indole 159 as a single diastereoisomer where as the same reaction with unsymmetrical alkyne generated two isomeric indole derivatives 159 and 160 in 10:1 to 15:1 ratios respectively99 (Scheme 50).
An indole derivative 162 was efficiently synthesized from 3-iodo-1-(4-tosyl)indole 161 in the presence of norbornene as olefin source. The observed stereochemistry of the compound was due to initial cis- addition of indol-3-yl palladium iodide to norbornene100 (Scheme 51).
4. CYCLIZATION OF 1,n-UNSATURATED SYSTEMS: CYCLOISOMERIZATION AND CASCADE ADDITION-CYCLIZATION REACTIONS
4.1 CYCLOISOMERIZATION REACTIONS
Palladium-catalyzed cycloisomerization reaction has proven as one of the versatile process to construct cyclic compounds from the acyclic 1,n-unsaturated precursors.101 In general, 1,6-unsaturated system affords five-membered cyclic product. The cycloisomerization of enyne 163 in the presence of palladium source Pd2(dba)3.CHCl3/PPh3/AcOH afforded five-membered heterocycles 164 (Scheme 52).102 By utilizing this protocol, a number of five-membered nitrogen heterocycles were synthesized.
Zhang and collaborators extensively investigated the palladium-catalyzed domino cycloisomerization/Suzuki coupling of 1,6-enynes.103 By utilizing Pd(PPh3)4 as catalyst, both electron rich (165a) and electron poor (165b) enynes underwent this cascade cyclization-coupling reaction to afford five-membered heterocycles 166a and 166b with an exo double bond (Scheme 53).
hno et al. reported Pd(0)-catalyzed stereoselective cyclization of allene 167a for the synthesis of 2,3-cis-pyrrolidines 168a.104 The cyclization in the presence of iodobenzene was achieved using Pd(PPh3)4 and K2CO3 in refluxing dioxane to give compound 168a. Internal allene 167b was also similarly cyclized into five-membered ring 168b. The observed (Z)-geometry of the double bond of 168a might be a consequence of thermodynamic preference for the syn-π-allylpalladium(II) intermediate over other isomers (Scheme 54).105
However, under a different reaction condition [Pd2(dba)3.CHCl3 in MeCN at 80 oC] allenenes 169c underwent steroselective cyclization-cyclopropanation in the presence of allyl carbonate to afford 3-azabicyclo[3.1.0]hexane 170c (Scheme 55).106 The catalytic cycle for the formation of compound 170c is suggested to proceed via generation of an unusual palladium carbene intermediate. However, an alternative route via alkyl palladium intermediate may also lead to the cyclopropane derivative.
Indolylcarbonates 171 on treatment with Pd2(dba)3.CHCl3 as catalyst and DPPBA-based ligand (S,S)-173 commonly known as Trost ligand afforded 4-vinyl-1,2,3,4-tetrahydro-β-carbolines 172 in high ee (Scheme 56).107 Pyrrolyl-based polycyclic systems has been synthesized. It is interesting to note that quaternary stereocenter is also accessible through this new approach.
1,6-Diynes 174 bearing a conjugated enone moiety underwent cycloreduction108 on treatment with Pd(PPh3)4 and formic acid to afford 2-(2-methylenecycloalkyl)furans 175 in good-to-excellent yields (Scheme 57).109
The acyclic peptides 176 with varying chain length and amino acid units underwent enyne cycloisomerization to give constrained small cyclic peptides 177 with novel linkers (Scheme 58).110 The reactions were carried out using Pd(OAc)2 as catalyst and P(o-tol)3 as ligand. It was anticipated that the linker length might play a role in the E/Z selectivity of the endocyclic double bond in products 177. However, E-stereochemistry of the endocyclic double bond and S-transoid form of the 1,3-dienes in the cyclic peptide were observed in all the cases.
4.2 CASCADE ADDITION-CYCLIZATION REACTIONS
Intramolecular acetoxypalladation-initiated cyclization of N-allylic alkylamides 178 for the syntheses of alkylidine-γ-butyrolactams 179 was developed111 by employing Pd(OAc)2 as catalyst (Scheme 59).
An expedient route to the synthesis of stereo-defined α-halomethylene-γ-butyrolactones, lactams and tetrahydrofurans via PdCl2-catalyzed cis-chloropalladation-cyclization of 1,6 enynes 180a in AcOH was developed by Zhang et al.112 The reaction showed excellent stereoselectivity (E/Z > 99/1) as only five membered (E)-181 were obtained in good yields (Scheme 60).
Lewis acid additive showed high influence on the diastereoselectivity of the intramolecular allyl transfer reaction of allenic aldehydes 182 with hexamethylditin catalyzed by (π-allyl)2PdCl2 (Scheme 61).113 Moderate-to-good yields of trans-products 184 were obtained by using B(C6F5)3 as Lewis acid additive. In the absence of any additive cis-products 183 were obtained in good-to-excellent yields.
5. CYCLOADDITION REACTIONS
5.1. [3+2] CYCLOADDITION REACTIONS
Palladium-catalyzed [3+2] cycloaddition114 reaction is an efficient method to prepare five-membered heterocycles. A number of oxazolidine115 and imidazolidine116 derivatives were synthesized by [3+2] cycloaddition between oxiranes and aziridines with heterocumulenes. An extensive investigation on the palladium-catalyzed [3+2] cycloaddition of 185 with different imines 186 was carried out using Pd2(dba)3 as catalyst and phosphoramidite ligand, bis-2-naphthyl ligand 188, developed by Trost et al.117 The reaction was generally performed at low temperature and both N-aryl and N-Boc imines underwent cycloaddition to afford substituted pyrrolidines 187 (Scheme 62).
A new mechanistically interesting and challenging [3+2] intramolecular cycloaddition route to bicyclic nitrogen heterocycles,118 where internal alkenes as two-carbon components in the cycloaddition, has been developed. Thus, alk-5-enylidenecyclopropanes 189 bearing nitrogen atom when refluxed in dioxane (50 mM) using Pd2(dba)3 (6%) and tris(2,4-di-t-butylphenyl)phosphine (L) (20%) afforded the bicyclic nitrogen heterocycles 190 (Scheme 63).
An unsusal [3+2] cycloaddition reaction was observed during Pd(OAc)2-catalyzed oxidative cyclization of 3-(3'-alkenyl)-N-methyl indole 191 using BQ as oxidant (Scheme 64).119 The reaction afforded an unusual phenolic compound 193 in 27% yield along with the desired N-methyl carbazole 192 in 30% yield. The formation of compound 193 presumably proceeded through the intermediate 194, which underwent formal hetero [3+2] cycloaddition reaction with BQ under the reaction conditions. Isoindoline fused with triazoles was efficiently synthesized by palladium-copper-catalyzed [3+2] cycloaddition reaction.120 Thus o-iodobenzyl azide 195 and terminal alkynes 196 when subjected to Pd(PPh3)2Cl2, CuI and Et3N afforded triazole derivatives 197 (Scheme 65).
An expedient route to 1H-1,2,3-triazole derivatives 200 from sodium azide and alkenyl bromides 198121 has been developed by utilizing Pd2(dba)3 as catalyst and large bite angle ligand x-phos which showed the highest reactivity due to its ability to behave as a trans-chelating ligand. The reaction is assumed to proceed via the intermediate 199 generated by a [3+2] cycloaddition of the azide anion with a vinylpalladium species (Scheme 66).
An interesting palladium-catalyzed decarboxylative ring-opening/ [3+2] cycloaddition reaction has been reported.122 Glycine-derived N-tosyl-5,5-divinyloxazolidin-2-one 201 on treatment with Pd2(dba)3.CHCl3 underwent [3+2] cycloaddition with strongly electron-deficient alkylidenemalonate derivatives to give highly substituted pyrrolidines 202 containing two continuous quaternary centers (Scheme 67).
Synthesis of spiro[isoquinolin-4,5'-isoxazole] compounds 204a-d was achieved by domino intramolecular Heck coupling and 1,3-dipolar cycloaddition.19 The multicomponent one-pot reaction between 2-iodobenzylbromide, allylamines 203 and 3,5-dichloro-2,4,6-trimethylbenzonitrile oxide was accomplished by using Pd(OAc)2 as catalyst to afford the desired spiro compounds 204 (Scheme 68).
5.2. [3+3] CYCLOADDITION REACTIONS
Very few examples of palladium-catalyzed [3+3] cycloaddition have been reported.123 Hayashi et al. in a recent investigation reported palladium-catalyzed [3+3] cycloaddition of 2-(acetoxymethyl)-3-(trimethylsilyl)propene 185a with azomethine imine 205a.124 The reaction was efficiently carried out in the presence of Pd(PPh3)4 to give highly functionalized hexahydropyridine derivatives 206 (Scheme 69).
Similarly, [3+3] cycloaddition reaction of TMM precursors 185a with 207b provided the corresponding cycloadduct 208 (Scheme 70).
5.3. [4+2] CYCLOADDITION (DIELS-ALDER REACTION)
Catalytic [4+2] cycloaddition reaction between a diene and a dienophile have been extensively applied for the construction of six-membered heterocyclic ring.125 1,6-enyne 209a on treatment with Pd2(dba)3.CHCl3 and PPh3 afforded [4+2] cycloaddition adduct 210 (Scheme 71).102 The reaction proceeds via in situ generation of the diene unit by cycloisomerization. However, trapping of the diene unit with other dienophiles like maleinamide and maleic anhydride gave the tricyclic boronate esters 211a-d as single diastereomers. The relative configuration of the three stereocenters is in agreement with the expected endo-approach.
The application of palladium-catalyzed domino Stille coupling and intramolecular [4+2] cycloaddition protocol for the construction of the intermediate oxabicycloadduct 214 was demonstrated during the total synthesis of (±)-lycoridine.126 The oxabicycloadduct 214 was prepared from amidofuran 212 and methyl-2-tri-n-butylstannylacrylate 213 in the presence of Pd(PPh3)4 (Scheme 72). The reaction is suggested to proceed through the expected cross-coupled amidofuran 215 which spontaneously underwent an intramolecular [4+2] cycloaddition to furnish the cycloadduct 214. It is anticipated that the exo orientation of the sidearm of the tethered vinyl group with respect to the oxygen-bridge in the transition state of the cycloaddition step might be responsible for the anti disposition of the carbomethoxy and oxybridge.
5.4. [2+2+1] CYCLOADDITION REACTIONS
Transition metal-catalyzed [2+2+1] cycloaddition, in particular Pausond-Khand reaction, between two olefin units with carbon monoxide have been well investigated and is considered to be a general method for the construction of five-membered ring compounds containing carbonyl group.127 Different allylpropargyl amines when subjected to Pausond-Khand reaction utilizing PdCl2 as catalyst and tetramethylthiourea (tmtu)128 216 as additive in the presence of CO using THF as solvent at 50 oC produced the desired cycloadducts 217 in excellent yields (Scheme 73).129
6. HETEROCYCLIZATION REACTIONS: CYCLIZATION VIA CARBON-HETEROATOM BOND FORMATION
6.1. INTRAMOLECULAR ADDITION OF N-H AND C=N BONDS ACROSS ALKENE, ALLENE AND ALKYNE
The applications of Pd catalysis in the formation of carbon-heteroatom bond via intramolecular addition of heteroatom nucleophiles on to carbon-carbon unsaturated bond are the most attractive and important tools in the transition metal-catalyzed synthesis of heterocycles.1,2
6.1.1. ADDITION TO ALKENE
Alkene appended heteroatom nucleophiles have been found to undergo palladiun(II)-catalyzed heterocyclization to produce a variety of heterocyclic compounds.130 Both activated and unactivated double bonds can react with the nucleophiles. Pd(II)-catalyzed intramolecular amination of N-allyl anthranilamides 218 afforded quinazolin-4-one 219 and 1,4-benzodiazepin-5-one 220 in high yields depending upon the reaction conditions employed (Scheme 74).131 The six-membered quinazoline 219 was obtained when the reaction was performed with Pd(OAc)2 and NaOAc in polar solvent DMSO at 100 oC for 24 h. The reaction is suggested to proceed via the initial formation of η3-allyl-Pd complex 221 and η2-allyl-Pd-complex 222.132 On the other hand, the synthesis of seven-membered benzazepine ring system 220 was achieved by using pyridine as a base in non-polar solvent xylene (Scheme 74).
The aerobic intramolecular oxidative amination of olefinic tosyl amines 225a-d using N-heterocyclic carbene133 (NHC)-coordinated Pd(II) complex (IMes)Pd(CF3CO2)2 as catalyst proceeded smoothly with air as well as pure oxygen gas, as the source of oxidant if carboxylic acid like PhCO2H or AcOH was employed as co-catalyst in the reaction to afford the indole derivatives 226a-d (Scheme 75).134
An efficient route to the synthesis of 3,5-disubstituted piperazinones 228 from the corresponding starting material 227 was reported.135 An extensive study on the diastereoselectivity of the intramolecular amidation reaction during the synthesis of 3,5-disubstutited piperazinones 228 has been reported.136 The cyclizations were carried out under three different conditions (Scheme 76). It is surprising that additive (LiCl) played an important role on the cis:trans ratios of products. The cis:trans of the products of Pd(0) catalysis in the presence of LiCl was always higher than those observed using Pd(0) alone. The favoured isomer was always the cis as observed. Interestingly, a complete reversal of the diastereoselectivity where cis: trans ratio spanning 21:79 to 2:98 in favour of the trans isomer was observed in the absence of LiCl.
The intramolecular amination of cis-allylic alcohol 229 and ester 231 with Pd(PhCN)2Cl2 in THF at room temperature afforded cis-1,3-disubstituted 1,2,3,4-tetrahydroisoquinoline 230 and cis-2-carbomethoxy-5-vinylpyrrolidine 232 respectively (Scheme 77).137 A chair-like trasition state model 233 where axial orientation of allylic alcohol due to the complexation of Pd(II) might be responsible for this cis-stereoselectivity in tetrahydroisoquinoline 230. The asymmetric intramolecular allylic amination was also achieved utilizing Pd2(dba)3.CHCl3 in the presence of chiral P,N ligands-(234), 2-phosphinophenyl)pyridine.138
Synthesis of optically active vicinal diamines via palladium-catalyzed C-O to C-N bond transformation involving the intramolecular nucleophilic attack of nitrogen functionality tethered to the C-4 amino group on the C-3 position was achieved.139 Based on this strategy trans- and cis-imidazolidin-2-ones (236a and 236b) were synthesized from compound 235 (Scheme 78).
Trost and collaborators demonstrated a highly efficient and atom economic dual catalytic approach comprised of Ru-catalyzed intermolecular enyne cross-coupling140 of 237 and 238 followed by intramolecular nucleophilic trapping of π-alkylpalladium species, in a one-pot reaction sequence for the synthesis of enantio- and diastereo pure N-heterocycles.141 The enyne coupling was carried out by sequential addition of RuCp(MeCN)3PF6 in acetone and [Pd(η3-C3H5)Cl]2/RR-(239) catalytic system followed by intramolecular nucleophile trapping process without isolating the 1,4-diene intermediate. Both pyrrolidines and piperidines are formed with good yield (Scheme 79).
The chemoselectivity and diastereoselectivity of PdCl2/CuCl2-catalyzed chlorocyclization of aminoalkenitol 240 exhibited a significant dependence on the nature of the solvent used (Scheme 80).142
Formation of unexpected bicyclic derivative 241 with a lower combined yield of desired C-6 substituted azasugars L-altro 242 and D-galacto 243 was observed when the reaction is conducted in AcOH. A reversal of diastereoselectivity in favour of D-galacto 243 was found in PhMe or methanol albeit in poor des (33% and 50%). DMF as a solvent performed comparatively better (88% de) and it is a solvent of choice for the preparation of L-altro 242. It is proposed that the initial bis-coordination of PdCl2 with both (C-3) OBn group and C=C moiety of 240 followed by intramolecular Re-attack of N-nucleophile promotes the formation of a σ-Pd-complex-I in a geometrically favorable C4 chair conformation. Subsequent formation of heterobimetallic143 σ-Pd/Cu-complex-249 followed by reductive elimination generates the bicycle 241, on the other hand nucleophilic addition to Pd(II)-promoted activated double bond from its Si-face leading to a σ-Pd-complex-250, subsequent formation of σ-Pd/Cu complex-250 and final reductive elimination furnishes the desired chlorocyclization product 242 (Schemes 81 and 82).
Similarly, chlorocyclization of sugar-derived aminoalkenitol 240b when subjected to PdCl2/CuCl2 catalyst and NaOAc as base in AcOH afforded L-ido configured C-6 chlorinated azasugar 251 as the major product along with its minor D-gluco diasteromer 252 with a good combined yield (70%) and high diastereoselectivity (90% de). The reaction in other solvents like DMF, DCM, THF, MeOH and PhMe were less efficient as poor yield and diastereoselectivity were obtained (Scheme 83).
However, for the palladium-catalyzed domino N-arylation/carboamination144 of γ-amino alkenes 253 with two different aryl bromides, an in situ ligand exchange protocol was found to be beneficial as the reaction proceeded through the formation of two C-N bonds and one C-C bond in one-pot.145 By utilizing Pd2(dba)3 as catalyst, the first arylation reaction was achieved with monodentate ligand 2-di-tert-butylphosphinobiphenyl (L) which underwent in situ ligand exchange with the chelating ligand dppe to effect the carboamination-arylation step and providing the cis-substituted pyrrolidine 254 (Scheme 84).
Another application of palladium-catalyzed carboamination reaction was achieved during the efficient synthesis of (±)-preussin 257a and (±)-3-epi-preussin 257b,146 a potent antifungal and antitumer agent. The TBS-protected anti- and syn-aminoalcohols 255a and 255b on treatment with Pd(OAc)2 and dpephos afforded the corresponding pyrrolidine derivatives 256a and 256b in 62 and 54% yields respectively (Scheme 85)147 from which (±)-preussin and (±)-3-epi-preussin were subsequently synthesized in few steps.
Wolfe et al. utilized the palladium-catalyzed cascade C-N and C-C bond formation strategy for the preparation of substituted imidazolidin-2-ones 260.148 The acyclic ureas 258 when subjected to intramolecular carbamination reaction in the presence of different aryl and heteroaryl bromides using Pd2(dba)3 as catalyst, x-phos as cocatalyst afforded the imidazilidin-2-one derivatives 260 (Scheme 86).
Palladium-catalyzed enantioselective C-3 allylation of 3-substituted-1H-indoles using trialkylboranes was developed by Trost et al.149 The selectivity is highly dependent on the borane reagent used in the reaction as in addition to promoting the ionization of allyl alcohol150 (Scheme 87).
ω-Olefinic-N-tosyl amides 264a,b and vinylic bromide underwent domino Heck-allylic substitution151,152 to furnish substituted pyrrolidone 265a (n = 1) and piperidone 265b (n = 2). The reactions were best carried out utilizing Pd(OAc)2, P(o-tol)3, Na2CO3 and n-Bu4NCl in MeCN at 90 oC to afford compounds 265a and 265b (Scheme 88).153
The aziridination of olefin was carried out in a palladium-catalyzed reaction between various alkenes and N,N-dichloro-p-toluene sulfonamide 266 (TsNCl2) as a nitrogen source.154 The reaction was carried out at room temperature using Pd(OAc)2 with an alkene/TsNCl2 ratio 1.5:1 (mol/mol) to afford the aziridines 268 (Scheme 138). The chemical yields under this condition are higher than those using bromamine-T as a nitrogen source and palladium dichloride as catalyst155 (Scheme 89).
Hartwig et al. synthesized tropene derivatives by sequential intermolecular and intramolecular transannular palladium catalyzed hydroamination of cycloheptatrienes.156 The combination of x-phos, Pd(CF3CO2)2 and PhCO2H showed highest efficiency in this transformation. The product 270 was isolated along with the dihydroquinoline derivative 271 (Scheme 90).
One-pot double oxidative amination of 272a,b having orthogonally positioned amide and aniline with Pd(OAc)2, charcoal and NaOAc in DMSO and O2 afforded the tetracyclic compounds 274a,b having three stereogenic centers (Scheme 91).157 The compound 272a when subjected to dieneoxidation-1,4-cyclization with Pd/C, DBU and t-BuO2H in reflxing CH2Cl2 afforded the analogue of Büchi ketone 273.
Sequential catalytic transfer of two sulfonamides to internal alkenes has been developed as an effective route to construct bisindoline.158 This domino oxidative diamination of 275 was accomplished utilizing Pd(OAc)2 and NaOAc to give bisindoline derivatives 276 in excellent yields (Scheme 92). The characteristic feature of this reaction is the flexibility of palladium catalyst in the sequential formation of Csp2-N and secondary Csp3-N bonds,159 employing a nitrogen source of the same electronic nature. Mechanistically the reaction proceeds through the palladium-tosyl amide precoordination 277 followed by endoselective anti-aminopalladation to convert into a chelation-controlled state 278. The complex-278 may undergo oxidation to palladium(IV) 279,160 amide dissociation followed by selective anti-amination/depalladation161 to afford bisindoles 276.
6.1.2. ADDITION TO ALLENE
The transition metal-catalyzed cyclization of functionalized allenes is important due to its unique reactivity and stereoselectivity.162 Palladium-catalyzed addition of heteroatom nucleophiles to allenes has found extensive applications in the synthesis of heterocycles.163
Grigg et al. developed a novel palladium-catalyzed cyclization-anion-capture cascade process involving in situ zipper generation via intramolecular nucleophilic attack on the π-allylpalladium species and their subsequent cyclization-anion-capture using boronic acid as anion capture reagent to access tricyclic heterocycles 282.164 The trifunctional aryl iodide/allene/nucleophile substrates 280a,b were treated with 2-iodothiophene, Pd(PPh3)4 and Cs2CO3 in PhMe at 70 oC for 16 h then phenyl boronic acid was added and the mixture was heated at 100 oC for further 22 h to afford the tricyclic compounds 282a,b as single diastereomers. The interesting feature is that three C-C bond, one C-N bond, two rings, two stereomers, and one tetrasubstituted C-center were formed in this one-pot cascade reaction sequence (Scheme 93).
In the case of compound 280c, a slightly modified condition was needed to nullify the faster rate of the oxidative addition onto C-Br bond by the more effective coordination of the oxygen atom of the amide due to the difference in electronegativity of the N-atom in position-1 compared to 4. To overcome this difficulty Lyrigg165 performed the reaction with excess of triphenylphosphine to give the desired cyclized product 282c as a single diastereomer.
6.1.3. ADDITION TO ALKYNE
Rutjes et al. reported an efficient synthesis of unsaturated proline and optically active tryptophan analogues via palladium-catalyzed intramolecular cyclization of aniline containing acetylenic amino acid.166 The type of product formation depends on the side chain length of the cyclization precursors. Thus, the compound 283 when treated with Pd(MeCN)2Cl2 afforded the imine 284 (Scheme 94). Here the catalyst Pd(MeCN)2Cl2 acts both as a cyclizing agent as well as a Lewis acid which is evidenced by the formation of cyclic amide 283a at ambient temperature. Treatment of this enamide with the same Pd catalyst in refluxing acetonitrile led to rapid conversion into the aforementioned imine 284.
In a recent report it has been observed that 2-(2-aminophenyl)-4-trimethylsilanylbut-3-yn-2-ol 285a and 1-(2-aminophenyl)-1-phenyl-3-trimethylsilanylprop-2-yn-1-ol 285b under the same combination of catalyst in MeOH underwent 6-endo-dig cyclization-dehydration to afford 4-methylquinoline 286a and 4-phenylquinoline 286b (Scheme 95).167
The asymmetric version of the intramolecular catalytic hydroamination and hydroxylation of alkynes using chiral palladium catalyst in situ generated from Pd2(dba)3.CHCl3 and (R,R)-renorphos 289 was recently developed by Yamamoto et al.168 The hydroamination reaction of 287 was examined under two different reaction conditions and condition-N was observed to be efficient (Scheme 96).
The amidoalkynes 290 underwent intramolecular hydroamination reaction when subjected to Pd(PPh3)4 in PhCO2H at 100 oC.169 The six-membered lactams 291 were obtained with E-geometry of the double bond. Tosylamide was formed to give excellent result during this cyclization. The reason behind the cyclization over β-hydride elimination might be due to the exchange of ligand at the palladium atom by π-Pd complex generated from 290 (Scheme 97), which inhibit the β-hydride elimination as well as diminish the basicity of the nitrogen atom of the resulting π-Pd complex due to the presence of two electron-withdrawing groups.
The first example of intramolecular addition of P-NH to substituted alkynes was achieved by the synthesis of novel six-membered phosphorous heterocycles, phosphaisoquinoline-1-ones, 293 with potential bioactivities using the intramolecular regioselective cyclization of o-(1-alkynyl)-phenylphosphonamides 292 in presence of Pd(MeCN)2Cl2 (Scheme 98).170
Cacchi et al. showed that ethyl 3-(o-trifluoroacetamidophenyl)-1-propargyl carbonate 294 reacted with p-iodoanisole under the hydroarylation condition Pd(OAc)2, PPh3, and piperidine to afford 2-methyl indole 296 along with 2-(piperidin-1-ylmethyl)indole 297.171 However, in the presence of N-substituted piperazine and Pd(PPh3)4, compound 294 afforded 2-(piperazin-1-ylmethyl)indoles 299. The mechanistic rationalization for the formation of indole is believed to be initiated with the formation of σ-allenylpalladium complex which may be in equilibrium with π-propargylpalladium intermediate 300.172 Subsequent intramolecular nucleophilic attack of the nitrogen at the central carbon of the allenyl/propargyl-palladium complex173 followed by protonation of the resultant carbene 301 may give the σ-allenylpalladium complex 302. Finally regioselective intermolecular nucleophilic attack of the nitrogen nucleophile at the less hindered allylic terminus of 302 may afford the desired indole derivatives (Scheme 99).
An aminopalladation-reductive elimination approach174 was adopted for the synthesis of 2,3-disubstituted pyrrolo[2,3-b]quinoxalines 304. The reaction of 2-alkynyl-3-trifluoroacetamido quinoxalines 303 with aryl and vinyl halides or triflates in the presence of Pd(PPh3)4 afforded the compounds 304 (Scheme 100).
α-Amino(2-alkynylphenyl)methylphosphonate 305 underwent endo-cyclization when subjected to Pd(PhCN)2Cl2 in acetonitrile to afford 2,3-disubstituted-2H-isoindol-1-ylphosphonates 306 in moderate-to-good yields (Scheme 101).175
The domino aminopalladation of 2-alkynylaniline 307 and subsequent conjugate addition to α,β-unsaturated carbonyl compound in the presence of Pd(OAc)2 and LiBr afforded the indole derivatives 308 (Scheme 102).176 The formation of indoles 308 may be rationalized by a domino intramolecular aminopalladation, olefin insertion and protonolysis of carbon-palladium bond. No β-hydride elimination product was formed giving preferentially the protonolysis product in acidic medium.
The synthesis of 4-(1-alkenyl)-3-arylisoquinolines 310 was achieved by the Pd(II)-catalyzed cyclization of 2-(1-alkynyl)arylaldimines 309 in the presence of methyl acrylate (Scheme 103).177 The reaction involved a sequence of Pd(II)-catalyzed intramolecular cyclization of imine onto C-C triple bond followed by intermolecular Heck olefination reaction.
The ortho-alkynylarylimine 309 underwent domino bis allylation178,179 with allyltributylstannane and allyl chloride in the presence of a catalytic amount of π-allylpalladium chloride dimer and Cu(OAc)2 to afford 1,4-diallyl-1,2-dihydroisoquinolines 313.180 The chemical yields of the reaction is highly dependent on the substituent R2 (Scheme 104).
The reaction proceeds with the generation of the amphiphilic bis-π-allylpalladium complex181 314a by oxidative addition of Pd(0) to allyl chloride followed by transmetallation with allyltributylstannanes. The complex 314a reacts with 309 to generate π-allylpalladium amides 315 which are subsequently converted to Pd-alkyne complexes 316 by Cu(OAc)2-assisted cleavage of the Pd-N interaction. Finally, attack of the nitrogen atom to the alkyne in a 6-endo-dig mode followed by reductive coupling affords the products 313 (Scheme 105).
A general regioselective synthesis of various substituted pyrrole derivatives was reported by Gabriele et al.182 The cycloisomerization reaction was carried out in two ways by employing two different catalytic systems such as Pd(II)-catalyzed and CuCl2-catalyzed cycloisomerization reactions. It is interesting when the triple bond is substituted with an alkyl or alkenyl group. Substrates 317, underwent cycloisomerization reaction smoothly with Pd(II) to afford the pyrrole derivatives 318 (Scheme 106). The pyrrole-2-acetic ester derivatives were also synthesized183 by the application of the oxidative carbonylation of 3-substituted enynamines.
6.2. INTRA- AND INTERMOLECULAR COUPLING OF NH WITH VINYL AND ARYL HALIDE OR TRIFLATE
Pd-catalyzed cross-coupling between Csp2-halides or triflates represent some of the most powerful and versatile tools for the construction of C-N and C-O bonds.1 Methodologies for the construction of this type of bonds intramolecularly have become extraordinarily popular, as they represent a very efficient entry into different types of important nitrogenated and oxygenated heterocyclic compounds.2
6.2.1. ARYL HALIDES OR TRIFLATES
Both intra- and intermolecular version of the palladium-catalyzed cross coupling reaction between aryl halides and amines184 have been applied to the synthesis of heterocycles.185 An efficient regioselective method for the synthesis of structurally diverse imidazopyridones 320 inexcellent yields by Pd-catalyzed reductive cyclization186 of N-substituted o-chloroaminopyridines 319 has been reported (Scheme 107).
A facile route based on the Pd(0)-catalyzed N-arylation approach for the construction of phenazine 322 has been reported by the cyclization of bromodiphenyl amine 321 using Pd2(dba)3 and ligand-323 (Scheme 108).187
The intermolecular cross coupling reaction between 2-bromobenzaldehyde with aryl hydrazine was achieved188 in the presence of Pd(dba)2, dpe-phos and K3PO4. The catalyzed reaction is suggested to proceed via the five-membered palladacycle 328b possibly in equilibrium with open structure 328a (Scheme 109). The cyclization involves a different six-membered palladacycle, which can be formed from 328 by deprotonation of NH by the base and subsequent metallotropic shift of Pd to form palladacycle 329. Finally dissociation of Pd-N bond produces the indazole compound 327.
A versatile route to mono-N- and di-N-alkylated quinazolinediones 332 via palladium-catalyzed urea arylation-intramolecular ester amidation protocol was achieved189 in the presence of Pd2(dba)3, x-phos and Cs2CO3 (Scheme 110). Between the o-chloro and o-bromo benzoate, the reaction of the latter one effectively coupled with the urea component 331 and gave both mono-N- and di-N-substituted compounds 332.
A new route to oxacarbazepine (trileptal) in which palladium-mediated intramolecular N-arylation of the amine 333 was studied.190 Under the optimized reaction conditions amine 333 afforded the dibenzoazepinone 334, thus minimizing the amount (<4%) of the dehalogenated byproduct. Moreover, water as a convenient additive or co-solvent produced a beneficial effect on the process in two ways: a) accelerating the rate of the reaction, b) better solvent for K3PO4 promoting the target amination (Scheme 111).
In case of heteroaromatic substrates 335, the aforementioned procedure worked well for the thiophene substrates giving the tricyclic compounds 336.191 In contrast, for the heteroaromatic substrates like the pyridine derivatives 335e,f failed to give the azepinones (Scheme 112).
An efficient synthetic protocol for the synthesis of a wide range of N-arylated 5-, 6- and 7-membered heterocycles 339a-c involving palladium-mediated sequential intramolecular and intermolecular arylamination reactions has been reported.192 The use of an in situ generated Pd(0) catalyst associated to N-heterocyclic carbene, N,N-bis(2,6-diisopropylphenyl)dihydroimidazol-2-ylidine (SIPr) as ligand, NaOt-Bu and Pd(OAc)2 was found to be effective and various indolines (n = 1), tetrahydroquinolines (n = 2), 2,3,4,5-tetrahydro-1H-1-benzazepines (n = 3) 339c were synthesized by the reactions between compound 337 with aryl chlorides (Scheme 113).
Pd(dba)2-catalyzed intramolecular Buchwald-Hartwig amination of the amide 340 has been found to be highly dependent on the nature of the added ligand and the solvent. Pd(OAc)2 was found to be inefficient and gave poor yield of the desired oxindole.193 However, oxindole 341 was obtained in 82% yield when the amination was carried out under microwave condition (Scheme 114).
A novel synthetic strategy for the synthesis of medium ring heterocycles dihydro-8H-5,7a-diazacyclohepta[jk]phenanthrene-4,7-dione 343 and 1,4-benzodiazepine-2,5-dione 344 from the same starting material 342, was achieved194 simply by switching the metal catalyst. Interestingly, Pd(OAc)2 triggered a domino intramolecular N-arylation/C-H activation/aryl-aryl bond forming process to provide the compound 343, while copper iodide promoted only the intramolecular N-arylation reaction leading to the formation of the compound 344 (Scheme 115). Compound 344 was also converted to the compound 343 simply by a Pd-catalyzed biaryl coupling.
A divergent regiocontrolled palladium-catalyzed domino sequence involving an intramolecular N-arylation and an intramolecular cyclization strategy for the synthesis of highly functionalized benzodiazepindiones 346 from the precursors 342a has been reported195 (Scheme 116).
Amide-NH2 was employed for the palladium-catalyzed aryl C-N cross-coupling with aryltriflates 347 bearing alkyl chain with a leaving group for the construction of indole derivatives.196 An optimization study of this domino sp2-sp3 amidation reaction revealed that the cyclization is highly dependent on the leaving group (X) of the compound 347. The formation of seven-membered heterocycle 350a was achieved when mesylate was used as leaving group. With acetate and carbonate along with the indole 349 uncyclized monoaminated products 350b and 350c were obtained. However, 2-triflyloxy phenethyl carbonates 347g afforded 2,3-dihydroindole 349 in 98% yield when 5 mol% of Pd2(dba)3 and 10 mol% x-phos as ligand were used (Scheme 117).
A combination of microwave techniques and traceless polymer-supported strategies was utilized for the synthesis of benzimidazoles libraries with two point of diversity using ameba resin as a traceless linker.197 The key intramolecular cyclization of resin bound urea was carried out smoothly, catalyzed by Pd(OAc)2 under microwave irradiation for 15 min. The compounds 352 were converted into polymer free benzimidazolones 353 (Scheme 118).
A new route to benzimidazo[1,2-a]quinolines 355 via Pd(PPh3)4-catalyzed intramolecular heterocyclization of readily accessible 2-(2-bromoanilino)quinolines 354 was reported.198 The overall process involved Buchwald-Hartwig amination in which the heteroarene ring nitrogen participate in the N-C bond formation (Scheme 119).
Nozaki et al. reported double N-arylation strategy of primary amines with 2,2΄-biphenylene ditriflate for the synthesis of multisubstituted carbazoles 357.199 Among the different ligands (358a-c, 359, 360) employed 358b and 360 were found to give better results. However ligand 358b is the ligand of choice as ligand 360 in the presence of Cs2CO3 or NaOt-Bu instead of K3PO4 afforded the carbazole in 50% or 14% yield respectively along with byproduct biphenyldiol (22% or 50%). The hydrolysis of triflate was also reported to occur when nucleophilic strong base was employed (Scheme 120).
Synthesis of ladder-type pyrrole rings, indolo[3,2-b]carbazoles 362a-c was achieved200 by Pd(OAc)2-catalyzed double N-arylation of 361a-c using 2-di-tert-butylphosphino-2'-methylbiphenyl (L1) as ligand and 2,6-di-tert-butyl-4-methylphenol (BHT) as additive. The diamination reaction with aniline for the formation of compounds 362a-c was influenced by the ligand employed (Scheme 121).
6.2.2. VINYL HALIDES OR TRIFLATES
The cross coupling reaction between vinyl halides or triflates with amine or alcohol are less. This protocol was applied for the synthesis of 1-aryl-1H-pyrazoles 364 from cyclic and acyclic β-bromovinyl aldehydes 363 and arylhydrazines201 by using Pd(OAc)2, dppf and NaOtBu (Scheme 122).
Ortho-gem-dihalovinylaniline 365 underwent domino intramolecular Buchwald-Hartwig amination and intermolecular Suzuki-Miyaura coupling when subjected to aryl boronic acid in the presence of Pd(OAc)2, as catalyst and Buchwald’s S-phos ligand. The methodology was examined with a broad variety of boronic acid to give 2-phenyl indoles 366a-i (Scheme 123)202 with an attractively low catalyst loading (typically 1-3 mol% Pd).
Domino Buchwald-Hartwig amination / Heck reaction was utilized for the synthesis of 2-vinylic indoles 368 from gem-dibromovinylanilines 367.203 The reaction was examined under three different catalytic combinations depending upon the nature of the N-substituents. The coupling with both electron deficient and electron rich N-aryl substrates proceeded smoothly to afford the vinyl indole (Scheme 124).
6.3. CYCLOCARBONYLATION REACTIONS
The palladium-mediated carbonylation204 and carboxylation205 reactions have been extensively employed in the synthesis of heterocycles. Ma et al. reported a mild and efficient Pd-catalyzed carbonylation of propargylic alcohols with various structural patterns for the synthesis of (Z)-R-chloroalkylidene-β-lactones selectively.206 The PdCl2/CuCl2-catalytic system with BQ oxidant and carbon monoxide was highly efficient for the cyclocarbonylation of propargylic amines 369 leading to the synthesis of (E)-α-chloroalkylidene-β-lactams 370.207 No Z-isomer or five-membered product was obtained (Scheme 125).
In recent report, 2-iodoaniline has been found to behave as a bifunctional substrate during carbonylation with carbon monoxide.208 When subjected to Pd(OAc)2/PPh3 catalyst system in the presence of carbon monoxide, the anilines 371a,b afforded 2-aryl-benzo[d][1,3]oxazin-4-one derivatives 372a,b via double carbon monoxide insertion keeping one NH2 intact where as symmetric 5H,11H-dibenzo[b,f][1,5]diazocine-6,12-diones (dianthranilides) 373c-f were obtained as the major products from the substrates 371c-f. The reaction is very much chemoselective (>96%) (Scheme 126).
6.4. REDUCTIVE N-HETEROCYCLIZATION OF NITROARENE
Palladium catalyzed reductive N-heterocyclization of nitroarenes is emerging as a versatile method for the preparation of five-membered nitrogenated heterocycles.209 The carbon monoxide behaves as a reducing agent in the presence of palladium.210 Ragaini et al. carried out a detailed investigation on the scope and efficiency of intermolecular cyclization of unfunctionalized nitroarenes and alkynes catalyzed by palladium-phenanthroline complexes leading to the synthesis of 3-arylindoles 375.211 The indole synthesis was achieved in the presence of [Pd(Phen)2][BF4]2 as catalyst and carbon monoxide in DMF at 170 oC with excellent selectivity (Scheme 127).
A plausible reaction mechanism for the indole synthesis may involve the formation of nitroarene intermediate 376 which interacts with the alkyne reversibly to give an intermediate 380 having two possible resonating structures, α-styryl cation212 and α-styryl radical.213 Final cyclization would give the hydroxyindole 379 which in the presence of CO and the catalyst is reduced to indole 375 (Scheme 128).
Another interesting example of N-heterocyclization reaction was observed during the synthesis of 3-alkoxy indole derivatives.214 Relatively lower temperature was required for efficient conversion and in the presence of Pd(dba)2/phenanthroline/dppp catalytic system 1-(2-nitrophenyl)-1-alkoxyalkenes 381a afforded the indole derivatives. The product 383 was similarly prepared (Scheme 129).
An efficient synthesis of naturally occurring carbazole alkaloid murrayquinone-A 388 has been reported.215 The carbazolone intermediate 387 was prepared from its nitroarene precursor 386 by Pd(dba)2/dppe/1,10-phenanthroline-catalyzed reductive N-heterocyclization in excellent yield. Murrayquinone-A 388 was obtained in few steps from 387 (Scheme 130).
A range of tetrahydrocarbazolone derivatives 390 was also synthesized as advanced intermediates to carbazole alkaloids utilizing the palladium catalyzed reductive N-heterocyclization approach216 (Scheme 131).
6.5. AMINO HECK REACTION
This reaction has been exploited in the synthesis of various types of pyrrole, isoquinoline and pyridine derivatives.217 The synthesis of trisubstituted imidazoles with a range of substituents at the 2-position was achieved by amino Heck reaction.218 This intramolecular C-N bond formation using N,O-pentafluorobenzoyl amidoximes 391 as precursors was carried out in the presence of 10 mol% of Pd(PPh3)4 and 5 equiv of triethylamine in DMF at 80 0C (Scheme 132).
This amino-Heck based methodology was also extended to the synthesis of optically active amino acid mimetics with C-terminal imidazole in good yields. The transformation of S-Phe and S-Leu based amidoximes 393a and 393b to the cyclized products 394a and 394b underwent smoothly in 1 h where as S-ala based cyclization product 394b was isolated within 30 min. (Scheme 133).
7. CYCLIZATION VIA CASCADE CARBON-CARBON/CARBON-HETEROATOM BOND FORMATION: HETEROCYCLIZATION REACTIONS
7.1 HETEROCYCLIZATION REATIONS WITH ALKYNES
Palladium-catalyzed intermolecular heterocyclization of alkynes has emerged as an accomplished route to the synthesis of large varieties of heterocycles.219 The annulation occurred via two different mechanistic pathways with the nature of alkyne used.220
7.1.1. REACTIONS WITH TERMINAL ALKYNES: SONOGASHIRA COUPLING-CYCLIZATION REACTIONS
Hopkins et al. reported the synthesis of 6-phenyl-5H-pyrrolo[2,3-b]pyrazines 397 by the reaction between alkynes and N-(3-chloropyrazin-2-yl)methanesulfonamide 396 under different catalytic conditions221 (Scheme 134).
The reaction also proceeded in the presence of Pd(dppf)Cl2-CuI, however, the yield was comparatively low. One-pot Sonogashira coupling-base induced cyclization was applied to the synthesis of 3-arylmethyleneisoindoline and pyrrolopyridinones 399a,b starting from their respective amides 398a and 398b respectively.35 The (Z)-selectivity may be due to an anti-addition across the carbon-carbon triple bond (Scheme 135).
An expedient synthesis of biologically active azaindole and indole comprises of Sonogashira coupling and base promoted cyclization starting from less commonly used 2-chloro-3-aminopyridines 400.222 2-Chloro-3-aminopyridines 400 were successfully converted to azaindoles 401 in excellent yields when subjected to Pd(OAc)2-dppb catalytic system (Scheme 136, condition A). Alternatively Pd(MeCN)2Cl2-x-phos catalytic system (Scheme 136, condition B) was found to have the same catalytic efficiency for the conversion.
Lu et al. reported a Pd-catalyzed practical one-pot three-component process for the preparation of 2,3-disubstituted indoles 403. Sonogashira coupling and aminopalladation were carried out under the same reaction conditions (Scheme 137).223 An examination of the base, solvent, temperature and ligand effect on the reaction revealed that a combination of 5 mol% of Pd(OAc)2, 20 mol% of PPh3 and K2CO3 in DMF at 60 oC was effective enough for this one-pot process to give the 2,3-disubstituted indole derivatives 403 in excellent yields.
A ligand-, copper- and amine-free synthesis of 2-substituted indole 366a in good yield via Sonogashira coupling-5-endo-dig cyclization has been reported.224 The reaction between N-tosyl 2-iodoaniline and phenyl acetylene was conducted under both ultrasonic irradiation and standard stirred condition utilizing Pd(OAc)2. A significant enhancement of rate was observed under ultrasonic irradiation (Scheme 138).
7.1.2. REACTIONS WITH INTERNAL ALKYNES
The heterocyclization reaction between N-(3-chloropyrazin-2-yl)-methanesulfonamide 404 and internal alkynes in the presence of Pd(dppf)Cl2 as catalyst and LiCl as additive under microwave irradiation furnished 6-substituted-5H-pyrrolo[2,3-b]pyrazines 406 (Scheme 139).225
A palladium-catalyzed synthetic strategy for the regioselective synthesis of highly substituted pyrroles 409 was developed226 by coupling-cyclization between stabilized iodoenamines and symmetrical alkynes utilizing Pd(OAc)2 as catalyst to afford the pyrroles in good yields. With unsymmetrical alkynes two methods were attempted to accomplish the reaction which showed poor regioselectivity affording two regioisomeric pyrroles in the ratio spanned from 1.5:1 to >25:1 (Scheme 140).
A one-pot synthesis of 2-aryl- and 2-vinyl indoles based on a ruthenium-catalyzed hydroxyamination227 and a palladium-catalyzed intramolecualr Heck reaction using 2-chloroaniline 410 as precursor352, 228 has recently been reported (Scheme 141).
7.2 HETEROCYCLIZATION REACTIONS WITH ALKENES
The allyl tosylamides were found to undergo intermolecular oxidative coupling reaction with t-butyl vinyl ether in the presence of Pd(OAc)2 as catalyst and Cu(OAc)2 as additive.229 Catechol and MS (3Å) were found to be beneficial co-catalyst in this reaction and in the presence of molecular oxygen as oxidant pyrrolidine derivatives 416 were synthesized (Scheme 142).
In a recent development, Pd(II)/pyridine catalyst system has been employed for the tandem oxidative cyclization of 417 to afford indoline derivatives230 418. The reaction was successfully accomplished utilizing Pd(OAc)2 as catalyst and molecular oxygen as sole oxidant (Scheme 143).
The total synthesis of enantiopure (+)-γ-lycorane was achieved231 from the intermediate pentacyclic oxylycorane 420 which was in turn synthesized through Pd(OAc)2-dppb catalyzed one-pot domino allylic amination-intramolecular Heck reaction (Scheme 144).
A domino-heterocyclization reaction between o-iodoaniline and 2-N,N-di-t-butoxycarbonylamino-5-oxopentanoate 423 was adopted for the synthesis of ring-A substituted tryptophan derivatives 424232 (Scheme 145).
7.3. HETEROCYCLIZATION REACTIONS WITH DIENES
The heterocyclization of N-carbobenzyloxy-o-iodoanilines 425 with 1-phenylthio-1,3-butadiene 426 was carried out in aqueous MeCN containing K2CO3 in the presence of a catalytic amount of Pd(OAc)2233 to afford vinylogous 2-(phenylthio)indole derivatives 427a-c in 49-75% yields (Scheme 146).
The regio- and stereoselective annulation234 of tributylstannylallenes 429 with (Z)-3-substituted-3-iodoprop-2-enamides 428 catalyzed by Pd(OAc)2 afforded 4,6-disubstituted-2-pyridone 430 in 85% yield. This annulation probably occurred via Stille reaction followed by intramolecular cyclization (Scheme 147).
7.4. HETEROCYCLIZATION REACTIONS WITH CARBON-HETEROATOM UNSATURATED BOND
The participation of the aza-allylic anions generated from imines in sequential Pd-catalyzed C-C and C-N bond-forming reactions has lead to the development of a new and efficient method for the synthesis of indole derivatives.235 Aza-allylic anions have been extensively employed as three atom synthons in Classical heterocyclic chemistry.236 The two component cascade sequence involving C- and N-arylations between o-dihalobenzenes and imine 431 was carried out in the presence of [Pd2(dba)3] as catalyst, x-phos as ligand and sodium tertiary butoxide as base in to give indoles 432 (Scheme 148).
The reaction of 2-bromobenzylamine 433a and 2-(2-bromophenyl)ethanamine 433b with tert-butylisonitrile in the presence of PdCl2, dppf and Cs2CO3 gave cyclic amidines 434a,b237 (Scheme 149).
7.5. HETEROCYCLIZATION REACTIONS WITH ARENES
Direct synthesis of carbazole by palladium-catalyzed domino reaction has been reported.238 The reaction involves an amination and direct arylation using readily available N-phenyl anilines and 1,2-halo(hetero)arenes 436 in the presence of Pd(OAc)2 as catalyst, PCy3 as ligand and sodium tertiary butoxide as base. PPh3 and N,N’-bis(diisopropylphenyl)imidazolium chloride gave good yields of carbazole derivatives 437 (Scheme 150).
A one-pot synthesis of carbazole derivative was achieved by palladium-catalyzed intermolecular coupling between o-dibromobenzene and 5-methylanthranillic acid.239 It is interesting to note that instead of second nucleophilic attack leading to the formation of o-diarylaminobenzene, the intramolecular reductive elimination occurred affording the carbazole derivative 439 (Scheme 151).
An efficient methodology for the construction of N-H carbazoles from 2-chloroanilines has recently been reported.240 The microwave assisted intermolecular reaction between 2-chloroaniline with different arylbromides using Pd(OAc)2 as catalyst and P(t-Bu)3 as ligand afforded the carbazole derivatives 442a-d. However, the reaction between 2-chloroaniline and 2-bromobiphenyl yielded an inseparable mixture of 442e and 442f. The domino reaction may proceed via Buchwald-Hartwig amination followed by intramolecular ring closure by C-H activation (Scheme 152).
A simple catalytic synthesis of condensed pyridones involving ipso substitution via palladacycles was reported by Catallani et al.241 Symmetrically condensed pyridones 446 were obtained starting from o-bromocarboxamides 445 in the presence of norbornene, Pd(OAc)2/PPh3 as the catalyst, K2CO3 as base in DMF (Scheme 153).
Total synthesis of hippadine 449 by a one-pot domino metallation-cross coupling-lactonization was achieved from 7-bromo indole 447 and 6-halogenated piperonoate 448 (Scheme 154).242
Suzuki reaction of 6-bromo indole afforded the hippadine derivatives in 21-74% yields where as comparatively lower yield of hippadine was obtained under Suzuki and Stille condition from electron-deficient methyl-6-iodo- and 6-bromopiperonoate.
A simple synthesis of bioactive 6-aminophenanthridines by Suzuki-Miyaura coupling reaction has recently been described.243 The condensation between 2-(4,4,5,5-tetramethyl-1,3-dioxaborolan-2-yl)aniline 450 and 2-chlorobenzonitrile 451 in the presence of Pd(OAc)2 (0.05 molequiv), Cs2CO3, AdNH2.HCl (adamantanamine hydrochloride), in dioxane afforded 6-aminophenanthridine 452 (Scheme 155).
8. PALLADIUM-CATALYZED MULTI-COMPONENT REACTION
Recently there is flurry of activities in the development of efficient synthetic procedure that can affect multiple chemical reactions in a one-pot event.244,245 Palladium-catalysts help to synthesize the libraries of heterocyclic compounds1 by multicomponent reaction.
8.1. PALLADIUM-CATALYZED THREE-COMPONENT REACTION
A one-pot coupling of imines, carbon monoxide and acid chloride employing Pd2(dba)3.CHCl3 as catalyst and diimine ligand 455 afforded 3-amido-substituted-β-lactams in low-to-good yields (Scheme 156).246
The use of ionic liquid [bmim]PF6 for the cyclocarbonylation of phenols and anilines has been reported by Alper et al.247 Recently, the same group also demonstrated the efficiency of the ionic liquid [bmim]PF6 and Pd2(dba)3.CHCl3 for the multicomponent cyclocarbonylation of o-iodo aniline with substituted allenes under low pressure (5 atm) of carbon monoxide248 to give 3-methylene quinolone derivative 458 (Scheme 157). The ionic liquid functioned as solvent and promoter, enhanced the efficiency of the cyclocarbonylation.
Grigg et al.249 recently reported a novel three-component palladium-catalyzed allene insertion-nucleophile incorporation-Michael addition cascade reaction for the synthesis of novel tetrahydroisoquinolines 460. When aryl iodide, nucleophile, palladium catalyst and allene were reacted in the presence of K2CO3 in PhMe at 100 oC, the corresponding tetrahydroisoquinoline derivatives 460 were obtained in moderate-to-good yields (Scheme 158).
A new one-pot, three-component coupling strategy based on two consecutive metal-catalyzed reactions, providing a straightforward route to elaborate five-membered nitrogen heterocycles was developed.250 The coupling of three flexible and readily available starting materials: propargylamines 461, vinyl sulfones (or nitroalkenes) 462 and phenolic derivative is suggested to involve a copper-catalyzed cycloaddition combined with palladium-catalyzed allylic substitution to give polysubstituted pyrrolines 463a,b in two isomeric forms in 34-69% yields in a ratio ranging from 54:46 to 100:0 (Scheme 159).
8.2 PALLADIUM-CATALYZED FOUR-COMPONENT REACTION
Four component reaction of unactivated silylacetylenes, allyl carbonate and trimethylsilyl azide in the presence of bimetallic catalyst Pd2(dba)3.CHCl3-CuCl and phosphine ligand P(OEt)3 afforded the triazole derivative 467.251 In both the cases, a [3+2] cycloaddition reaction between initially generated copper acetylide and π-allyl palladium azide252 (Path a) or between allyl azide and π-allyl palladium methoxide (Path b) may occur to afford the triazole skeleton (Scheme 160).
A novel one-pot solution phase Ugi-Heck reaction (Ugi-4CR-Heck) for the preparation of indol-2-ones has been reported.253 The reaction is suggested to proceed via the intermediate Ugi product, which subsequently undergoes intramolecular Heck reaction to afford the indol-2-one derivatives 476 (Scheme 161).
9. MISCELLANEOUS REACTIONS
9.1. CYANOAMIDATION REACTION
The synthesis of α-alkylidene derivatives by the palladium-catalyzed intramolecular cyanoamidation of alkynyl cyanoformamides has recently been described.254 Different alkynyl cyanoformamides 477 underwent cyclization in the presence of Pd(PPh3)4 to afford the α-alkylidene derivatives 478 in 45-99% yields. The reaction proceeds exclusively in a 5-exo-manner. The other product 479 was formed probably via 1,3-migration of the C-4 proton (Scheme 162).
The same synthetic strategy has been applied to the synthesis of 4-membered lactam ring.255 The cyanoformamide 480 in the presence of Pd(PPh3)4 affoirded the lactam 481 (Scheme 163).
9.2. RING-OPENING CYCLIZATION REACTION
6-Vinyl-1,3-oxazinanone 482a underwent decarboxylative ring formation in the presence of Pd(PPh3)4 to afford 2-vinylazitidine 483.256 Notably, the ratio of the diastereomers in the product azitidine (16:1) was slightly lower than that of the starting material perhaps due to interconversion of the diastereomers on the time scale of the cyclization. The faster than cyclization, epimerization through π-σ-π allyl interconversation was further ascertained by the formation of 483 from cis-482b (Scheme 164).
Two separate mechanistic pathways, involving five-membered metallacycle 485 (Path a) or π-allyl complex 487 (Path b) are suggested to operate for the rapid formation of azitidine moiety. Reductive elimination from the five-membered metallacycle should give overall inversion of stereo-chemistry where as over all retention of stereo chemistry is observed due to the back side attack of the free nitrogen anion. Interestingly, it was observed that vinyl azitidine formed from 484 was shown to be anti isomer which clearly indicated a back side attack of the amide anion on the π-allyl ligand with over all retention of stereochemistry (Scheme 165).
Highly regioselective palladium-catalyzed ring opening and cyclization reaction of 2-vinylpyrrolidines 489 with aryl isocyanates 490 was carried out in the presence of Pd2(dba)3.CHCl3 and dppp at 40-60 oC in THF to furnish 1,3-diazepin-2-ones 491 in good isolated yield257 (Scheme 166).
The feasibility of NHC-Pd(0) complexes as viable catalysts for the diamination of conjugated diene was recently investigated.258 The reaction between di-t-butyldiaziridinone 493 as nitrogen source and 1-phenylbutadiene 492 was tested with several commercially available NHC-Pd complexes. The reaction is highly regio- and stereoselective with catalysts, (NHC)Pd(allyl)Cl complexes to produce the diaminated product 494 in moderate-to-excellent yields. The steric hindrance of NHC ligand played an important role in the reductive elimination step of the catalytic cycle as is evident in the higher conversion with more sterically hindered (IPr)Pd(allyl)Cl than IMesPd(allyl)Cl. Due to good solubility in organic solvent sodium t-pentoxide was found to be most effective for this diamination reaction (Scheme 167).
10. CONCLUSION
Hetrocyclic compounds are in high demand in chemical, pharmaceutical and agrochemical industries. The application of Pd-based catalysts in the synthesis of heterocycles is highly essential now because they are air-stable versatile in catalyzing a wide varity of chemical transformations and functionalities. In this review we have presented a portion of exiciting palladium chemistry which is now a powerful tool for the synthesis of nitrogen heterocycles.
Significant contributions have already been made in the synthesis of nitrogen heterocycles by Pd-catalyzed reactions. Unfortunately or fortunately new challenges are continuously forthcoming. Proper tuning of the reaction conditions and development of improved Pd-catalysts will lead to the development of new protocols that may allow synthesis of much more complex biologically active materials. We sincerely hope that this review will be useful not only for organic synthetic and organometallic chemists but also for heterocyclic natural product, pharmaceutical and agrochemical synthetic chemistry.
11. ACKNOWLEDGEMENTS
We thank the DST (New Delhi) for financial assistance. Both of us (B. C. and S. S.) is grateful to the CSIR (New Delhi) for a senior research fellowship.
References
1. (a) J. Tsuji, Palladium Reagents and Catalysts – New Perspectives for the 21st Century; John Wiley & Sons: New York, 2004; CrossRef (b) Palladium in Organic Synthesis; J. Tsuji, Ed.; Springer: Berlin, 2005; (c) In Handbook of Organopalladium Chemistry for Organic Synthesis; E. Negishi, Ed.; Wiley & Sons: New York, 2002, 2169.
2. (a) E. Negishi and L. Anastasia, Chem. Rev., 2003, 103, 1979; CrossRef (b) L. A. Agrofoglio, I. Gillaizeau, and Y. Saito, Chem. Rev., 2003, 103, 187; CrossRef (c) I. Nakamura and Y. Yamamoto, Chem. Rev., 2004, 104, 2127; CrossRef (d) S. Cacchi and G. Fabrizi, Chem. Rev., 2005, 105, 2873; CrossRef (e) G. Zeni and R. C. Larock, Chem. Rev., 2006, 106, 4644; CrossRef (f) E. M. Beccalli, G. Broggini, M. Martinelli, and S. Sottocornola, Chem. Rev., 2007, 107, 5318; CrossRef (g) D. Conreaux, D. Bouyssi, N. Monteiro, and G. Balme, Curr. Org. Chem., 2006, 10, 1325; CrossRef (h) S. Cacchi, G. Fabrizi, and A. Goggiamani, Curr. Org. Chem., 2006, 10, 1423; CrossRef (i) B. Gabriele, G. Salerno, and M. Costa, Synlett., 2004, 2468; CrossRef (j) G. Zeni and R. C. Larock, Chem. Rev., 2004, 104, 2285. CrossRef
3. (a) A. B. Dounay and L. E. Overman, Chem. Rev., 2003, 103, 2945; CrossRef (b) H. P. Bell, H. Ila, and L. F. Tietze, Chem. Rev., 2004, 104, 3453; CrossRef (c) P. J. Guiry and H. A. McManus, Chem. Rev., 2004, 104, 4151. CrossRef
4. (a) L. F. Tietze and F. Haunert, In Stimulating concepts in chemistry, F. Vögtle, J. F. Stoddart and M. Shibasaki, Eds., Wiley-VCH: Weinheim, 2000, 39; CrossRef (b) L. F. Tietze and A. Modi, Med. Chem. Rev., 2000, 20, 304; (c) C. E. Garret and K. Prasad, Adv. Synth. Catal., 2004, 346, 889. CrossRef
5. (a) G. Dyker, In Handbook of Organopalladium Chemistry for Organic Synthesis, E. Negishi, Ed.; John Wiley & Sons: New York, 2000, IV.2.2.2,1255; (b) L. F. Tietze, H. Schirok and M. Wöhrmann, Chem. Eur. J., 2000, 6, 510; CrossRef (c) R. Grigg and V. Savic, Chem. Commun., 2000, 873; CrossRef (d) L. F. Tietze, K. Thede, R. Schimpf, and F. Sannicolό, Chem. Commun., 2000, 583; CrossRef (e) L. Bhat, A. G. Steinig, R. Appelbe, and A. de Meijere, Eur. J. Org. Chem., 2001, 1673; CrossRef (f) K. Wakabayashi, H. Yorimitsu, and K. Oshima, J. Am. Chem. Soc., 2001, 123, 5374. CrossRef
6. R. F. Heck, Org. React., (N.Y.) 1982, 27, 345.
7. A. Jutand, Eur. J. Inorg. Chem., 2003, 2017. CrossRef
8. K. Fagnou and M. Lautens, Angew. Chem., Int. Ed., 2002, 41, 26. CrossRef
9. (a) U. Anwar, A. Casaschi, R. Grigg and J. M. Sansano, Tetrahedron, 2001, 57, 1361; CrossRef (b) A. de Meijere and S. Bräse, J. Organomet. Chem., 1999, 576, 88; CrossRef (c) G. Poli, G. Giambastiani, and A. Heumann, Tetrahedron, 2000, 56, 5959; CrossRef (d) M. Lautens, E. Tayama, and C. Herse, J. Am. Chem. Soc., 2005, 127, 72. CrossRef
10. (a) M. Bauer and M. E. Maier, Org. Lett., 2002, 4, 2205; CrossRef (b) G. A. Holloway, H. M. Hügel, and M. A. Rizzacasa, J. Org. Chem., 2003, 68, 2200; CrossRef for Heck Macrocyclization see, (c) K. Akaji, K. Teruya, M. Akaji, and S. Aimoto, Tetrahedron, 2001, 57, 2293; CrossRef (d) V. Balraju, D. S. Reddy, M. Periasamy, and J. Iqbal, Tetrahedron Lett., 2005, 46, 5207; CrossRef for Suzuki Macrocyclization (e) W. Li and K. Burgess, Tetrahedron Lett., 1999, 40, 6527; CrossRef for Stille Macrocyclization (f) H. Deng, J.-K. Jung, T. Liu, K. W. Kuntz, M. L. Snapper, and A. H. Hoveyda, J. Am. Chem. Soc., 2003, 125, 9032. CrossRef
11. (a) D. Solé, L. Vallverdú, E. Peidró, and J. Bonjoch, Chem. Commun., 2001, 1888; CrossRef (b) D. Solé, L. Vallverdú, and J. Bonjoch, Adv. Synth. Catal., 2001, 343, 439; CrossRef (c) O. Gaertzen and S. L. Buchwald, J. Org. Chem., 2002, 67, 465; CrossRef (d) D. Solé, L. Vallverdú, X. Solans, M. Font-Bardı´a, and J. Bonjoch, J. Am. Chem. Soc., 2003, 125, 1587; CrossRef (e) J. Bonjoch, F. Diaba, G. Puigbó, E. Peidró, and D.Solé, Tetrahedron Lett., 2003, 44, 8387; CrossRef (f) D. A. Culkin and J. F.Hartwig, Acc. Chem. Res., 2003, 36, 234. CrossRef
12. (a) T. Honda, H. Namiki, and F. Satoh, Org. Lett., 2001, 3, 631; CrossRef (b) R. Imbos, A. J. Minnaard, and B. L. Feringa, J. Am. Chem. Soc., 2002, 124, 184; CrossRef (c) R. Ferraccioli, D. Carenzi, and M. Catellani, Synlett, 2002, 1860; CrossRef (d) D. Solé, F. Diaba, and J. Bonjoch, J. Org. Chem., 2003, 68, 5746. CrossRef
13. (a) Y. Fukuyama, H. Yuasa, Y. Tonoi, K. Harada, M. Wada, Y. Asakawa, and T. Hashimoto, Tetrahedron, 2001, 57, 9299; CrossRef (b) B. M. Trost, J. Org. Chem., 2004, 69, 5813; CrossRef (c) Q. Huang, A. Fazio, G. Dai, M. A. Campo, and R. C. Larock, J. Am. Chem. Soc., 2004, 126, 7460. CrossRef
14. R. C. Larock and S. Babu, Tetrahedron Lett., 1987, 28, 5291. CrossRef
15. (a) M. T. Reetz and J. G. de Vries, Chem. Commun., 2004, 1559; CrossRef (b) M. B. Thathager, J. E. ten Elshof, and G. Rothenberg, Angew. Chem., Int. Ed., 2006, 45, 2886; CrossRef (c) M. B. Thathager, P. J. Kooyman, R. Boerleider, E. Jansen, and C. J. Vlsevir, Adv. Synth. Catal., 2005, 347, 1965; CrossRef (d) C. C. Cassol, A. P. Umpierre, G. Machado, S. I. Wolke, and J. Dupont, J. Am. Chem. Soc., 2005, 127, 3298. CrossRef
16. P. Liu, L. Huang, Y. Lu, M. Dilmeghani, J. Baum, T. Xiang, J. Adams, A. Tasker, R. Larsen, and M. M. Faul, Tetrahedron Lett., 2007, 48, 2307. CrossRef
17. C. Gutler and S. L. Buchwald, Chem. Eur. J., 1999, 5, 3107. CrossRef
18. G. Y. Li, G. Zheng, and A. F. Noonan, J. Org. Chem., 2001, 66, 8677. CrossRef
19. E. M. Beccalli, G. Broggini, M. Martinelli, N. Masciocchi, and S. Sottocornola, Org. Lett., 2006, 8, 4521. CrossRef
20. A. Ashimori, B. Bachand, M. A. Calter, S. P. Govek, L. E. Overman, and D. J. Poon, J. Am. Chem. Soc., 1998, 120, 6488. CrossRef
21. M. C. McDermott, G. R. Stephenson, D. L. Hughes, and A. J. Walkington, Org Lett., 2006, 8, 2917. CrossRef
22. (a) C. H.-T. Chen, D. P. Curran, and W. Liu, J. Am. Chem. Soc., 1999, 121, 11012; CrossRef (b) D. P. Curran, S. J. Geib, and M. Petit, Tetrahedron, 2004, 60, 7543. CrossRef
23. A. J. von Wangelin, H. Neumann, D. Gördes, S. Hübner, C. Wendler, S. Klaus, D. Strübing, A Spannenberg, H. Jiao, L. El Firdoussi, K. Thurow, N. Stoll, and M. Beller, Synthesis, 2005, 2029. CrossRef
24. A. Madin, C. J. O’Donnell, T. Oh, D. W. Old, L. E. Overman, and M. J. Sharp, J. Am. Chem. Soc.,
2005, 127, 18054. CrossRef
25. V. Gracias, J. D. Moore, and S. W. Djuric, Tetrahedron Lett., 2004, 45, 417. CrossRef
26. G. D. Artman III and S. M. Weinreb, Org. Lett., 2003, 5, 1523. CrossRef
27. H. Fuwa and M. Sasaki, Chem. Commun., 2007, 2876. CrossRef
28. G. R. Humphrey and J. T. Kuethe, Chem. Rev., 2006, 106, 2875. CrossRef
29. H. Fuwa and M. Sasaki, Org. Lett., 2007, 9, 3347. CrossRef
30. (a) R. Di Fabio, F. Micheli, D. Baraldi, B. Bertani, N. Conti, G. Dal Forno, A. Feriani, D. Donati, C. Marchioro, T. Messeri, A. Missio, A. Pasquarello, G. Pentassuglia, D. A. Pizzi, S. Provera, A. M. Quaglia, and F. M. Sabbatini, Farmaco, 2003, 58, 723; CrossRef (b) L. A. Arnold, W. Luo, and R. K. Guy, Org. Lett., 2004, 6, 3005; CrossRef (c) S. E. Gibson, J. O. Jones, S. B. Kalindjinan, J. D. Knight, J. W. Steed, and M. J. Tozer, J. Chem. Soc., Chem. Commun., 2002, 1938; CrossRef (d) L. F. Tietze, K. M. Sommer, G. Schneider, P. Tapolzsányi, J. Wölfling, P. Müller, M. Noltemeyer, and H. Terlau, Synlett, 2003, 1494. CrossRef
31. M. Quadir, J. Cobb, P. W. Sheldrahe, N. Whittal, A. J. P. White, K. K. (M). Hii, P. N. Horton, and M. B. Hursthouse, J. Org. Chem., 2005, 70, 1545. CrossRef
32. E. L. Cropper, A. J. P. White, A. Ford, and K. K. (M). Hii, J. Org. Chem., 2006, 71, 1732. CrossRef
33. P. Ribiere, V. Declerck, Y. Nedellec, N. Yadav-Bhatnagar, J. Martinez, and F. Lamaty, Tetrahedron, 2006, 62, 10456. CrossRef
34. A. V. Kalinin, B. A. Chauder, S. Rakhit, and V. Snieckus, Org. Lett., 2003, 5, 3519. CrossRef
35. S. County, B. Liegault, C. Meyer, and J. Cossy, Tetrahedron, 2006, 62, 3882. CrossRef
36. S. County, B. Liegault, C. Meyer, and J. Cossy, Org. Lett., 2004, 6, 2511. CrossRef
37. S.-H. Min, S.-J. Pang, and C.-G. Cho, Tetrahedron Lett., 2003, 44, 4439. CrossRef
38. R. Yanada, S. Obika, T. Inokuma, K. Yanada, M. Yamashita, S. Ohta, and Y. Takemoto, J. Org. Chem., 2005, 70, 6972. CrossRef
39. W. S. Cheung, R. J. Patch, and M. R. Player, J. Org. Chem., 2005, 70, 3741. CrossRef
40. M. B. Andrus and C. Song, Org. Lett., 2001, 3, 3761. CrossRef
41. J. P. Wolf, R. A. Singer, B. H. Yang, and S. L. Buchwald, J. Am. Chem. Soc., 1999, 121, 9550. CrossRef
42. P. A. Donets and E. V. der Eycken, Org. Lett., 2007, 9, 3017. CrossRef
43. N. Arai, M. Takahashi, M. Mitani, and A. Mori, Synlett, 2006, 3170. CrossRef
44. R. M. de Figueiredo, S. Thoret, C. Huet, and J. Dubois, Synthesis, 2007, 529. CrossRef
45. K. C. Majumdar, B. Chattopadhyay, and A. Taher, Synthesis, 2007, 3647. CrossRef
46. L. Joucla, A. Putey, and B. Joseph, Tetrahedron Lett., 2005, 46, 8177. CrossRef
47. C. W. G. Fishwick, R. Grigg, V. Sridharan, and J. Virica, Tetrahedron, 2003, 59, 4451. CrossRef
48. P. R Reddy, V. Balraju, G. R. Madhaban, B. Banerji, and J. Iqbal, Tetrahedron Lett., 2003, 44, 353. CrossRef
49. A. C. Spivey, J. McKendrick, and R. Srikaran, J. Org. Chem., 2003, 68, 1843. CrossRef
50. V. Balraju, S. D. Reddy, M. Periasamy, and J. Iqbal, J. Org. Chem., 2005, 70, 9626. CrossRef
51. B. Alcaide, P. Almendros, and R. Rodriguez-Acebes, J. Org. Chem., 2005, 70, 2713. CrossRef
52. H. Ohno, M. Yamamoto, M. Iuchi, and T. Tanaka, Angew. Chem., Int. Ed., 2005, 44, 5103. CrossRef
53. X. Wang and J. A. Jr. Porco, J. Am. Chem. Soc., 2003, 125, 6040. CrossRef
54. G. Kim, J. H. Kim, and K.Y. Lee, J. Org. Chem., 2006, 71, 2185. CrossRef
55. D. A. Culkin and J. F. Hartwig, J. Am. Chem. Soc., 2001, 123, 5816. CrossRef
56. D. Solé, X. Urbaneja, and J. Bonjoch, Tetrahedron Lett., 2004, 45, 3131. CrossRef
57. (a) R. Tamura, A. Kamimura, and N. Ono, Synthesis, 1991, 423; CrossRef (b) H. Muratake and H. Nakai, Tetrahedron Lett., 1999, 40, 2355; CrossRef (c) E. M. Vogl and S. L. Buchwald, J. Org. Chem., 2002, 67, 106. CrossRef
58. J. Tsuji, Palladium Reagents and Catalysts-Innovations in Organic Synthesis; Willey: Chichester, 1995.
59. D. Solé, X. Urbaneja, and J. Bonjoch, Org. Lett., 2005, 7, 5461. CrossRef
60. (a) S. Thorimbert, G. Giambastiani, C. Commandeur, M. Vitale, G. Poli, and M. Malacria, Eur. J. Org. Chem., 2003, 2702; CrossRef (b) S. Lemaire, G. Giambastiani, G. Prestat, and G. Poli, Eur. J. Org. Chem., 2004, 2840. CrossRef
61. For generalities on phase-transfer catalysis, see: (a) E. V. Demlow and S. S. Demlow, Phase Transfer Catalysis, 3rd ed.; VCH: Weinheim, 1993; (b) C. M. Starks, C. L. Liotta, and M. Halpern, Phase Transfer Catalysis: Fundamentals, Applications, and Industrial Perspectives; Chapman & Hall: New York, 1994.
62. (a) M. Nakoji, T. Kanayama, T. Okino, and Y. Takemoto, J. Org. Chem., 2002, 67, 7418; CrossRef (b) M. Nakoji, T. Kanayama, T. Okino, and Y. Takemoto, Org. Lett., 2001, 3, 3329; CrossRef (c) G. Chen, Y. Deng, L. Gong, A. Mi, X. Cui, Y. Jiang, M. C. K. Choi, and A. S. C. Chan, Tetrahedron Asymmetry, 2001, 12, 1567. CrossRef
63. D. Madec, G. Prestat, E. Martini, P. Fristrup, G. Poli, and P.-O. Norrby, Org. Lett., 2005, 7, 995. CrossRef
64. C. Amatore, A. Jutand, M. A. M’Barki, G. Meyer, and L. Mottier, Eur. J. Inorg. Chem., 2001, 873. CrossRef
65. B. Lygo and B. Andrews, Acc. Chem. Res., 2004, 37, 518. CrossRef
66. K.-T. Yip, J.-H. Li, O.-Y. Lee, and D. Yang, Org. Lett., 2005, 7, 5717. CrossRef
67. D. Yang, J.-H. Li, Q. Gao, and Y.-L. Yan, Org. Lett., 2003, 5, 2869. CrossRef
68. (a) F. Kakiuchi and S. Murai, Acc. Chem. Res., 2002, 35, 826; CrossRef (b) F. Kakiuchi and N. Chatani, Adv. Synth. Catal., 2003, 345, 1077; CrossRef (c) L.-C. Campeau and K. Fagnou, Chem. Commun., 2006, 1253; CrossRef (d) C. G. Espino and J. Du Bois, In Modern Rhodium-Catalyzed Organic Reactions; P. A. Evans, Ed.; Wiley-VCH: Weinheim, Germany, 2005, 379; (e) H. M. L. Davies, Angew. Chem., Int. Ed., 2006, 45, 6422; CrossRef (f) A. R. Dick and M. S. Sanford, Tetrahedron, 2006, 62, 2439; CrossRef (g) I. V. Seregin and V. Gevorgyan, J. Chem. Soc., Chem. Rev., 2007, 36, 1173; CrossRef (h) K. Godula and D. Sames, Science, 2006, 312, 67. CrossRef
69. (a) V. Ritleng, C. Sirlin, and M. Pfeffer, Chem. Rev., 2002, 102, 1731; CrossRef (b) C.-J. Li, Acc. Chem. Res., 2002, 35, 533; CrossRef (c) C. Jia, T. Kitamura, and Y. Fujiwara, Acc. Chem. Res., 2001, 34, 633; CrossRef (d) C. Jia, D. Piao, J. Oyamada, W. Lu, T. Kitamura, and Y. Fujiwara, Science, 2000, 287, 1992. CrossRef
70. (a) D. E. Ames and A. Opalko, Synthesis, 1983, 234; CrossRef (b) W. S. Yue and J. J. Li, Org. Lett. , 2002, 4, 2201; CrossRef (c) M. Lautens, J.-F. Paquin, and S. Piguel, J. Org. Chem., 2002, 67, 3972; CrossRef (d) S. Pache and M. Lautens, Org. Lett., 2003, 5, 4827; CrossRef (e) D. Alberico, J.-F. Paquin, and M. Lautens, Tetrahedron, 2005, 61, 6283; CrossRef (f) A. Martins, U. Marquardt, N. Kasravi, D. Alberico, and M. Lautens, J. Org. Chem., 2006, 71, 4937; CrossRef (g) D. Alberico and M. Lautens, Synlett, 2006, 2969; CrossRef (h) T. A. Dwight, N. R. Rue, D. Charyk, R. Josselyn, and B. Deboef, Org. Lett., 2007, 9, 3137. CrossRef
71. (a) M. Catellani, In Handbook of Organopalladium Chemistry for Organic Synthesis; E. I. Negishi and A. de Meijere, Eds.; John Wiley & Sons: Hoboken, NJ, 2002, 1479; (b) M. Catellani, Synlett, 2003, 298; CrossRef (c) J. Tsuji, Palladium Reagents and Catalysis-New Perspectives for the 21st Century; John Wiley & Sons: New York, 2004, 409. CrossRef
72. (a) R. Giri, N. Maugel, J.-J. Li, D.-H. Wang, S. P. Breazzano, L. B. Saunders, and J.-Q. Yu, J. Am. Chem. Soc., 2007, 129, 3510; CrossRef (b) X. Chen, C. E. Goodhue, and J.- Q. Yu, J. Am. Chem. Soc., 2006, 128, 12634; CrossRef (c) V. G. Zaitsev, D. Shabashov, and O. Daugulis, J. Am. Chem. Soc., 2005, 127, 13154; CrossRef (d) D. Shaabashov and O. Daugulis, Org. Lett., 2005, 7, 3657; CrossRef (e) T. E. Barder, S. D. Walker, J. R. Martinelli, and S. L. Buchwald, J. Am. Chem. Soc., 2005, 127, 4685; CrossRef (f) H. Ren, and P. Knochel, Angew. Chem., Int. Ed., 2006, 45, 3462; CrossRef (g) C.-G. Dong, and Q.-G. Hu, Angew. Chem., Int. Ed., 2006, 45, 2289; CrossRef (h) S. J. Pastine, D. V. Gribkov, and D. Sames, J. Am. Chem. Soc., 2006, 128, 14220. CrossRef
73. (a) Z. Li and C.-J. Li, J. Am. Chem. Soc., 2004, 126, 11810; CrossRef (b) Z. Li and C.-J. Li, J. Am. Chem. Soc., 2005, 127, 6968; CrossRef (c) B. Deboef, S. J. Pastine, and D. Sames, J. Am. Chem. Soc., 2004, 126, 6556; CrossRef (d) H. Chen, L. Gan, Y. Shi, and X. Wei, J. Org. Chem., 2001, 66, 6369; CrossRef (e) Z. Li and C.-J. Li, J. Am. Chem. Soc., 2005, 127, 3672. CrossRef
74. X. Wan, D. Xing, Z. Fang, B. Li, F. Zhao, K. Zhang, L. Yang, and Z. Shi, J. Am. Chem. Soc., 2006, 128, 12046. CrossRef
75. (a) D. Alberico, M. E. Scott, and M. Lautens, Chem. Rev., 2007, 107, 174; CrossRef (b) G. Dyker, Angew. Chem., Int. Ed., 1999, 38, 1698; CrossRef (c) J. P. Wolfe and J. S. Thomas, Curr. Org. Chem., 2005, 9, 625. CrossRef
76. (a) F. Bellina, S. Cauteruccio, L. Mannina, R. Rossi, and S. Viel, J. Org. Chem., 2005, 70, 3997; CrossRef (b) J. G. Zeevaart, C. J. Parkinson, and C. B. de Koning, Tetrahedron Lett., 2004, 45, 4261; CrossRef (c) A. Yokooji, T. Okazawa, T. Satoh, M. Miura, and M. Nomura, Tetrahedron, 2003, 59, 5685; CrossRef (d) I. E. Nifant’ev, A. A. Sitnikov, N. V. Andriukhova, I. P. Laishevtsev, and Y. N.Luzikov, Tetrahedron Lett., 2002, 43, 3213; CrossRef (e) K. Li, Y. Zeng, B. Neuenswander, and J. A. Tunge, J. Org. Chem., 2005, 70, 6515; CrossRef (f) K. Inamoto, T. Saito, M. Katsuno, T. Sakamoto, and K. Hiroya, Org. Lett., 2007, 9, 2931. CrossRef
77. M. P. Krahl, A. Jäger, T. Krause, and H. -J. Knölker, Org. Biomol. Chem., 2006, 4, 3215. CrossRef
78. J.-P. Leclere, M. André, and K. Fagnou, J. Org. Chem., 2006, 71, 1711. CrossRef
79. S. Hostyn, B. U. W. Maes, G. V. Baelen, A. Gulevskaya, C. Meyers, K. Smits, Tetrahedron, 2006, 62, 4676. CrossRef
80. K.-F. Lindahl, A. Carrol, R. J. Quinn, and J. A. Ripper, Tetrahedron Lett., 2006, 47, 7493. CrossRef
81. T. Kuroda and F. Suzuki, Tetrahedron Lett., 1991, 32, 6915. CrossRef
82. C. Bressy, D. Alberico, and M. Lautens, J. Am. Chem. Soc., 2005, 127, 13148. CrossRef
83. C. Blaszykowski, E. Aktoudianakis, C. Bressy, D. Alberico, and M. Lautens, Org. Lett., 2006, 8, 2043. CrossRef
84. D. G. Hulcoop and M. Lautens, Org. Lett., 2007, 9, 1761. CrossRef
85. F. Jafarpour and M. Lautens, Org. Lett., 2006, 8, 3601. CrossRef
86. A. Pinto, L. Neuville, P. Retailleau, and J. Zhu, Org. Lett., 2006, 8, 4927. CrossRef
87. B. Martin-Matute, C. Mateo, D. J. Cardenas, and A. M. Echavarren, Chem. Eur. J., 2001, 7, 2341. CrossRef
88. (a) A. J. Mota, A. Dedieu, C. Bour, and J. Suffert, J. Am. Chem. Soc., 2005, 127, 7171; CrossRef (b) D. L. Davies, S. M. A. Donald, and S. A. Macgregor, J. Am. Chem. Soc., 2005, 127, 13754; CrossRef (c) D. Garcia-Cuadrado, A. A. C. Braga, F. Maseras, and A. M. Echavarren, J. Am. Chem. Soc., 2006, 128, 1066. CrossRef
89. C. Xie, Y. Zhang, Z. Huang, and P. Xu, J. Org. Chem., 2007, 72, 5431. CrossRef
90. (a) I. D. Hills, W. R. Netherton, and G. C. Fu, Angew. Chem., Int. Ed., 2003, 72, 5749; CrossRef (b) B. F. Landeros, and J. F. Hartwig, J. Am. Chem. Soc., 2005, 127, 6944. CrossRef
91. (a) H. H. A. Linde, Helv. Chim. Acta., 1965, 48, 1822; CrossRef (b) D. J. Abraham, R. D. Rosenstein, R. L. Lyon, and H. H. S. Fong, Tetrahedron Lett., 1972, 13, 909. CrossRef
92. A. L. Bowie, C. H. Chambers, and D. Trauner, Org. Lett., 2005, 7, 5207. CrossRef
93. M. C. Harris, O. Geis, and S. L. Buchwald, J. Org. Chem., 1999, 64, 6019. CrossRef
94. (a) S. Pivas-Art, T. Satoh, Y. Kawamura, M. Miura, and M. Nomura, Bull. Chem. Soc. Jpn., 1998, 71, 467; CrossRef (b) T. Okazawa, T. Satoh, M. Miura, and M. Nomura, J. Am. Chem. Soc., 2002, 124, 5286. CrossRef
95. (a) H. Ohno, K. Miyamura, Y. Takeoka, and T. Tanaka, Angew. Chem., Int. Ed., 2003, 42, 2647; CrossRef (b) H. Ohno, M. Yamamoto, M. Iuchi, and T. Tanaka, Angew. Chem., Int. Ed., 2005, 44, 5103; CrossRef (c) H. Ohno, K. Miyamura, T. Mizutani, Y. Kadoh, Y. Takeoka, H. Hamaguchi, and T. Tanaka, Chem. Eur. J., 2005, 11, 3728. CrossRef
96. H. Ohno, M. Iuchi, N. Fujii, and T. Tanaka, Org. Lett., 2007, 9, 4813. CrossRef
97. (a) C. Bour and J. Suffert, Org. Lett., 2005, 7, 653; CrossRef (b) J. Zhao, M. A. Campo, and R. C. Larock, Angew. Chem., Int. Ed., 2005, 44, 1873; CrossRef (c) H.-J. Knolker, R. R. Kethiri, Chem. Rev., 2002, 102, 4303; CrossRef (d) E. Duval, and G. D. Cuny, Tetrahedron Lett., 2004, 45, 5411. CrossRef
98. (a) J. Zhao and R. C. Larock, Org. Lett., 2005, 7, 701; CrossRef (b) J. Zhao and R. C. Larock, J. Org. Chem., 2006, 71, 5340. CrossRef
99. (a) J. Zhao and R. C. Larock, Org. Lett., 2005, 7, 701; CrossRef (b) J. Zhao and R. C. Larock, J. Org. Chem., 2006, 71, 5340. CrossRef
100. F. Faccini, E. Motti, and M. Catellani, J. Am. Chem. Soc., 2004, 126, 78 and references therein. CrossRef
101. (a) B. M. Trost and F. D. Toste, J. Am. Chem. Soc., 2000, 122, 714; CrossRef (b) P. Cao, B. Wang, and X. Zhang, J. Am. Chem. Soc., 2000, 122, 6490; CrossRef (c) Y. Yamamoto, Y. Nakagai, N. Ohkoshi, and K. Itoh, J. Am. Chem. Soc., 2001, 123, 6372; CrossRef (d) K. M. Brummond, H. Chen, P. Sill, and L. You, J. Am. Chem. Soc., 2002, 124, 15186. CrossRef
102. A. Hercouet, F. Berrée, C. H. Lin, L. Toupet, and B. Carboni, Org Lett., 2007, 9, 1717. CrossRef
103. (a) G. Zhu, X. Tong, J. Cheng, Y. Sun, D. Li, and Z. Zhang, J. Org. Chem., 2005, 70, 1712; CrossRef (b) G. Zhu and Z. Zhang, Org. Lett., 2003, 5, 3645. CrossRef
104. H. Ohno, Y. Takeoka, Y. Kadoh, K. Miyamura, and T. Tanaka, J. Org. Chem., 2004, 69, 4541. CrossRef
105. H. Ohno, M. Anzai, A. Toda, S. Ohishi, N. Fujii, T. Tanaka, Y. Takemoto, and T. Ibuka, J. Org. Chem., 2001, 66, 4904. CrossRef
106. H. Ohno, Y. Takeoka, K. Miyamura, Y. Kadoh, and T. Tanaka, Org. Lett., 2003, 5, 4763. CrossRef
107. M. Bandini, A. Melloni, F. Piccinelli, R. Sinisi, S. Tommasi, and A. Umani-Ronchi, J. Am. Chem. Soc., 2006, 128, 1424. CrossRef
108. (a) C. H. Oh, H. H. Jung, J. S. Kim, and S. W. Cho, Angew. Chem., Int. Ed., 2000, 39, 752; CrossRef (b) C. H. Oh, C. Y. Rhim, M. Kim, D. I. Park, and A. K. Gupta, Synlett, 2005, 2694. CrossRef
109. C. H. Oh, H. M. Park, and D. I. Park, Org. Lett., 2007, 9, 1191. CrossRef
110. V. Balraju, R. V. Dev, D. S. Reddy, and J. Iqbal, Tetrahedron Lett., 2006, 47, 3569. CrossRef
111. J. Song, Q. Shen, F. Xu, and X. Lu, Tetrahedron, 2007, 63, 5148. CrossRef
112. G. Zhu and Z. Zhang, J. Org. Chem., 2005, 70, 3339. CrossRef
113. C.–M. Yu, J. Youn, and M.–K. Lee, Org. Lett., 2005, 7, 3733. CrossRef
114. (a) S. Yamago and E. Nakamura, Org. React., 2002, 61, 1; (b) A. Brandi, S. Cachi, F. M. Cordero, and A. Goti, Chem. Rev., 2003, 103, 1213; CrossRef (c) A. Delgado, J. R. Rodríguez, L. Castedo, and J. L. Mascarenãs, J. Am. Chem. Soc., 2003, 125, 9282. CrossRef
115. B. M. Trost, R. C. Bunt, R. C. Lemoine, and T. L. Calins, J. Am. Chem. Soc., 2000, 122, 5968. CrossRef
116. C. Larksarp and H. Alper, J. Org. Chem., 2001, 66, 3502. CrossRef
117. B. M. Trost, S. M. Silverman, and J. P. Stambuli, J. Am. Chem. Soc., 2007, 129, 12398. CrossRef
118. M. Gulías, R. García, A. Delgado, L. Castedo, and J. L. Mascarenãs, J. Am. Chem. Soc., 2006, 128, 384. CrossRef
119. A. Kong, X. Han, and X. Lu, Org. Lett., 2006, 8, 1339. CrossRef
120. C. Chowdhury, S. B. Mandal, and B. Achari, Tetrahedron Lett., 2005, 46, 8531. CrossRef
121. J. Barluenga, C. Valdes, G. Beltrán, M. Escribano, and F. Aznar, Angew. Chem., Int. Ed., 2006, 45, 6893. CrossRef
122. J. G. Knight, K. Tchabanenko, P. A. Stoker, and S. J. Harwood, Tetrahedron Lett., 2005, 46, 6261. CrossRef
123. (a) R. P. Hsung, L.-L. Wei, H. M. Sklenicka, H. C. Shen, M. J. McLaughlin, and L. R. Zehnder, Trends Heterocycl. Chem., 2001, 7, 1; (b) S. J. Hedley, W. J. Moran, D. A. Price, and J. P. A. Harrity, J. Org. Chem., 2003, 68, 4286; CrossRef (c) K. M. Goodenough, W. J. Moran, P. Raubo, and J. P. A. Harrity, J. Org. Chem., 2005, 70, 207. CrossRef
124. R. Shintani and T. Hayashi, J. Am. Chem. Soc., 2006, 128, 6330. CrossRef
125. (a) K. A. Jorgensen, Angew, Chem. Int. Ed., 2000, 39, 3558; CrossRef (b) K. Mikami, K.Aikawa, Y. Yusa, and M. Hatano, Org. Lett., 2002, 4, 91; CrossRef (c) K. Mikami and K. Aikawa, Org. Lett., 2002, 4, 99; CrossRef (d) K. Mikami and H. Ohmura, Org. Lett., 2002, 4, 3355. CrossRef
126. H. Zhang and A. Padwa, Org. Lett., 2006, 8, 247. CrossRef
127. (a) I. U. Khand, G. R. Knox, P. L. Pauson, W. E. Watts, and M. I. Foreman, J. Chem. Soc., Perkin Trans. 1, 1973, 977; CrossRef (b) O. Geis and H. G. Schmalz, Angew. Chem., Int. Ed., 1998, 37, 911; CrossRef (c) L. V. R. Bonaga and M. E. Krafft, Tetrahedron, 2004, 60, 9795. CrossRef
128. Y. Tang, L. Deng, Y. Zhang, G. Dong, J. Chen, and Z. Yang, Org. Lett., 2005, 7, 593. CrossRef
129. Y. Tang, L. Deng, Y. Zhang, G. Dong, J. Chen, and Z. Yang, Org. Lett., 2005, 7, 1657. CrossRef
130. (a) S. R. Fix, J. L. Brice, and S. S. Stahl, Angew. Chem., Int. Ed., 2002, 41, 164; CrossRef (b) R. W. Bates and K. Sa-Ei, Org. Lett., 2002, 4, 4225; CrossRef (c) H. Sasai, Tetrahedron Lett., 2003, 44, 711. CrossRef
131. E. M. Beccalli, G. Broggini, G. Paladino, A. Penoni, C. Zoni, J. Org. Chem., 2004, 69, 5627. CrossRef
132. (a) B. M. Trost, Acc. Chem. Res., 1980, 13, 385; CrossRef (b) P. A. Van der Schaaf, J.-P. Sutter, M. Grellier, G. P. M. Van Mier, A. L. Spek, G. Van Koten and M. Pfeffer, J. Am. Chem. Soc., 1994, 116, 5134. CrossRef
133. (a) M. M. Rogers and S. S. Stahl, N-Heterocyclic Carbenes in Transition Metal Catalysis; Glorius, F., Ed.; Springer: New York, 2006; (b) C. C. Scarborough, M. J. W. Grady, I. A.Guzei, B. A. Gandhi, E. E. Bunel, and S. S. Stahl, Angew. Chem., Int. Ed., 2005, 44, 5269; CrossRef (c) J. A. Mueller, C. P. Goller, and M. S. Sigman, J. Am. Chem. Soc., 2004, 126, 9724. CrossRef
134. M. M. Rogers, J. E. Wendlandt, I. A. Guzei, and S. S. Stahl, Org. Lett., 2006, 8, 2257. CrossRef
135. B. Ferber, S. Lemaire, M. M. Mader, G. Prestat, and G. Poli, Tetrahedron Lett., 2003, 44, 4213. CrossRef
136. B. Ferber, G. Prestat, S. Vogel, D. Madec, and G. Iovanni Poli, Synlett, 2006, 2133. CrossRef
137. J. Eustache, P. V. de Weghe, D. L. Nouen, H. Uyehara, C. Kabuto, and Y. Yamamoto, J. Org. Chem., 2005, 70, 4043. CrossRef
138. K. Ito, S. Akashi, B. Saito, and T. Katsuki, Synlett, 2003, 1809. CrossRef
139. T. Oshitari, R. Akagi, and T. Mandai, Synthesis, 2004, 1325. CrossRef
140. (a) J. Tsuji, Transition Metal Reagents and Catalysts; John Wiley & Sons Ltd., New York, 2000, 305; (b) B. M. Trost, F. D. Toste, and A. B. Pinkerton, Chem. Rev., 2001, 101, 2067. CrossRef
141. B. M. Trost, M. R. Machacek, B. D. Faulk, J. Am. Chem. Soc., 2006, 128, 6745. CrossRef
142. P. Szolcsányi and T. Gracza, Tetrahedron, 2006, 62, 8498. CrossRef
143. (a) I. J. S. Fairlamb, A. F. Lee, F. E. M. loe-Mie, E. H. Niemela, C, T. O’Brien, and A. C. Whitwood, Tetrahedron, 2005, 61, 9827; CrossRef (b) A. S. Batsanov, J. C. Collings, I. J. S. Fairlamb, J. P. Holland, J. A. K. howard, Z. Lin, T. B. Marder, A. C. Parsons, R. M. Ward, and J. Zhu, J. Org. Chem., 2005, 70, 703. CrossRef
144. J. E. Ney and J. P. Wolfe, Angew. Chem., Int. Ed., 2004, 43, 3605. CrossRef
145. Q. Yang, J. E. Ney, and J. P. Wolfe, Org. Lett., 2005, 7, 2575. CrossRef
146. (a) R. E. Schwartz, J. Liesch, O. Hensens, L. Zitano, S. Honeycutt, G. Garrity, R. A. Fromtling, J. Onishi, and R. Monaghan, J. Antibiot., 1988, 41, 177; (b) J. H. Johnson, D. W. Phillipson, and A. D. Kahle, J. Antibiot., 1989, 42, 1184.
147. M. Beaudoin and J. P. Wolfe, Org. Lett., 2006, 8, 2353. CrossRef
148. J. A. Fritz, J. S. Nakhla, and J. P. Wolfe, Org. Lett., 2006, 8, 2531. CrossRef
149. B. M. Trost and J. Quancard, J. Am. Chem. Soc., 2006, 128, 6314. CrossRef
150. Y. Tamaru, Eur. J. Org. Chem., 2005, 2647. CrossRef
151. C. S. Nylund, J. M. Klopp, and S. M. Weinreb, Tetrahedron Lett., 1994, 35, 4287. CrossRef
152. R. C. Larock and J. M. Zenner, J. Org. Chem., 1995, 60, 482. CrossRef
153. P. Pinho, A. J. Minnaard, and B. L. Feringa, Org. Lett., 2003, 5, 259. CrossRef
154. J. Han, Y. Li, S. Zhi, Y. Pan, C. Timmons, and G. Li, Tetrahedron Lett., 2006, 47, 7225. CrossRef
155. A. M. M. Antunes, S. J. L. Marto, P. S. Branco, S. Prabhakar, and A. M. Lob, Chem. Commun., 2001, 405. CrossRef
156. N. Sakai, A. Ridder, and J. F. Hartwig, J. Am. Chem. Soc., 2006, 128, 8134. CrossRef
157. R. Beniazza, J. Dunet, F. Robert, K. Schenk, and Y. Landais, Org. Lett., 2007, 9, 3913. CrossRef
158. K. Muniz, J. Am. Chem. Soc., 2007, 129, 14542. CrossRef
159. (a) J. L. Brice, J. E. Harang, V. I. Timokhim, N. A. Anastasi, and S. S. Stahl, J. Am. Chem. Soc., 2005, 127, 2868; CrossRef (b) For allylic amination under oxidative conditions, see: K. J. Fraunhoffer, and M. C. White, J. Am. Chem. Soc., 2007, 129, 7274. CrossRef
160. A. R. Dick, J. W. Kampf, and M. S. Sanford, J. Am. Chem. Soc., 2005, 127, 12790. CrossRef
161. K. Tsutsumi, S. Ogoshi, S. Nishiguchi, and H. Kurosawa, J. Am. Chem. Soc., 1998, 120, 1938. CrossRef
162. (a) H. F. Schuster and G. M. Coppola, Allenes in Organic Synthesis; John Wiley & Sons: New York, 1984; (b) S. Ma, Chem. Rev., 2005, 105, 2829 and references therein; CrossRef (c) J. D. Winkler and J. R. Ragains, Org. Lett., 2006, 8, 4031; CrossRef (d) B. M. Trost and J. Xie, J. Am. Chem. Soc., 2006, 128, 6044; CrossRef (e) T. Yoshino, F. Ng, and S. J. Danishefsky, J. Am. Chem. Soc., 2006, 128, 14185. CrossRef
163. (a) G. Liu and X. Lu, Org. Lett., 2001, 3, 3879; CrossRef (b) N. Krause, A. Hoffmann-Roder, and J. Canisius, Synthesis, 2002, 1759; CrossRef (c) N. A. Nedolya, N. I. Schlyakhtina, V. P. Zinov’eva, A. I. Albanov, and L. Brandsma, Tetrahedron Lett., 2002, 43, 1569. CrossRef
164. R. Grigg, C. Kilner, E. Mariani, and V. Sridharan, Synlett, 2006, 3021. CrossRef
165. K. R. Roesch, H. Zhang, and R. C. Larock, J. Org. Chem., 2001, 66, 8042. CrossRef
166. B. C. van Esseveldt, J. F. L. van Delft, R. Gelder, and F. P. J. T. Rutjes, Org. Lett., 2003, 5, 1717. CrossRef
167. B. Gabriele, R. Mancuso, G. Salerno, G. Ruffolo, and P. Plastina, J. Org. Chem., 2007, 72, 6873. CrossRef
168. (a) L. M. Lutete, I. Kadota, and Y. Yamamoto, J. Am. Chem. Soc., 2004, 126, 1622; CrossRef (b) N. T. Patil, L. M. Lutete, H. Wu, N. K. Pahadi, I. D. Gridnev, and Y. Yamamoto, J. Org. Chem., 2006, 71, 4270. CrossRef
169. N. T. Patil, H. Zhibao, G. B. Bajracharya, and Y. Yamamoto, J. Org. Chem., 2006, 71, 3612. CrossRef
170. W. Tang and Y. -X. Ding, J. Org. Chem., 2006, 71, 8489. CrossRef
171. I. Ambrogio, S. Cacchi, and G. Fabrizi, Org. Lett., 2006, 8, 2083. CrossRef
172. K. Tsutsumi, S. Ogoshi, S. Nishiguchi, and H. Kurosawa, J. Am. Chem. Soc., 1998, 120, 1938. CrossRef
173. (a) J.-R. Labrosse, P. Lhoste, and D. Sinou, Org. Lett., 2000, 2, 527; CrossRef (b) M. Yoshida, M. Fujita, T. Ishii, and M. Ihara, J. Am. Chem. Soc., 2003, 125, 4874. CrossRef
174. A. Arcadi, S. Cacchi, G. Fabrizi, and L. M. Parisi, Tetrahedron Lett., 2004, 45, 2431. CrossRef
175. Q. Ding, Y. Ye, R. Fan, and J. Wu, J. Org. Chem., 2007, 72, 5439. CrossRef
176. Z. Shen and X. Lu, Tetrahedron, 2006, 62, 10896. CrossRef
177. Q. Huang and R. C. Larock, J. Org. Chem., 2003, 68, 980. CrossRef
178. (a) H. Nakamura, K. Aoyagi, J.-G. Shim, and Y. Yamamoto, J. Am. Chem. Soc., 2001, 123, 372; CrossRef (b) H. Nakamura, J.-G. Shim, and Y. Yamamoto, J. Am. Chem. Soc., 1997, 119, 8113. CrossRef
179. H. Nakamura, H.Iwama, and Y. Yamamoto, J. Am. Chem. Soc., 1996, 118, 6641. CrossRef
180. M. Ohtaka, H. Nakamura, and Y. Yamamoto, Tetrahedron Lett., 2004, 45, 7339. CrossRef
181. H. Nakamura, M. Ohtaka, and Y. Yamamoto, Tetrahedron Lett., 2002, 43, 7631. CrossRef
182. B. Gabriele, G. Salerno, and A. Fazio, J. Org. Chem., 2003, 68, 7853. CrossRef
183. B. Gabriele, G. Salerno, A. Fazio, and L. Veltri, Adv. Synth. Catal., 2006, 348, 2212. CrossRef
184. (a) A. R. Muci and S. L. Buchwald, Top. Curr. Chem., 2002, 219, 131; CrossRef (b) J. P. Wolfe, S. Wagaw, J. F. Marcoux, and S. L. Buchwald, Acc. Chem. Res., 1998, 31, 805. CrossRef
185. (a) H. Siebeneicher, I. Bytschkov, and S. Doye, Angew. Chem., Int. Ed., 2003, 42, 3042; CrossRef (b) C. T. Brain and J. T. Steer, J. Org. Chem., 2003, 68, 6814; CrossRef (c) G. Cuny, M. Bois-Choussy, and J. Zhu, Angew. Chem., Int. Ed., 2003, 42, 4774. CrossRef
186. M. McLaughlin, M. Palucki, and I. W. Davies, Org. Lett., 2006, 8, 3311. CrossRef
187. M. Tietze, A. Iglesias, E. Merisor, J. Conrad, I. Klaiber, and U. Beifuss, Org. Lett., 2005, 7, 1549. CrossRef
188. A. Y. Lebedov, A. S. Khartulyari, and A. Z. Voskoboynikov, J. Org. Chem., 2005, 70, 596. CrossRef
189. M. C. Willis, R. H. Snell, A. J. Fletcher, and R. L. Woodword, Org. Lett., 2006, 8, 5089. CrossRef
190. M. Carril, R. SanMartin, F. Churruca, I. Tellitu, and E. Domınguez, Org. Lett., 2005, 7, 4787. CrossRef
191. M. Carril, R. SanMartin, E. Domınguez, and I. Tellitu, Tetrahedron, 2007, 63, 690. CrossRef
192. R. Omar-Amrani, R. Schneider, and Y. Fort, Synthesis, 2004, 2527. CrossRef
193. F. Bonnaterre, M. Bois-Choussy, and J. Zhu, Org. Lett., 2006, 8, 4351. CrossRef
194. G. Cuny, M. Bois-Choussy, and J. Zhu, J. Am. Chem. Soc., 2004, 126, 14475. CrossRef
195. A. Saleedo, L. Neuville, C. Rondot, P. Retailleau, and J. Zhu, Org. Lett., 2008, 10, 857. CrossRef
196. M. D. Ganton, and M. A. Kerr, Org. Lett., 2005, 7, 4777. CrossRef
197. X.–J. Xu and Y.–X. Zong, Tetrahedron Lett., 2007, 48, 129. CrossRef
198. C. Venkatesh, G. S. M. Sundaram, H. Illa, and H. Junjappa, J. Org. Chem., 2006, 71, 1280. CrossRef
199. A. Kuwahara, K. Nakano, and K. Nozaki, J. Org. Chem., 2005, 70, 413. CrossRef
200. K. Kawaguchi, K. Nakano, and K. Nozaki, J. Org. Chem., 2007, 72, 5119. CrossRef
201. C. S. Cho and D. B. Patel, Tetrahedron, 2006, 62, 6388. CrossRef
202. Y.-Q. Fang and M. Lautens, Org. Lett., 2005, 7, 3549. CrossRef
203. A. Fayol, Y.-Q. Fang, and M. Lautens, Org. Lett., 2006, 8, 4203. CrossRef
204. (a) Top. Organomet. Chem. 2006, Vol. 18, Ed. by M. Beller; (b) S. A. Vizer, K. B. Yerzhanov, A. A. A. A. Quntar, and V. M. Dembitsky, Tetrahedron, 2004, 60, 5499; CrossRef (c) J. Tsuji, Palladium Reagents and Catalysts: Innovation in Organic Synthesis; John Wiley & Sons: Chichester, U.K., 1995.
205. (a) B. Gabriele, G. Salerno, M. Costa, and G. P. Chiusoli, Curr. Org. Chem., 2004, 8, 919; CrossRef (b) G. Vasapollo and G. Mele, Curr. Org. Chem., 2006, 10, 1397; CrossRef (c) D. H. Gibson, Chem. Rev., 1996, 96, 2063. CrossRef
206. S. Ma, B. Wu, and S. Zhao, Org. Lett., 2003, 5, 4429. CrossRef
207. S. Ma, B. Wu, and X. Jiang, J. Org. Chem., 2005, 70, 2588. CrossRef
208. P. Acs, E. Muller, Rangits. T. Lorand, and L. Kollar, Tetrahedron, 2006, 62, 12051. CrossRef
209. (a) J. T. Kuethe, A. Wong, and I. W. Davies, Org. Lett., 2003, 5, 3975; CrossRef (b) S. W. Dantale and B. C. G. Soderberg, Tetrahedron, 2003, 59, 5507; CrossRef (c) F. Ragaini, S. Cenini, D. Brignoli, M. Gasperini, and E. Gallo, J. Org. Chem., 2003, 68, 460. CrossRef
210. I. W. Davies, J. H. Smitrovich, R. Sidler, C. Qu, V. Gresham, and C. Bazaral, Tetrahedron, 2005, 61, 6425. CrossRef
211. F. Ragaini, A. Rapetti, E. Visentin, M. Monzani, A. Caselli, and S. Cenini, J. Org. Chem., 2006, 71, 3748. CrossRef
212. C. Galli, P. Gentili, A. Guarnieri, S. Kobayashi, and Z. Rappoport, J. Org. Chem., 1998, 63, 9292. CrossRef
213. B. Brocklehurst, J. S. Robinson, and D. N. Tawn, Chem. Phys. Lett., 1972, 12, 610. CrossRef
214. R. W. Clawson Jr., R. E. Deavers III, N. G. Akhmedov, and B. C. G. Soderberg, Tetrahedron, 2006, 62, 10829. CrossRef
215. T. L. Scott and B. C. G. Söderberg, Tetrahedron, 2003, 59, 6323. CrossRef
216. T. L. Scott, X. Yu, S. P. Gorugantula, G. Carrero-Martínez, and B. C. G. Söderberg, Tetrahedron, 2006, 62, 10835. CrossRef
217. (a) M. Kitamura and K. Narasaka, Chem. Record, 2002, 2, 268; CrossRef (b) H. Tsutsui, K. Narasaka, and M. Kitamura, Bull. Chem. Soc. Jpn., 2002, 75, 1451; CrossRef (c) S. Zaman, M. Kitamura, and K. Narasaka, Bull. Chem. Soc. Jpn., 2003, 76, 1055. CrossRef
218. (a) S. Zaman, K. Mitsuru, and A. D. Abell, Org. Lett., 2005, 7, 609; CrossRef (b) A. Minatti and K. Muniz, Chem. Soc. Rev., 2007, 36, 1142
. CrossRef
219. (a) G. Battistuzzi, S. Cacchi, G. Fabrizi, Eur. J. Org. Chem., 2002, 58, 2671; CrossRef (b) M. C. Willis, D. Taylor, and A. T. Gillmore, Org. Lett., 2004, 6, 4755; CrossRef (c) R. C. Larock, In Palladium-Catalyzed Annulation of Alkynes; J. Tsuji, Ed.; Topics in Organometallic Chemistry; Spring-Verlag: Berlin, Heidelberg, 2005, 14, 147.
220. (a) K. Sonogashira, In Comprehensive Organic Synthesis; B. M. Trost, and I. Fleming, Eds.; Pergamon: New York,1991, 3, 52; (b) S. Thorand and N. Krause, J. Org. Chem.,1998, 63, 8551; CrossRef (c) T. Konno, J. Chae, T. Ishihara, and H. Yamanaka, Tetrahedron, 2004, 60, 11695; CrossRef (d) M. Shen, G. Li, B. Z. Lu, A. Hossain, F. Roschangar, V. Farina, C. H. Senanayake, Org. Lett., 2004, 6, 4129; CrossRef (e) N. Gathergood and P. J. Scammells, Org. Lett., 2003, 5, 921; CrossRef (f) S. Cacchi, G. Fabrizi, D. Lamba, F. Marinelli, and L. M. Parisi, Synthesis, 2003, 728; CrossRef (g) G. Dai and R. C. Larock, J. Org. Chem., 2003, 68, 920. CrossRef
221. C. R. Hopkins and N. Collar, Tetrahedron Lett., 2004, 45, 8087. CrossRef
222. M. McLaughlin, M. Palucki, and I. W. Davies, Org. Lett., 2006, 8, 3307. CrossRef
223. B. Z. Lu, W. Zhao, H.-X. Wei, M. Dufour, V. Farina, and C. H. Senanayake, Org. Lett., 2006, 8, 3271. CrossRef
224. S. S. Palimkar, P. H. Kumar, R. J. Lahoti, and K. V. Srinivasan, Tetrahedron, 2006, 62, 5109. CrossRef
225. C. R. Hopkins and N. Collar, Tetrahedron Lett., 2005, 46, 1845. CrossRef
226. M. L. Crawley, I. Goljer, D. J. Jenkins, J. F. Mehlmann, L. Nogle, R. Dooley, and P. E. Mahaney, Org. Lett., 2006, 8, 5837. CrossRef
227. (a) L. Ackermann, L. T. Kaspar, and C. J. Gschrei, Org. Lett., 2004, 6, 2515; CrossRef (b) L. Ackermann and R. Born, Tetrahedron Lett., 2004, 45, 9541; CrossRef (c) L. T. Kaspar, B. Fingerhut, and L. Ackermann, Angew. Chem., Int. Ed., 2005, 44, 5972. CrossRef
228. L. Ackermann and A. Althammer, Synlett, 2006, 3125. CrossRef
229. C. Scarborough and S. S. Stahl, Org. Lett., 2006, 8, 3251. CrossRef
230. K.-T. Yip, M. Yang, K.-L. Law, N.-Y. Zhu, and D. Yang, J. Am. Chem. Soc., 2006, 128, 3130. CrossRef
231. B. D. Chapsal, and I. Ojima, Org. Lett., 2006, 8, 1395. CrossRef
232. Y. Jia and J. Zhu, Synlett, 2005, 2469. CrossRef
233. T. G. Back, A. Pandyra, and J. E. Wulff, J. Org. Chem., 2003, 68, 3299. CrossRef
234. K. Cherry, M. Abarbri, J.-L. Parrainb, and A. Duchêne, Tetrahedron Lett., 2003, 44, 5791. CrossRef
235. For a review of the application of azaallyl anions in classic heterocyclic synthesis, see: S. Angelinckx, N. Giubellina, and N. De Kimpe, Chem. Rev., 2004, 104, 2353. CrossRef
236. J. Barluenga, A. Jiménez-Aquino, C. Valdés, and F. Aznar, Angew.Chem., 2007, 46, 1529. CrossRef
237. C. G. Saluste, S. Crumpler, M. Furberb, and R. J. Whitbya, Tetrahedron Lett., 2004, 45, 6995. CrossRef
238. L. Ackermann, and A. Althammer, Angew. Chem., Int. Ed., 2007, 46, 1627. CrossRef
239. C. Jacquelin, N. Saettel, C. Hounsou, and M.-P. Teulade-Fichou,Tetrahedron Lett., 2005, 46, 2589. CrossRef
240. R. Bedford and M. Betham, J. Org. Chem., 2006, 71, 9403. CrossRef
241. (a) R. Ferraccioli, D. Carenzi, E. Motti, and M. Catellani, J. Am. Chem. Soc., 2006, 128, 722; CrossRef (b) T. Okazawa, T. Satoh, M. Miura, and M. Nomura, J. Am. Chem. Soc., 2002, 124, 5286. CrossRef
242. U. Mentzel, D. Tanner, and J. E. Tønder, J. Org. Chem., 2006, 71, 5807. CrossRef
243. F. G. M. Blondel, N. Desban, S. Bouaziz, J.-M. Vierfond, and H. Galons, Tetrahedron. Lett., 2005, 46, 3725. CrossRef
244. (a) C. Hulme and V. Gore, Curr. Med. Chem., 2003, 10, 51; CrossRef (b) R. V. A. Orru and M. de Greef, Synthesis, 2003, 1471; CrossRef (c) J. Zhu, Eur. J. Org. Chem., 2003, 1133. CrossRef
245. (a) L. Weber, K. Illgen, and M. Almstetter, Synlett, 1999, 366; CrossRef (b) D. J. Ramon and M. Yus, Angew. Chem., Int. Ed., 2005, 44, 1602. CrossRef
246. R. Dhawan, R. D. Dghaym, D. J. St. Cyr, and B. A. Arndtsen, Org. Lett., 2006, 8, 3927. CrossRef
247. F. Ye, and H. Alper, Adv. Synth. Catal., 2006, 348, 1855. CrossRef
248. (a) F. Ye and H. Alper, J. Org. Chem., 2007, 72, 3218; CrossRef (b) D. J. Cole-Hamilton, Science, 2003, 299, 1702; CrossRef (c) J. Mo, L. Xu, and J. Xiao, J. Am. Chem. Soc., 2005, 127, 751; CrossRef (d) H. Hagiwara, Y. Sugawara, K. Isobe, T. Hoshi, and T. Suzuki, Org. Lett., 2004, 6, 2325. CrossRef
249. R. Grigg, M. Inman, C. Kilner, I. Köppen, J. Marchbank, R. Selby, and V. Sridharan, Tetrahedron, 2007, 63, 6152. CrossRef
250. B. Clique, S. Vassiliou, N. Monteiro, and G. Balme, Eur. J. Org. Chem., 2002, 1493. CrossRef
251. S. Kamijo, T. Jin, and Y. Yamamoto, Tetrahedron Lett., 2004, 45, 689. CrossRef
252. (a) S. Kamijo and Y. Yamamoto, J. Am. Chem. Soc., 2002, 124, 11940; CrossRef (b) S. Kamijo, T. Jin, and Y. Yamamoto, J. Org. Chem., 2002, 67, 7413. CrossRef
253. M. Umkehrer, C. Kalinski, J. Kolb, and C. Burdack, Tetrahedron Lett., 2006, 47, 2391. CrossRef
254. Y. Kobayashi, H. Kamisaki, R. Yanada, and Y. Takemoto, Org. Lett., 2006, 8, 2711. CrossRef
255. Y. Kobayashi, H. Kamisaki, H. Takeda, Y.Yasui, R. Yanada, and Y. Takemoto, Tetrahedron, 2007, 63, 2978. CrossRef
256. C. Wang, J. A. Tunge, Org. Lett., 2006, 8, 3211. CrossRef
257. H.-B. Zhou and H. Alper, J. Org. Chem., 2003, 68, 3439. CrossRef
258. L. Xu, H. Du, and Y. Shi, J. Org. Chem., 2007, 72, 7038. CrossRef