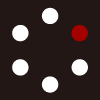
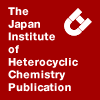
HETEROCYCLES
An International Journal for Reviews and Communications in Heterocyclic ChemistryWeb Edition ISSN: 1881-0942
Published online by The Japan Institute of Heterocyclic Chemistry
e-Journal
Full Text HTML
Received, 9th December, 2009, Accepted, 19th February, 2010, Published online, 22nd February, 2010.
DOI: 10.3987/COM-09-S(E)2
■ Mannich-Type Reaction of N,O-Acetals with Ketones Mediated by a Combination of TiCl4 and PhSiCl3
Satoshi Kamogawa, Takashi Ikeda, Masami Kuriyama, Yoshihiro Matsumura, and Osamu Onomura*
Department of Pharmaceutical Sciences, Graduate School of Biomedical Sciences, Nagasaki University, 1-14 Bunkyo-machi, Nagasaki 852-8521, Japan
Abstract
A combination of TiCl4 and PhSiCl3 efficiently conducts the Mannich-type reaction of N,O-acetals with ketones to afford α-substituted cyclic amine derivatives in good yields. This method was applicable to preparation of azabicyclo compounds by the intramolecular Mannich-type reaction.INTRODUCTION
Lewis acid mediated Mannich-type reaction between N,O-acetals 2 prepared by electrochemical oxidation1 of amine derivatives 1 and carbon nucleophiles (NuH) is one of powerful methods for syntheses of α-substituted amine derivatives 3 (Eq. 1).2,3 We have already reported that some active methylene compounds such as malonates, acetoacetates, and some modified ketones such as enol ethers and enol esters reacted well with 2 as the carbon nucleophiles to form the corresponding α-substituted amine derivatives with high yields.4,5 However, similar reactions between 2 and unmodified ketones did not proceed with good yields. For example, as shown in Eq. 2, although the reaction of N,O-acetal 4 with isopropenyl acetate (5) gave the desired α-acetonylated product 7 in 86% yield, the yield of 7 from acetone (6) was only 23%. We report herein one of the most powerful protocols for TiCl4-mediated Mannich-type reaction of 2 with unmodified ketones promoted by co-existing PhSiCl3.
RESULTS AND DISCUSSION
Since one reason of the low yield of 7 from 6 seemed to be that 6 hardly enolized under the reaction conditions, we tested combinations of TiCl4 and some chlorosilanes6,7 which might accelerate enolization of 6 (Eq. 3). These results are summarized in Table 1. Although in the case of using mono- and di-chlorosilanes the yields of 7 were low (entries 1 and 2), using trichlorosilanes and tetrachlorosilane improved the yields (entries 3-6).
Next, we carried out some experiments to optimize amounts of TiCl4 and PhSiCl3 as a representative combination (Eq. 4), since the yields of 7 promoted by three trichlorosilanes and tetrachlorosilane were almost same (Table 1). The results are shown in Table 2. A combination of a catalytic (0.15 equiv) or an equimolar (1.5 equiv) amount of TiCl4 and an equimolar amount of PhSiCl3 could work well (entries 3 and 6).8 On the other hand, a combination of an equimolar amount of TiCl4 and a catalytic amount of PhSiCl3 did not improve the yield (entry 5).
Similarly this combination of TiCl4 (0.1 equiv) and PhSiCl3 (1.5 equiv) could efficiently mediate the Mannich-type reaction of 4 with acetophenone 8 or cyclopentanone 10 to improve the corresponding products 9 and 119 (Eqs. 5 and 6).
Similar improvement was accomplished in the case of using TiCl2(O-i-Pr)2 as a Lewis acid. Although using only TiCl2(O-i-Pr)2 did not proceed a reaction of 4 with 10, a combination of TiCl2(O-i-Pr)2 and PhSiCl3 could mediate the reaction of 4 with 10 to afford 11 in moderate yield (Eq. 7).
The yields of the reaction of 4 with 10 using a combination of Lewis acid and PhSiCl3 shown in Eqs. 6 and 7 exceeded that of the reaction of 4 with trimethylsilyl enol ether 12 (Eq. 8).
Also, the Mannich-type reaction of N-benzyloxycarbonylated pyrrolidines 13 with 10 was effectively mediated by a combination of TiCl4 and PhSiCl3 to afford the corresponding product 148 (Eq. 9).
Piperidine derivative 15 reacted with 10 in the presence of PhSiCl3 to give the corresponding coupling product 16 with moderate yield (Eq. 10). The diastereomer ratio 66:34 of 16 was almost same as that of 16 obtained from t-butyldimethylsilyl enol ether 17, while that of 16 obtained from 12 was opposite (Eq. 11).10
Furthermore, a combination of TiCl4 and PhSiCl3 effectively mediated the intramolecular Mannich-type reaction of N-methoxycarbonylated pyrrolidines 18a (n=1) and its piperidine analogue 18b (n=2) to form tropinone skeleton 19a and its [3.3.1] analogue 19b which is a precursor for alkaloid and redox-catalysts (Eq. 12).11
The role of the trichlorosilanes and tetrachlorosilane in the present system is not clarified at present. We presume that trichlorosilanes and tetrachlorosilane facilitates smooth enolate generation. Another possibility is that they may facilitate intermediary formations of enol silyl ethers.5,6 However, this speculation could be ruled out, because these species can hardly be generated under the present acidic conditions.
CONCLUSION
In conclusion, a combination of TiCl4 and PhSiCl3 conducts the Mannich type reaction of N,O-acetals with ketones, wherein PhSiCl3 acts as an efficient promoter. Although mechanistic details are still not clear, this reaction is a very promising method in organic synthesis.
EXPERIMENTAL
All commercial materials were used without further purification unless otherwise stated. Analytical thin layer chromatography was performed on Merck silica gel 60 F254 plates (0.25mm). Compounds were visualized by deeping in anisaldehyde followed by heating. Liquid chromatography was performed using indicated solvent on silica gel 60 (200-300 mesh). IR spectra were obtained on a Shimadzu FTIR-8100A. 1H NMR spectra were obtained on Varian Gemini 300 and 400 MHz spectrometer and are reported in parts per million (δ) with tetramethylsilane (TMS) as the internal standard. The coupling constants are recorded in hertz.
A combination of TiC4- and PhSiCl3-mediated Mannich-type reaction: Typical procedure
Under a nitrogen atmosphere, TiCl4 (12 µL, 0.15 mmol) and phenyltrichlorosilane (240 µL, 1.5 mmol) were added dropwise to the solution of 4 (159 mg, 1.0 mmol) and acetone (183 μL, 2.5 mmol) in CH2Cl2 (5 mL) at −78 oC. The resulting mixture was stirred for 12 h and allowed to stand until it warmed to room temperature. The solution was poured in ice water (10 mL) and extracted with CHCl3 (10 mL x 3). The combined organic layer was dried over MgSO4 and the solvent removed under reduced pressure. The residue was purified by silica gel column chromatography (n-hexane : AcOEt = 10 : 1) to afford 7 as a colorless oil (112 mg, 61%). Compounds 7,4 9,4 19a,4 and 19b12 are known.
1-Methoxycarbonyl-2-(2-oxocyclopentyl)pyrrolidine (11)
1H-NMR (300 MHz, CDCl3) δ 1.40-2.50 (m, 10H), 2.70-3.05 (m, 1H), 3.25-3.50 (m, 2H), 3.65 and 3.68 (2s, 3H), 4.10-4.30 (m, 1H); 13C-NMR (100 MHz, CDCl3) δ 218.49 (1C), 155.11 (1C), 56.71, 56.94, 51.82, 51.48, 46.86, 46.54 , 38.42, 34.10, 32.34, 30.33, 27.51, 24.62, 20.25; IR (neat) 2974, 2880, 1747, 1713, 1464, 1396, 1207, 1124, 747 cm−1. Anal. Calcd for C11H17NO3: C, 62.54, H, 8.11; N, 6.63. Found: C, 62.62, H, 8.11; N, 6.37.
1-Benzyloxycarbonyl-2-(2-oxocyclopentyl)pyrrolidine (14)
1H-NMR (300 MHz, CDCl3) δ 1.40-2.40 (m, 11H), 3.30-3.63 (m, 2H), 4.10-4.30 (m, 1H), 5.00-5.18 (m, 2H), 7.20-7.42 (m, 5H); 13C-NMR (100 MHz, CDCl3) δ 218.88, 218.75, 154.82, 154.59, 136.51, 128.23, 66.38, 56.93, 56.12, 51.98, 51.59, 46.79, 46.70, 38.60, 38.26, 30.50, 28.43, 27.69, 25.48, 24.81, 20.36, 20.15; IR (neat) 3374, 3090, 2963, 2880, 1736, 1705, 1597, 1498, 1454, 1419, 1288, 1134 cm−1. HRMS. Calcd for C17H21NO3: 287.1522. Found: 287.1525.
1-Methoxycarbonyl-2-(2-oxocyclopentyl)piperidine (16)
More polar diastereomer (minor isomer in Eq. 10): 1H-NMR (300 MHz, CDCl3) δ 1.30-2.60 (m, 13H), 2.99 (t, J=9.9Hz, 1H), 3.65 and 3.68 (2s, 3H), 3.95-4.30 (m, 1H); 13C-NMR (75 MHz, CDCl3) δ 218.56, 156.93, 52.10, 51.62, 45.19, 39.85, 38.48, 28.16, 26.81, 24.74, 20.39, 18.54; IR (neat) 2947, 2864, 1732, 1693, 1446, 1263, 1180, 767 cm−1. Anal. Calcd for C12H19NO3: C, 63.98, H, 8.50; N, 6.22. Found: C, 64.00, H, 8.46; N, 6.19.
Less polar diastereomer (major isomer in Eq. 10): 1H-NMR (300 MHz, CDCl3) δ 1.30-2.40 (m, 12H), 2.53-2.80 (m, 2H), 3.68 (s, 3H), 3.93-4.40 (m, 2H); 13C-NMR (75 MHz, CDCl3) δ 219.03, 155.76, 52.18, 50.30, 46.81, 39.57, 38.51, 26.61, 26.08, 24.85, 19.78, 18.47; IR (neat) 2939, 2864, 1732, 1693, 1446, 1410, 1263, 1186, 1153, 767 cm−1. Anal. Calcd for C12H19NO3: C, 63.98, H, 8.50; N, 6.22. Found: C, 64.19, H, 8.42; N, 6.33.
ACKNOWLEDGEMENT
This work was supported by the president’s discretion fund of Nagasaki University and Linking mechanism of research results to practical application of Japan Science and Technology Agency.
References
1. T. Shono, H. Hamaguchi, and Y. Matsumura, J. Am. Chem. Soc., 1975, 97, 4264; CrossRef T. Iwasaki, H. Horikawa, K. Matsumoto, and M. Miyoshi, J. Org. Chem., 1979, 44, 1552; CrossRef T. Shono, Y. Matsumura, O. Onomura, M. Ogaki, and K. Kanazawa, J. Org. Chem., 1987, 52, 536; CrossRef G. N. Wanyoike, O. Onomura, T. Maki, and Y. Matsumura, Org. Lett., 2002, 4, 1875; CrossRef S. S. Libendi, Y. Demizu, Y. Matsumura, and O. Onomura, Tetrahedron, 2008, 64, 3935; CrossRef G. N. Wanyoike, Y. Matsumura, and O. Onomura, Heterocycles, 2009, 79, 339. CrossRef
2. Recent representative reviews, see: W. C. Speckamp, and M. J. Moolenaar, Tetrahedron, 2000, 56, 3817; CrossRef A. Yazici, and S. G. Pyne, Synthesis, 2009, 339; CrossRef A. Yazici, and S. G. Pyne, Synthesis, 2009, 513. CrossRef
3. Some literatures, see: S. Yamasaki, T. Iida, and M. Shibasaki, Tetrahedron Lett., 1999, 40, 307; CrossRef G. R. Humphrey, R. A. Miller, P. J. Pye, K. Rossen, R. A. Reamer, A. Maliakal, S. S. Ceglia, E. J. J. Grabowski, R. P. Volante, and P. J. Reider, J. Am. Chem. Soc., 1999, 121, 11261; CrossRef Y. Matsumura, Y. Kanda, K. Shirai, O. Onomura, and T. Maki, Org. Lett., 1999, 1, 175; CrossRef O. Onomura, Y. Kanda, Y. Nakamura, T. Maki, and Y. Matsumura, Tetrahedron Lett., 2002, 43, 3229; CrossRef Y. Matsumura, T. Ikeda, and O. Onomura, Heterocycles, 2006, 67, 113; CrossRef Y. Matsumura, D. Minato, and O. Onomura, J. Organomet. Chem., 2007, 692, 654. CrossRef
4. T. Shono, Y. Matsumura, and K. Tsubata, J. Am. Chem. Soc., 1981, 103, 1172. CrossRef
5. O. Okitsu, R. Suzuki, and S. Kobayashi, J. Org. Chem., 2001, 66, 809; CrossRef O. Onomura, T. Ikeda, and Y. Matsumura, Heterocycles, 2005, 66, 81. CrossRef
6. Me3SiCl promoted aldol reaction, see: Y. Yoshida, N. Matsumoto, R. Hamasaki, and Y. Tanabe, Tetrahedron Lett., 1999, 40, 4227; CrossRef Me2Si(OTf)2 catalyzed aldol reaction, see: S. Kobayashi, and K. Nishio, J. Org. Chem., 1993, 58, 2647; CrossRef Chiral Lewis base catalyzed asymmetric aldol reaction in the presence of SiCl4, see: S. Kotani, Y. Shimoda, M. Sugiura, and M. Nakajima, Tetrahedron Lett., 2009, 50, 4602. CrossRef
7. Chiral Lewis base catalyzed asymmetric reduction of ketones or imines with HSiCl3 reported by us, see: F. Iwasaki, O. Onomura, K. Mishima, T. Maki, and Y. Matsumura, Tetrahedron Lett., 1999, 40, 7507; CrossRef F. Iwasaki, O. Onomura, K. Mishima, T. Kanematsu, T. Maki, and Y. Matsumura, Tetrahedron Lett., 2001, 42, 2525; CrossRef O. Onomura, Y. Kouchi, F. Iwasaki, and Y. Matsumura, Tetrahedron Lett., 2006, 47, 3751; CrossRef Y. Matsumura, K. Ogura, Y. Kouchi, F. Iwasaki, and O. Onomura, Org. Lett., 2006, 17, 3789; CrossRef O. Onomura, P. G. Kirira, T. Tanaka, S. Tsukada, Y. Matsumura, and Y. Demizu, Tetrahedron, 2008, 64, 7498; CrossRef R. Šebesta, M. Mečiarová, E. Molnár, J. Czismadiová, P. Fodran, O. Onomura, and Š. Toma, J. Organomet. Chem., 2008, 693, 3131. CrossRef
8. Although big activation of acetone with a combination of TiCl4 (1.0 equiv) and PhSiCl3 (1.0 equiv) was observed by 1H-NMR analyses shown below, enolization of acetone could not be proved. 1H-NMR (300 MHz, CDCl3) δ 2.18 for acetone; 2.24 for acetone with TiCl4 (1.0 equiv); 2.17 for acetone with PhSiCl3 (1.0 equiv); 2.66 for acetone with a combination of TiCl4 (1.0 equiv) and PhSiCl3 (1.0 equiv).
9. Two diastereomers of 11 were not separable by SiO2 column chromatography. Also, the diastereomer ratio of 11 could not be determined by NMR, HPLC or GC. Similarly, the diastereomer ratio of 14 could not be determined.
10. The diastereomer ratios of 16 shown in Eqs. 10 and 11 might suggest the formation of sterically hindered silyl enol ether from 10 and PhSiCl3.
11. Recent examples, see: U. Albrecht, H. Armbrust, P. Langer, Synlett, 2004, 143; CrossRef Y. Demizu, H. Shiigi, T. Oda, Y. Matsumura, and O. Onomura, Tetrahedron Lett., 2008, 49, 48; CrossRef H. Shiigi, H. Mori, T. Tanaka, Y. Demizu, and O. Onomura, Tetrahedron Lett., 2008, 49, 5247; CrossRef Y. Demizu, H. Shiigi, H. Mori, K. Matsumoto, and O. Onomura, Tetrahedron: Asymmetry, 2008, 19, 2659; CrossRef T. Nagase, T. Takahashi, T. Sasaki, A. Nagumo, K. Shimamura, Y. Miyamoto, H. Kitazawa, M. Kanesaka, R. Yoshimoto, K. Aragane, S. Tokita, and N. Sato, J. Med. Chem., 2009, 52, 4111; CrossRef M. Shibuya, M. Tomizawa, Y. Sasano, and Y. Iwabuchi, J. Org. Chem., 2009, 74, 4619. CrossRef
12. T. Momose, M. Toshima, N. Toyooka, Y. Hirai, and C. H. Eugster, J. Chem. Soc., Perkin Trans. 1, 1997, 1307. CrossRef