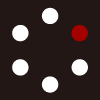
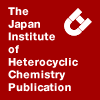
HETEROCYCLES
An International Journal for Reviews and Communications in Heterocyclic ChemistryWeb Edition ISSN: 1881-0942
Published online by The Japan Institute of Heterocyclic Chemistry
e-Journal
Full Text HTML
Received, 25th December, 2009, Accepted, 8th February, 2010, Published online, 9th February, 2010.
DOI: 10.3987/COM-09-11895
■ Peptide Mass Spectrometry by Ionic Probe Attachment Ionization Using NHS-TMpybox and Mal-TMpybox
Fumihiro Ito and Kentaro Yamaguchi*
Faculty of Pharmaceutical Sciences at Kagawa Campus, Tokushima Bunri University, 1314-1 Shido, Sanuki City, Kagawa 769-2193, Japan
Abstract
The application of a new ionization method that uses metal-complex-based ionization probes containing the 2,6-bis(oxazolinyl)pyridine (pybox) ligand for bioactive peptides is presented. Bradykinin, angiotensin-I, angiotensin-II, and nociceptine as well as a couple of pentapeptides were analyzed. This method was proven to effectively ionize large complex molecules, including biomolecules.Mass spectrometry (MS) is used for the precise determination of the molecular masses of peptides and proteins.1 Two newly developed ionization methods, electrospray ionization (ESI)2 and matrix-assisted laser desorption ionization (MALDI),3 have continued to revolutionize the role of MS in biological research. These methods allow the high-precision analysis of very high molecular mass biomolecules. Large biomolecules examined by ESI–MS typically show a distribution of multiply charged molecules that enable detection with a conventional mass spectrometer, such as a quadrupole, an ion trap and magnetic instruments. Charge transfer to a peptide may proceed via mainly protonation. It has been suggested that protonation occurs at the basic residues of the peptide to form positive ions.4 However, problems that hinder the reliable and reproducible ionization of complex large biomolecules by these methods still remain, because of the oft-observed ambiguous protonation during ESI–MS analysis. The protonation seems to be more difficult in the case of cold spray ionization (CSI), a variant of the ESI method and operating under low temperature to ionize labile organic species.5 Therefore, an effective adjustable-charge-state ionization technique6 is required for these molecules. As regards previous studies of instrumental analysis using chemical probes, the ruthenium(II) bisterpyridine complex as an N-terminal labeling reagent of proteins by Ueyama and co-workers7 and the KAP series that enhance ESI efficiency of biomolecules by Suzuki and co-workers8 are known. In particular, the ruthenium(II) bisterpyridine complex has been used in various related research areas.9 Recently, we developed new metal-complex-based ionization probes containing the 2,6-bis(oxazolinyl)pyridine (pybox) ligand, N-hydroxysuccinimide-tetramethylpybox (NHS-TMpybox) 1 and maleimide-tetramethylpybox (Mal-TMpybox) 2, which can ionize target molecules by donating plural charges effectively and reliably, and devised a new method called “ionic probe attachment ionization”.10 In this paper, we describe the application of the charged probes to the ionization of bioactive peptides by CSI–MS.5
The preparation of the charged probe that comprises three functional parts: a charged site, a linker, and an anchoring site, was described in the previous report.10 Two kinds of probes, N-hydroxysuccinimide (NHS) having NHS-TMpybox 1 and maleimide having a Mal-TMpybox 2, were used in this study. La(III) complex 3 was mixed with 1 and 2 to give respective charged probes 4 and 5 in which the metal cation was enclosed by the two pybox ligands to prevent coordination with the counter anion, which would promote ionic dissociation (Scheme 1).11
Two methods to introduce probes 4 and 5 to the target molecule are available, as described in the previous report.10 One involves the addition of a DMSO solution of previously prepared NHS-TMpybox 1 or Mal-TMpybox 2 to the phosphate-buffered standard solution of the target molecule, followed by purification (method A). The other method involves the direct mixing of the MeCN solution of 3 with 1 or 2-binding crude compound that was freeze-dried without further purification (method B). Metal complex 3 was used in both methods to obtain triply charged metal cation probes. Then, the synthesized probes were applied to the CSI-MS measurement of several peptides.
Probe-binding CUSToM peptides
NHS-TMpybox 1 and Mal-TMpybox 2 charged probes in method A ionized two pentapeptides, TTCTT and CTTTC (Figure 1). Single probe attachment to TTCTT yielded a simple doubly charged ion peak with -H+ (Figure 1a), while in the case of TTKTT, the ion included acetylacetonato (acac-) as the counter anion (preciously reported10). Deprotonation occurred in the maleimide groups of Mal-TMpybox or the carboxyl group that had a tendency to release their protons (Scheme 2). This tendency was confirmed by the case of GSH10 that also exhibited doubly charged ion peaks. Then, as expected, two probes were introduced to CTTTC that has two attachment sites, giving quadruply charged ion peaks, respectively (Figure 1b). However, unlike TTCTT, 2-binding CTTTC with acac- as the counter anion was detected.
The charge of the target molecule could be controlled by the number of probes attached. Method B, which is simpler than method A, is thought to be more favorable for the analysis of complex large biomolecules.
PROBE-BINDING BIOACTIVE PEPTIDES
This charged probe method was applied to the ionization of four liner bioactive peptides, angiotensin-I (AT-I), angiotensin-II (AT-II), bradykinin (BK), and nociceptine, via method B. In the case of charged probe 1 binding-BK, triply charged ions ([M]3+: m/z 591, [M + H2O]3+: m/z 597 and [M + acetylacetone]3+: m/z 624) and doubly charged ions ([M + acac-]2+: m/z 936 and [M + acac- + 2H2O]2+: m/z 954) were detected, but plural charged probe attachment was not observed (Figure 2a). Then, similar to BK, in AT series, triply charged ions ([M + acetylacetone + NHS]3+: m/z 658, [M + acetylacetone + NHS + H2O]3+: m/z 664) and doubly charged ions ([M + acac- + NHS]2+: m/z 986, [M + acac- + NHS + H2O]2+: m/z 995) were detected in AT-II (Figure 2b), whereas only triply charged ion ([M + 3acetylacetone + 4H2O]3+: m/z 794) was observed in AT-I (Figure 2c). Finally, nociceptine, which have many attachment sites (two lysines and α-amino group) available, were examined. Quadruply charged ions [M + 2acac- + 3MeCN + 2H2O] (m/z 898) and [M + 2acac- + 3MeCN + 3H2O] (m/z 903) by the attachment of two probes (Figure 2d) were observed in this case. These results confirm that the newly developed charged probes can ionize biomolecules to give reliable corresponding mass spectra.
In conclusion, an effective and reliable ionization method called ionic probe attachment ionization was presented. Bioactive peptides were ionized using these probe attachments. In the ionization of custom pentapeptide CTTTC and nociceptine by means of charged probe attachment, quadruply charged ions produced by the attachment of two probes were observed in the CSI mass spectra. This method reduced the mass-to-charge ratio by donating a charge using the probe, thus enabling the detection of molecular ion in the lower mass region by means of a conventional mass spectrometer.
EXPERIMENTAL
CSI mass spectra were recorded on a JEOL JMS-T100LC mass spectrometer equipped with a cold-spray ion source. This setup made it possible to conduct measurements at low temperatures. Measurement conditions were as follows: sprayer temperature: –20 ~ 20 °C; needle voltage: +1500 ~ 2500 V; ring lens voltage: +5 ~ 10 V; orifice 1 voltage: +10 ~ 50 V; orifice 2 voltage: +5 ~ 10 V; conc. ca. 1 mM.
Introducing the probe to the target molecules
Method A: To a solution (0.5 mL) of the target compound (1 mM in phosphate pH standard solution) was added a solution (0.5 mL) of probe (1 or 2) (1 mM in DMSO) at room temperature. After the mixture was stirred at room temperature for 2 h, it was purified by reversed-phase flash column chromatography (H2O/MeCN = 100 : 0 to 60 : 40). After the target compound was evaporated in vacuo, a solution (0.5 mL) of ligand 3 (1 mM in MeCN) was added. The mixture was stirred at room temperature for 12 h and a part of the mixture was diluted with MeCN and subjected to CSI-MS.
Method B: To a solution (0.5 mL) of the target compound (5 mM in H2O) was added a solution (0.5 mL) of probe (1 or 2) (5 mM in DMSO) at room temperature. After the mixture was stirred at room temperature for 48 h, it was vacuum-freeze dried. A solution (1 mL) of ligand 3 (8 mM in MeCN) was added to the residue and the mixture was stirred at room temperature for 5 h. Then, a part of the mixture was diluted with MeCN and subjected to CSI-MS.
References
1. G. Siuzdak, ‘The Expanding Role of Mass Spectrometry in Biotechnology’; MCC Press: San Diego, 2003.
2. M. Karas, D. Bachmann, and F. Hillenkamp, Anal. Chem., 1985, 57, 2935. CrossRef
3. J. B. Fenn, M. Mann, C. K. Meng, S. F. Wong, and C. M. Whithouse, Science, 1989, 246, 64. CrossRef
4. R. D. Smith, J. A. Loo, C. G. Edmonds, C. J. Barinaga, and H. R. Udseth, Anal. Chem., 1990, 62, 882. CrossRef
5. K. Yamaguchi, J. Mass Spectrom., 2003, 38, 473. CrossRef
6. T. Maki and K. Ishida, J. Org. Chem., 2007, 72, 6427; CrossRef F.-Y. Che and L. D. Fricker, J. Mass Spectrom., 2005, 40, 238; CrossRef H. Mirzaei and F. Regnier, Anal. Chem., 2006, 78, 4175. CrossRef
7. M. Kaneko, R. Masui, K. Ake, Y. Kousumi, S. Kuramitsu, M. Yamaguchi, H. Kuyama, E. Ando, S. Norioka, T. Nakazawa, T. Okamura, H. Yamamoto, and N. Ueyama, J. Proteome Res., 2004, 3, 983; CrossRef A. Ito, T. Okamura, H. Yamamoto, N. Ueyama, M. Yamaguchi, H. Kuyama, E. Ando, S. Tsunasawa, K. Ake, R. Masui, S. Kuramitsu, T. Nakazawa, and S. Norioka, Rapid Commun. Mass Spectrom., 2007, 21, 2647. CrossRef
8. A. Honda, S. Hayashi, H. Hifumi, Y. Honma, N. Tanji, N. Iwasawa, Y. Suzuki, and K. Suzuki, Anal. Sci., 2007, 23, 11. CrossRef
9. J. R. Peterson, T. A. Smith, and P. Thordarson, Chem. Commun., 2007, 1899. CrossRef
10. F. Ito, T. Nakamura, S. Yorita, H. Danjo, and K. Yamaguchi, Tetrahedron Lett., 2009, 50, 6252. CrossRef
11. Although other counter ions including BF4 were tested, PF6 seemed to be favorable for the CSI process based on solvation, because the low coordination ability of this anion might promote dissociation.; See, S. Sakamoto, M. Fujita, K. Kim, and K. Yamaguchi, Tetrahedron, 2000, 56, 955. CrossRef