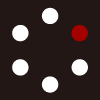
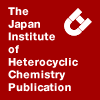
HETEROCYCLES
An International Journal for Reviews and Communications in Heterocyclic ChemistryWeb Edition ISSN: 1881-0942
Published online by The Japan Institute of Heterocyclic Chemistry
e-Journal
Full Text HTML
Received, 23rd December, 2009, Accepted, 18th February, 2010, Published online, 19th February, 2010.
DOI: 10.3987/COM-09-11892
■ A Novel Approach to Diindenocarbazoles via Palladium-Catalyzed Intramolecular Arylation
Lingling Rong, Qiancai Liu,* Jie Tang, and Zhenguo Chi
Department of Chemistry, East China Normal University, 3663 Zhongshan RD(N9, Shanghai 200062, China
Abstract
The diindenocarbazole derivatives are synthesized by the reaction of 2,7-dibromo-9-hexylcarbazole with benzoyl chloride under Friedel-Crafts conditions, followed by Wolff-Kishner-Huang reduction to produce 3,6-dibenzyl-2,7-dibromo-9-hexylcarbazole, which was cyclized to form bis(indeno)-9-hexylcarbazole by palladium-catalyzed double intramolecular arylation. The diindeno-carbazole was further converted into fully alkylated compounds.During the past few decades, organic light-emitting diodes (OLEDs) have attracted considerable interest owing to their promising applications in flat-panel displays.1 The possibility to mass produce efficient displays relies mostly on the ability to explore better functional materials from small organic molecules to polymers. Many efforts have ever been made, however, it was not until recently that the breakthroughs for the OLED technology in small- and medium-size display were obtained. Despite recent achievements, the short lifetime of blue-emitting organic materials as a major problem still remains a challenge.2 Phenylene-based oligomers and polymers might be one of the promising candidates of blue-emitting materials used in organic light-emitting diodes (OLEDs). Among which three major classes of polyphenylene (e.g., polyfluorenes, polyindenofluorenes and ladder-type poly-p-phenylene) have been of particular interests.3 However, reports have shown that the emission of fore-mentioned materials in the solid state is unstable due to the appearance of long-wavelength emission bands from excimer formation and ketone defects.4-5 Many ladder-type oligomers and polymers with significant enhancement in the performance of organic devices were reported by Muellen, Scherf and co-workers.6 Attentions were focused on aromatic diketones such as fluorenone and indenofluorenones and their more conjugated analogues due to their good electron-accepting, transporting abilities as well as photovoltaic utilizations. Some new indenofluorenone and bisindenofluorenone-based molecular synthons have recently been explored and appeared to be a promising family for n-channel semiconducting polymers. But only few examples about ladder-like oligomers with extended π systems incorporating with carbazole especially different bisindenocarbazole (BIC) have been reported so far.7-8 Generally the synthesis of all precursors leading to BICs starting with 2,7-disubstituted carbazoles were made through two efficient routes. In the first method, one or two benzoyl groups are introduced at the 3- and 6-positions of 2,7-dibromo- carbazole by a Friedel-Craft acylation. Tetraphenylene diketones were then obtained by the Suzuki-cross coupling reaction of diketone above with arylboronic acid. Then the diketone was treated with aryllithium to afford diol and followed ring-closure reaction to form new ladder BIC monomer.6a-c The second pathway is quite similar to that described above. The 2,7-dibromocarbazoles with different alkyl chains such as sec-butyl, 2-ethylhexyl, and methyl groups at 9-position were used as starting materials. 2-Acetylphenyl groups are introduced at 2 and 7-positions by Suzuki cross-coupling in excellent yields. The resulting diketones are reduced to a diol quantitatively followed the ring-closure reaction in the presence of a Lewis acid to afford BICs. Only one regiosiomer is found due to the high activities of the 3- and 6-positions of the carbazole. The final step is to introduce different alkyl groups to the BIC core by treatment with BuLi and alkyl halides.10a Furthermore, Sonntag and Strohriegl10b recently described a new BIC derivative containing p-hexylphenyl on both ends which was found to be thermally stable while having excellent electrochemical stability. Further studes were involved a nickel(0)-mediated Yamamoto-type polymerization with dichloro-bisindeno- or dibromo-bisindeno-carbazole precursors to form semi-ladder polymers with a strong blue emission centered at 445 nm. It was also possible to obtain carbazole-based ladder polymers via the polymerization of two different carbazole units, one containing boronic esters while the other bearing benzoyl groups at the 3- and 6-positions ready for further ring-closure. For example, Scherf et al.11 have reported a ladder-type polymer with carbazole moieties while others described new ladder-type conjugated polymer containing carbazole and fluorenyl cores in backbone via intramolecular Friedel-Crafts alkylation.12
Palladium-catalyzed intramolecular arylation to form polyarene especially those with carbo- and heterocycles is now becoming a routinely synthetic strategy. The arylation of arenes catalyzed by palladium is a more direct alternative for the synthesis of biaryls and different heterocycle-fused aromatics, and different compounds with fluorenyl moiety can also be formed from aromatics with o-halogenbenzyl.groups.13-15
Stimulated by research described above, we envisaged that there should be alternative way to prepare BIC monomers. Here we present a new synthetic approach toward new bisindenocarbazoles. Route for the preparation of these materials was designed and completed, which makes it possible to introduce alkyl chains with different lengths to the indenocarbazole core. Scheme 1 shows the synthetic route to the new bis(indeno)carbazoles.
The preparation of 2,7-dibromo-9-hexylcarbazole (3) and 2,7-dibromo-3,6-dibenzoyl-9-hexyl carbazole (4) were completed starting with 4,4’-dibromobiphenyl in a similar manner as reported in literature.9 However, the initial attempts to complete intramolecular arylation of 2,7-dibromo-3,6-dibenzoyl- 9-hexylcarbazole by Pd(OAc)2 in a polar solvents such as DMF or DMAc under different conditions [e.g. with base, a phosphorus ligand and phase transfer catalyst] by following Scherf’s report, all failed.14c Considering palladium-catalyzed arylation of compounds with o-halogen-benzyl moiety can generally lead to the formation of carbo- and heterocylic rings,13-15 therefore we decided to convert 2,7-dibromo-3,6-dibenzoyl)-9-hexylcarbazole (4) into 2,7-dibromo-3,6-dibenzyl-9-hexylcarbazole (5) by Wolff-Kishner-Huang reduction. Then the intramolecular ring-closure reaction of compound 5 to the bisindenocarbazoles (6) was performed with Pd(OAc)2 as catalyst in refluxing DMA for few hours. However, the unstable compound 6 had to be used within a short period. Finally, different alkyl chains can be introduced to the planar bisindenocarbazole (6) with BuLi and alkyl bromides to form compounds 7 and 8. The alkylation of bisindenocarbazoles in the very last step is a big advantage of this synthetic approach, because different alkyl groups can be introduced into bisindenocarbazole core in a satisfactory way. All of the bis(indeno)carbazoles show good solubility in common organic solvents. The structures of the bis(indeno)carbazoles were confirmed by 1H and 13C NMR, elemental analysis as well as mass spectrometry. The UV-vis spectra of compounds 7 and 8 (Figure 1) were measured with maximum absorptions at 358 and 381 nm respectively, while smooth fluorescence emission spectra were obtained with pure blue emission at 428 nm (Figure 2).
In conclusion, we have successfully designed and synthesized the diindeno-carbazole derivatives, which could be used as blue emission materials from primary spectrometric study. The key step of the synthetic strategy involves palladium-catalyzed intramolecular double arylation. Different chains can be introduced to bis(indeno)carbazoles to adjust their properties.
EXPERIMENTAL
Melting point was measured on X-4 micrographic melting point measuring instrument, (Shanghai Precision Scientific Instrument Company). 1H NMR and 13C NMR spectra were measured on a Bruker DR×500 spectrometer (1H NMR 500 MHz, 13C NMR 125 Hz) for all compounds except compound 6, and JEOL ECS-400 Spectrometer (1H NMR 400 MHz, 13C NMR 100.6 MHz) for compound 6, respectively. Element analyses were measured on Vario EL element analysis instrument. UV-vis absorption spectra were measured on VNICAM Helios (carry 50). Molecular fluorescence spectra were measured on HITACHI FL-4500. Mass spectra were measured either on Agilent 5973N mass spectrometer or micOTOF II (ESI) spectrometers.
4,4'-Dibromo-2-nitrobiphenyl (1)
The fuming nitric acid (200 mL) was added slowly to a solution of 4,4'-dibromophenyl (40 g, 0.128 mol) in 600 mL of glacial acetic acid at 100 °C for 1 h, the initially formed precipitate redissolved. The solution was cooled down and the resulting yellow paste was collected by filtration. Recrystallization from EtOH afforded 42.96 g of the title compound as a yellow solid. Mp 128-130 °C (Yield: 90%).
2,7-Dibromocarbazole (2)
A mixture of 1 (20.77 g, 58.2 mmol) and 30 mL of triethyl phosphite was refluxed for 18 h under nitrogen. The excess of triethyl phosphite was distilled off. The crude product was purified by column chromatography (5-20% EtOAc in hexane), and washed with hot hexane to provide 15.30 g of the title compound as a white sold. Mp 230-232 °C (Yield: 42.05%).
2,7-Dibromo-N-hexylcarbazole (3)
To a solution of compound 2 (5 g, 1.5 mmol) in anhydrous DMF (50 mL) was added NaH (0.87 g, 60% w/w dispersion in mineral oil, 21.5 mmol) stepwise. After stirring at room temperature for 0.5 h, 1-bromohexane (3.3 g, 20 mmol) was added dropwise and the solution was stirred for further 13 h under nitrogen. The reaction was carefully quenched with H2O and extracted with CH2Cl2. The organic fraction was dried MgSO4 and the solvent was removed under reduced pressure. The residue was recrystallized from EtOH to afford 5.7 g of 3 as a white solid. Mp 73-76 °C (Yield: 89.30%). 1H NMR (CDCl3): δ 7.849 (d, 2H, J = 1.5Hz), 7.500 (d, 2H, J = 1.5Hz), 7.316 (dd, 2H, J = 1.5Hz, 1.5Hz), 4.143 (t, 2H, J = 7.5Hz), 1.791-1.821 (m, 2H), 1.289-1.371 (m, 6H), 0.873 (t, 3H, J = 7.0Hz). 13C NMR (CDCl3): δ 12.80, 21.34, 25.65, 27.55, 30.29, 42.13, 110.78, 118.47,120.05, 120.25, 121.30, 140.13.
3,6-Dibenzoyl-2,7-dibromo-N-hexylcarbazole (4)
To a mixture of compound 3 (1 g, 2.45 mmol) and AlCl3 (1.97 g, 14.7 mmol) in of CH2Cl2 (20 mL) was added benzoyl chloride (1.05 g, 7.35 mmol) slowly at 0 °C. The mixture was stirred for 4 h at 50 °C, then quenched with ice. The precipitate was dissolved in 2 M NaOH and the product was extracted with CH2Cl2. The organic fraction was dried MgSO4 and the solvent was removed under reduced pressure. Recrystallization from EtOH afforded 1.28 g of the title compound as a white solid. Mp 161-163 °C (Yield: 84.65%). 1H NMR (CDCl3): δ 8.024 (s, 2H), 7.839 (d, 4H, J = 7.7Hz), 7.718 (s, 2H), 7.596 (t, 2H, J = 7.5Hz), 7.462 (t, 4H, J = 7.7Hz), 4.317 (t, 2H, J = 7.3Hz), 1.916-1.945 (m, 2H), 1.340-1.443 (m, 6H), 0.918 (t, 3H, J = 7.0Hz). 13C NMR (CDCl3): δ 13.99, 22.52, 26.83, 28.85, 31.46, 43.79, 114.09, 118.22, 121.00, 122.19, 128.53, 130.34, 131.95, 133.38, 137.14, 142.32, 195.94.
3,6-Dibenzyl-2,7-dibromo-N-hexylcarbazole (5)
The mixture of 3,6-dibenzoyl-2,7-dibromo-N-hexylcarbazole (4) (1 g, 1.6 mmol), KOH (5 g, 89.1 mmol) and N2H4.H2O (50 mL, 85% w/w, 51.5 g) in triethylene glycol (70 mL) was heated to reflux for 7 h. After cooling, the reaction mixture was poured into water and the white precipitate formed was extracted into CH2Cl2. The organic phase was dried over MgSO4, the volatiles were removed by rotory evaporation. The residue was chromatographed on silica gel with eluent (PE:EtOAc = 20:1, V/V) to form a pale-yellow solid (0.89 g, yield 94.7%), recrystallization from petroleum ether afforded analytical pure sample as a white solid. Mp 123-125 °C. 1H NMR (CDCl3): δ 7.803 (s, 2H), 7.616 (s, 2H), 7.321(t, 4H, J = 7.9Hz), 7.241 (t, 6H, J = 7.9Hz), 4.299 (s, 4H), 4.188 (t, 2H, J = 7.3Hz), 1.831-1.875 (m, 2H), 1.350-1.416 (m, 6H), 0.921 (t, 3H, J = 6.9Hz).13C NMR (CDCl3, 500MHz): δ 13.99, 22.53, 26.85, 28.80, 31.49, 41.73, 43.45, 112.93, 121.89, 122.29, 122.53, 126.06, 128.39, 128.83, 130.58, 140.30, 140.44.
Bisindeno[3,2-b:2’,3’-h]-9-hexylcarbazole (6)
A mixture of 5 (500 mg, 0.85 mmol), Pd(OAc)2 (58 mg, 0.256 mmol), Na2CO3 (360 mg, 3.4 mmol), and PhCH2Et3NCl (299 mg, 1.105 mmol) in of DMF (15 mL) was refluxed for 4 h at 140 °C under nitrogen. The mixture was poured into water, and extracted with CH2Cl2. The organic layer was washed with aqueous NaHCO3 and concentrated. The product was chromatographed (12.5% CH2Cl2 in hexane) to provide crude compound (100 mg, yield: 27.5%), which was recrystallized from EtOH to afford analytical pure sample as a white solid. Mp 259-263 °C. 1H NMR (CDCl3): δ 8.21 (s, 2H), 7.92 (d, 2H, J = 4Hz), 7.75 (s, 2H), 7.57 (d, 2H, J = 8Hz), 7.42 (t, 2H, J = 8Hz), 7.31 (t, 2H, J = 8Hz), 4.44 (t, 2H, J = 8Hz), 4.05 (s, 4H), 1.94-2.02 (m, 2H), 1.27-1.54 (m, 6H), 0.89 (t, 3H, J = 6Hz). 13C NMR (CDCl3): δ 14.04, 22.58, 27.04, 28.87, 31.65, 36.36, 43.31, 99.51, 116.07, 119.58, 125.12, 126.47, 126.67, 134.22, 139.85, 141.03, 142.30, 144.25.
1,1,1’,1’-Tetrahexyldisindeno[3,2-b:2’,3’-h]-9-hexylcarbazole (7)
To a mixture of compound 6 (100 mg, 0.234 mmol) and THF (30 mL) was added slowly BuLi (2.5 M, 0.34 mL) at 0 °C under nitrogen. After the color of solution turned to orange, 1-bromohexane (0.10 mL, 0.118g) was added. The solution was stirred at room temperature for 4 h. Again BuLi (2.5 M, 0.34 mL) and 1-bromohexane (0.10 mL, 0.118 g) were added in turn. The solution was stirred for further 12 h and quenched with H2O. The mixture was then extracted with CH2Cl2. The organic fraction was dried MgSO4 and the solvent was removed under reduced pressure. The product was purified by column chromatography (hexanes) to provide crude compound (120 mg, yield: 69.8%), recrystallization from EtOH to afford analytical pure sample as a white solid. Mp 137-139 °C. 1H NMR (CDCl3): δ 8.001 (s, 2H), 7.821 (d, 2H, J = 7.3Hz), 7.645 (s, 2H), 7.362 (t, 4H, J = 7.4Hz), 7.300 (t, 2H, J = 7.9Hz), 4.395 (t, 2H), 2.053-2.107 (m, 8H), 1.980-2.038 (m, 3H), 1.532-1.547 (m, 2H), 1.354-1.438 (m, 5H) 1.004-1.128 (m, 26H), 0.901-0.929 (m, 3H), 0.656-0.748 (m, 16H). 13C NMR (CDCl3): δ 13.96, 14.06, 22.61, 23.82, 29.03, 29.70, 29.84, 31.53, 31.66, 41.27, 54.33, 99.28, 113.87, 119.31, 122.94, 126.59, 126.71, 139.31, 140.99, 141.73, 141.97, 151.54. m/z = 763 (M+).
1,1,1’,1’-Tetra(2-ethylhexyl)disindeno[3,2-b:2’,3’-h]-9-hexylcarbazole (8)
To a mixture of compound 6 (100 mg, 0.234 mmol) and THF (40 mL) was added slowly BuLi (2.5 M, 0.34 mL) at 0 °C under nitrogen. While the solution became orange, 2-ethylhexyl bromide (0.12 mL, 0.131 g) was added. The solution was stirred for 4 h. Then BuLi (2.5M, 0.34 mL) and 2-ethylhexyl bromide (0.12 mL, 0.131 g) were respectively added by the same way. The solution was stirred for 12 h. The reaction was quenched with H2O and extracted with CH2Cl2. The organic fraction was dried MgSO4 and the solvent was removed under reduced pressure. The product was chromatographed with hexanes to provide (130 mg, yield 63.4%). Mp 87-90 °C. 1H NMR (CDCl3): δ 8.000 (t, 2H, J = 4.1Hz), 7.798 (d, 2H, J = 7.7Hz), 7.629 (s, 2H), 7.396 (d, 2H, J = 7.4Hz), 7.336 (t, 2H, J = 7.1Hz), 7.249 (d, 2H, J = 7.4Hz), 4.409 (t, 2H, J = 7.3Hz), 1.971-2.135 (m, 10H), 1.470-1.496 (m, 2H), 1.365-1.410 (m, 2H), 1.302-1.344 (m, 2H), 0.868-0.896 (m, 12H), 0.667-0.802 (m, 34H), 0.446-0.512 (m, 18H). 13C NMR (CDCl3): δ 9.95, 10.05, 10.24, 13.72, 13.82, 13.98, 22.53, 22.60, 22.70, 26.79, 26.85, 26.98, 27.93, 28.06, 31.62, 33.65, 33.69, 34.50, 45.16, 45.28, 54.08, 99.06, 119.14, 124.13, 126.03, 126.55, 141.09, 142.01. Anal. Calcd for C64H93N (875.73): C, 87.71; H, 10.70; N, 1.60. Found: C, 87.46; H, 9.881 N, 1.434. MS (ESI): calcd for C64H93N 875.73; found 875.7313.
ACKNOWLEDGEMENTS
The authors would like to thank Shanghai Natural Science Foundation (09ZR1409400), Laboratory of functional organic molecules, the Sino-French Institute of ECNU, and National Natural Science Foundation of China (No. 50773096) for financial support.
References
1. K. Muellen and U. Scherf, Organic Light-Emitting Devices: Synthesis, Properties and Applications, Wiley-VCH, Weinheim, 2006.
2. B. Geffroy, P. Le Roy, and C. Prat, Polym. Int., 2006, 55, 572. CrossRef
3. a) A. C. Grimsdale and K. Muellen, Macromol. Rapid Commun., 2007, 28, 1676; CrossRef b) U. Scherf, J. Mater. Chem., 1999, 9, 1853. CrossRef
4. V. N. Bliznyuk, S. A. Carter, J. C. Scott, G. Klrner, R. D. Miller, and D. C. Miller, Macromolecules, 1999, 32, 361. CrossRef
5. J.-I. Lee, G. Klaerner, and R. D. Miller, Chem. Mater., 1999, 11, 1083. CrossRef
6. a) L. Romaner, A. Pogantsch, P. Scandiucci de Freitas, U. Scherf, M. Gaal, E. Zojer, and E. J. W. List, Adv. Funct. Mater., 2003, 13, 597; CrossRef b) M. Gaal, E. J. W. List, and U. Scherf, Macromolecules, 2003, 36, 4236; CrossRef c) F. Dierschke, A. C. Grimsdale, and K. Muellen, Macromol. Chem. Phys., 2004, 205, 1147. CrossRef
7. a) U. Scherf and K. Muellen, Macromolecules, 1992, 25, 3546; CrossRef b) J. Jacob, S. Sax, T. Piok, E. J. W. List, A. C. Grimsdale, and K. Muellen, J. Am. Chem. Soc., 2004, 126, 6987; CrossRef c) J. Jacob, S. Sax, M. Gaal, E. J. W. List, A. C. Grimsdale, and K. Muellen, Macromolecules, 2005, 38, 9933; CrossRef d) F. Schindler, J. Jacob, A. C. Grimsdale, U. Scherf, K. Muellen, J. M. Lupton, and J. Feldmann, Angew. Chem., 2005, 117, 1544; CrossRef F. Schindler, J. Jacob, A. C. Grimsdale, U. Scherf, K. Muellen, J. M. Lupton, and J. Feldmann, Angew. Chem. Int. Ed., 2005, 44, 1520; CrossRef e) A. K. Mishra, M. Graf, F. Grasse, J. Jacob, E. J. W. List, and K. Muellen, Chem. Mater., 2006, 18, 2879; CrossRef f) G. Zhou, M. Baumgarten, and K. Muellen, J. Am. Chem. Soc., 2007, 129, 12211. CrossRef
8. a) P. L. T Boudreault, N. Blouin, and M. Leclerc, Adv. Polym. Sci., 2008, 212, 99; CrossRef b) A. Grimsdale and K. Muellen, Adv. Polym. Sci., 2006, 199, 1. CrossRef
9. F. Dierschke, A. Grimsdale, and K. Müllen, Synthesis, 2003, 2470. CrossRef
10. a) M. Sonntag and P. Strohriegl, Tetrahedron, 2006, 62, 8103; CrossRef b) S M. Sonntag and P. Strohriegl, Tetrahedron Lett., 2006, 47, 8313. CrossRef
11. S. A. Patil, U. Scherf, and A. Kadashchuk, Adv. Funct. Mater., 2003, 13, 609. CrossRef
12. B. Wang, F. Shen, P. Lu, S.Tang, W. Zhang, S. Pan, M. Liu, L. Liu, S. Qiu, and Y. Ma, J. Polym. Sci.: Part A: Polym. Chem., 2008, 46, 3120. CrossRef
13. a) J. Hassan, M. Sevignon, C. Gozzi, E. Schulz, and M. Lemaire, Chem. Rev., 2002, 102, 1359; CrossRef b) G. Bringmann, S. Tasler, R.-M. Pfeifer, and M. Breuning, J. Organomet. Chem., 2002, 661, 49; CrossRef c) L.-C. Campeau and K. Fagnou, Chem. Commun., 2006, 1253; CrossRef d) T. Satoh and M. Miura, J. Synth. Org. Chem. Jpn., 2006, 64, 1199.
14. For Palladium-catalyzed o-halobenzyl arenes to form fluorenes, see: a) S. J. Hwang, H. J. Kim, and S. Chang, Org. Lett., 2009, 11, 4588; CrossRef b) L. C. Campeau, M. Parisien, A. Jean, and K. Fagnou, J. Am. Chem. Soc., 2006, 128, 581; CrossRef c) U. Scherf, Makromol. Chem. Rapid Commun. 1993, 14, 575. CrossRef
15. S. Pascual, P. de Mendoza, and A. M. Echavarren, Org. Biomol. Chem., 2007, 5, 2727. CrossRef