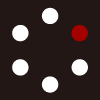
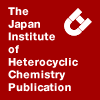
HETEROCYCLES
An International Journal for Reviews and Communications in Heterocyclic ChemistryWeb Edition ISSN: 1881-0942
Published online by The Japan Institute of Heterocyclic Chemistry
e-Journal
Full Text HTML
Received, 19th January, 2010, Accepted, 1st March, 2010, Published online, 2nd March, 2010.
DOI: 10.3987/COM-10-11912
■ Geranyl Derivatives of Isoquinoline Alkaloids Show Increased Biological Activities
Yumi Nishiyama, Kinuko Iwasa,* Suguru Okada, Sousuke Takeuchi, Masataka Moriyasu, Miyoko Kamigauchi, Junko Koyama, Atsuko Takeuchi, Harukuni Tokuda, Hye-Sook Kim, Yusuke Wataya, Kazuyoshi Takeda, Yi-Nan Liu, Pei-Chi Wu, Kenneth F. Bastow, Toshiyuki Akiyama, and Kuo-Hsiung Lee
Kobe Pharmaceutical University, Motoyamakita, Higashinada, Kobe 658-8558, Japan
Abstract
Three types of isoquinoline alkaloids were tested for antimicrobial, cytotoxic, anti-malarial, anti-oxidant, and anti-HIV activities, as well as inhibitory activity against Epstein-Barr virus early antigen (EBV-EA) activation induced by 12-O-tetradecanoylphorbol-13-acetate (TPA) in Raji cells. N- or O-Geranylation contributed to increased potency in four types of activities except anti-HIV and anti-oxidant. Some types of alkaloids may be useful as lead compounds for developing potential chemotherapeutic agents. N,N-Geranylated salsolinol was significantly active in three different assays, antimicrobial, cytotoxic, and EBV-EA, and may be a most useful compound.INTRODUCTION
A number of aromatic prenyltransferases, responsible for prenyl group attachment, have only recently been isolated and characterized.1-4 Aromatic prenyltransferases catalyze the transfer of a C5 (dimethylallyl), C10 (geranyl) or C15 (farnesyl) prenyl group derived from the corresponding isoprenyl diphosphate metabolites onto a variety of electron-rich aromatic acceptors. Prenyl groups appear in a wide variety of bioactive natural products of microbial and plant origin, including amino acids, stilbenes, alkaloids, polyketides and phenylpropanoids such as flavonoids, creating natural product hybrids with altered or enhanced bioactivities. For example, prenylated indole alkaloids containing both aromatic and isoprenoid moieties, are widely distributed in terrestrial and marine organisms.5 Prenylation appears in many cases to provide a higher level of bioactivity compared to the nonprenylated precursors and sometimes to cause biological activities distinct from those of the parent compounds.6,7 Thus, prenylated compounds represent a new frontier for the development of novel drugs, in particular, anti-microbial, anti-oxidant, anti-inflammatory, anti-viral, and anti-cancer agents. We have previously tested the antimicrobial, antimalarial, cytotoxic, anti-HIV, and anti-oxidant activities and inhibitory effects on EBV-EA induction of isoquinoline alkaloids.8,9 Some of the tested isoquinolines showed significant activities in these assays. It was expected that addition of the prenyl group to the isoquinoline alkaloids [simple isoquinolines, 1-benzylisoquinolines, and protoberberines] would increase their potency compared with that of the parent compounds in some assays. In this paper, we describe the synthesis of N- and N,N-geranyl, and O- and O,O-geranyl derivatives of isoquinoline alkaloids and compare their activities with those of non-prenylated compounds in five assays: antimicrobial activity, antimalarial activity, cytotoxicity evaluation,10 inhibitory effects on EBV-EA induction,11 free radical scavenging activity, and anti-HIV activity.12
RESULTS AND DISCUSSIONS
Chemistry. Geranylation of (±)-salsolinol (1)8 with geranyl bromide and sodium hydride in N,N-dimethylformamide has been previously described to give the N- and N,N-geranyl derivatives (2, 3).13 (±)-N-Methylsalsolinol (4) and quaternary (±)-N,N-dimethylsalsolinol (5) has been prepared from (±)-carnegine.13 Geranylation of 5 by the same methods gave the O- and O,O-geranyl derivatives (6-8) (Scheme 1). Geranylation of (±)-tetrahydropapaveroline (9)9 gave the N- and N,N-geranyl derivatives (10 and 11), and (±)-N-methylpapaveroline (12) produced the O,O-geranyl derivative (13) (Scheme 2). Geranylation of (±)-2,3,9,10-tetrademethyltetrahydropalmatine (14)9 afforded the N-geranyl derivatives (15 and 16) and (±)-2,3,10,11-tetrademethylpseudotetrahydropalmatine (17)9 gave the N-geranyl derivative (18) (Scheme 3). Each geranylated compound was purified by preparative HPLC of the reaction mixtures using NH4OAc (0.05% TFA)-MeOH (0.05% TFA).
The HPLC of the reaction mixture in geranylation of salsolinol (1) is shown in Figure 1 (I). The peaks a1 and b1 were determined to be (±)-N-geranylsalsolinol (2) and (±)-N,N-digeranylsalsolinol (3), respectively (Scheme 1). Their structures, including the stereostructure, have been previously described:13 orientation of the C (1)-methyl group is quasi-axial in 2 and 3 and that of the N-geranyl group is quasi-equatorial in 2.
These stereostructures were identical with the optimized geometry of 2 and 3 calculated. The optimized geometry and molecular orbital of 2 and 3 were calculated by the DFT (Density Function Theory) method using the Materials Studio DMol3 package of Accelrys Inc.14,15 (Figure 1) and they may be necessary to clarify the biological activities’ mechanisms.
HPLC of the reaction mixture in geranylation of (±)-N,N-dimethylsalsolinol (5) is shown in Figure 1. Compounds 6-8, corresponding to the peaks a2, b2, and c2, respectively, (Figure 1, II) were separated and purified by preparative HPLC. The molecular formula of compound 6 was determined to be C22H34NO2 by analysis of its HRSIMS ([M]+, m/z 344.2606), which indicates the presence of one geranyl group.
The 1H NMR spectrum displays two aromatic proton singlets at δ 6.68 and 6.74, a methine proton at δ 4.57 (q, J = 6.5 Hz), two methylene groups [δ 3.77 (1H), 3.55 (1H), and 3.08 (2H)], two N-methyl proton signals [δ 3.17 (3H) and 3.14 (3H)], and a methyl proton signal [δ1.69 (3H, d, J = 6.5 Hz)], characteristic of the isoquinoline moiety, and three methyl proton signals at δ 1.75 (3H, s), 1.65 (3H, s), and 1.59 (3H, s), two olefinic protons at δ 5.48 (1H, t, J = 6.5 Hz) and 5.09 (1H, t, J = 6.5 Hz), and three methylene groups [δ 4.62 (2H, d, J = 6.5), δ 2.12 (2H, m), and δ 2.09 (2H, m)], assignable to the geranyl group. The olefinic protons at δ 5.48 and 5.09 show COSY coupling to the methylene protons at δ 4.62 and 2.12, respectively, which led to the assignment of H-2’ (δ 5.48) and H-6’ (δ 5.09), respectively. The methylene protons at δ 4.62 and 2.12 were assigned to H2-1’ and H2-5’, respectively.
The NOE correlations between an aromatic proton at δ 6.74 and a proton at δ 4.57 (H-1) indicated that this aromatic proton was assigned as H-8. The aromatic proton at δ 6.68, which was correlated with the methylene protons at δ 3.08 (H2-4) was assigned as H-5. NOE correlations were also observed between the aromatic proton at δ 6.74 (H-8) and methylene protons at δ 4.62 (H-1’), indicating that a geranyl group was attached to oxygen at C-7. Based on the above evidence, compound 6 was indicated to be 7-O-geranyl-N, N-dimethylsalsolinol (Scheme 1). Moreover, the 13C NMR spectrum of 6 supports this assignment, with signals corresponding to 22 carbons, including two protonated olefinic carbons (δ 124.90; 120.94), two protonated aromatic carbons (δ 116.01; 113.25), six quaternary carbons (δ 148.79; 147.83; 142.30; 132.63; 124.39; 122.17), five methylene carbons (δ 67.06; 56.86; 40.61; 27.41; 24.30), a methine carbon (δ 69.51), two N-methyl carbons (δ 51.50; 50.11) and four methyl carbons (δ 25.84; 18.63; 17.72; 16.69). The carbon signal at δ 147.83 (C-7) displays HMBC correlations with the proton signal at δ 4.62 (H-1’), confirming 7-O-geranylation, which was already suggested by the NOE correlation between H-8 and H-1’. On the basis of these data, the structure of 6 was established to be (±)-7-O-geranyl-N,N-dimethylsalsolinol.
The molecular formula of compound 7 was determined to be C22H34NO2 by analysis of its HRSIMS ([M]+, m/z 344.2603), which indicated the presence of one geranyl group. The 1H NMR spectrum is almost the same with that of 6. The NOE correlations between the aromatic proton at δ 6.66 and δ 4.55 (H-1) and the aromatic proton at δ 6.79 and the methylene protons at δ 3.12 (H2-4) indicate that the aromatic protons at δ 6.66 and 6.79 were assigned to H-8 and H-5, respectively. NOE correlations were also observed between the aromatic proton at δ 6.79 (H-5) and methylene protons at δ 4.63 (H-1’), indicating that a geranyl group is attached to oxygen at C-6. Based on the above evidence, compound 7 was found to be 6-O-geranyl-N,N-dimethylsalsolinol (Scheme 1). Moreover, the 13C NMR spectrum of 7 supports this structure, with signals corresponding to 22 carbons. The carbon signal at δ 148.62 (C-6) display HMBC correlations with the proton signal at δ 4.63 (H-1’), confirming 6-O-geranylation, which was suggested by the NOE correlation between H-5 and H-1’. On the basis of these data, the structure of 7 was confirmed to be (±)-6-O-geranyl-N,N-dimethylsalsolinol.
The molecular formula of compound 8 was determined to be C32H50NO2 by analysis of its HRSIMS ([M]+, m/z 480.3858), which indicated the presence of two geranyl groups. The 1H NMR spectrum displays two aromatic protons as singlets at δ 6.83 and 6.79, a methine proton at δ 4.54 (q, J = 6.5 Hz), two methylene groups [δ 3.79 (1H), 3.57 (1H), and 3.14 (2H)], two N-methyl groups [δ 3.17 (3H) and 3.15 (3H)], and a methyl proton signal [δ 1.69 (3H, d, J = 6.5 Hz)], characteristic of an isoquinoline moiety, and six methyl proton signals at δ 1.66 (6H, s), 1.60 (6H, s), 1.736 (3H, s), and 1.742 (3H, s), four olefinic protons at δ 5.48 (2H) and 5.10 (2H), and six methylene groups [δ 4.58 (2H), 4.59 (2H), 2.12 (4H, m), and δ 2.07 (4H)], assignable to two geranyl groups. The aromatic proton at δ6.83 shows NOE correlations with both methylene protons at δ 3.14 (H-4) and 4.58 (or 4.59, H-1’). This aromatic proton was assigned H-5. Therefore, the geranyl group is attached to the oxygen at C-6. The aromatic proton at δ 6.79 displays NOE correlations with protons at δ 4.54 (H-1), 4.59 (or 4.58, H-1’’), and 1.69 (1-Me) indicating that this aromatic proton was H-8. This indicated that the other geranyl group is attached to the oxygen at C-7. From these data, the structure of 8 was deduced to be 6, 7-O-digeranyl-N,N- dimethylsalsolinol (Scheme 1). The 13C NMR spectrum of 8 supports this structure.
Assignments of 1H and 13C signals of 6-8 were made by 1D and 2D (1H-1H COSY, NOESY, HSQC, and HMBC) spectroscopic data. The geometry of the disubstituted olefinic bonds (between 2’ and 3’ or 2’’ and 3’’) was determined to be E on the basis of the NOE correlation between H-2’ and H-4’or H-2’’ and H-4’’, respectively. The NOE correlations between H-1 and both quasi-equatorial and quasi-axial N-methyl groups indicate the quasi-equatorial orientation of H-1, that is, quasi-axial orientation of the methyl group at C-1. These stereostructures were identical with the optimized geometry of 6-8 calculated.
HPLC of the reaction mixture in geranylation (Scheme 2) of (±)-tetrahydropapaveroline (9) is shown in Figure 1 (III). The compounds corresponding to the peaks a3 and b3 (Figure 1, III) (10 and 11) were purified by prep. HPLC of the reaction mixture. The molecular formula of compound 10 (peak a3) was determined to be C26H34NO4 by analysis of its HRSIMS ([M + H]+, m/z 424.2494), which indicates the presence of one geranyl group. The 1H NMR spectrum displays two aromatic proton singlets at δ 6.65 and 6.18, a methine proton at δ 4.42 (1H, t, J = 6.5 Hz), two methylene groups [δ 3.68 (1H), 3.38 (1H), and 2.97 (2H)] arisen from the isoquinoline moiety, three aromatic protons at δ 6.75 (1H, d, J = 8.5 Hz), 6.50 (1H, brd, J = 8.5 Hz), and 6.58 (1H, brs), methylene protons [δ 3.04 (1H) and 3.14 (1H)] characteristic of a benzyl group, and three methyl proton signals at δ 1.70 (3H, s), 1.64 (3H, s), and 1.57 (3H, s), two olefinic protons at δ 5.26 (1H, m) and 5.12 (1H, m), and three methylene groups [δ 3.83 (1H), δ 3.71 (1H), and δ 2.18 (4H)], assignable to a geranyl group. The NOE correlations between the methylene protons (H-1’’) of the geranyl group and both the methine proton (H-1) of the isoquinoline structure and the methylene protons (H-9) of the benzyl group indicate that the geranyl group was attached to nitrogen. Based on the these evidences, compound 10 was established to be (±)- N-geranyltetrahydropapaveroline (Scheme 2). The 13C NMR spectrum of 10 supports this structure, with signals corresponding to 24 carbons (two carbons overlap), including two protonated olefinic carbons (δ 124.56; 114.15), five protonated aromatic carbons (δ 121.87; 117.67; 116.76; 116.12; 115.75), nine quaternary carbons (δ 149.65; 147.30; 146.92; 146.04; 145.65; 133.29; 121.93; 121.87x2), six methylene carbons (δ 51.86; 44.80; 40.74x2; 27.14; 22.90), a methine carbon (δ 63.01), and three methyl carbons (δ 25.94; 17.84; 16.80). Assignments of 1H and 13C signals of 10 were made by 1D and 2D (1H-1H COSY, NOESY, HSQC, and HMBC) spectroscopic data. The geometry of the disubstituted olefinic bond (between 2’’ and 3’’) was determined to be E on the basis of the NOE correlation between H-2’’ and H-4’’. The geranyl group may be a quasi-equatorial orientation, because a methylene proton (H-1’’) of the geranyl group displayed NOE correlations with both the methine proton (H-1) of the isoquinoline structure and the methylene protons (H-9) of the benzyl group. Therefore, the benzyl group is considered to be quasi-axial orientation. The upfield shift (+ 3.6 ppm) at C-3 in 10 compared with N-methylsalsolinol (4) is interpreted as being due to larger steric compression by the quasi-axial benzyl group at C-1 in 10 than that by the quasi-axial methyl group at C-1 in 4.
The molecular formula of compound 11 (peak b3) was determined to be C36H50NO4 by analysis of its HRSIMS ([M]+, m/z 560.3746), which indicates the presence of two geranyl groups. The 1H NMR and 13C NMR spectra of 11 display the signals due to the 1-benzyltetrahydroisoquinoline structure and two sets of signals due to two geranyl groups. The protons at δ 3.78 (H-1”) and 4.13 (H-1”’) show HMBC correlations with carbons at δ 58.30 (C-1’’’) and 55.79 (C-1”), respectively. Furthermore, HMBC correlations were observed from protons at δ 3.96 (H-1’’) and δ 4.13 (H-1’’’) to the carbon at δ 52.01 (C-3). The proton at δ 4.20 (H-1’’’) also shows a correlation with the carbon at δ 70.72 (C-1). It was concluded that both geranyl groups are attached to nitrogen on the basis of these HMBC correlations, as well as by NOE correlations between H-1 (δ 4.42) and H-1’’ (δ 3.96), H-3 (δ 3.53) and H-1’’’ (δ 4.20), and H-3 (δ 3.68) and H-1’’’ (δ 4.13 and δ 4.20), respectively. Assignments of 1H and 13C signals of 11 were made by 1D and 2D (1H-1H COSY, NOESY, HSQC, and HMBC) spectroscopic data. Based on these evidences, compound 11 was determined to be (±)-N,N-digeranyltetrahydropapaveroline (Scheme 2). The geometries of the disubstituted olefinic bonds (between 2’’ and 3’’ and between 2”’ and 3’’’) were determined to be E on the basis of the NOE correlations between H-2’’ and H-4’’, and H-2”’ and H-4”’, respectively. NOE correlations were observed between the proton at δ 4.20 (H-1’’’) and H2-3 at δ 3.68 and 3.53 (quasi-axial and quasi-equatorial protons), indicating that C-1’’’ of the geranyl group is a quasi-equatorial orientation. The quasi-equatorial orientation of the proton at C-1 was suggested by the NOE correlations between the methine proton at δ 4.42 (H-1) and the proton (δ 3.96) of C-1’’ of the quasi-axial geranyl group. Therefore, the orientation of the benzyl group is quasi-axial. This was suggested from the fact that the upfield shift (+ 4.1 ppm) at C-3 in 11 compared with N, N-dimethylsalsolinol (5) arises from larger steric compression by the quasi-axial benzyl group at C-1 in 11 than that by the quasi-axial methyl group at C-1 in 5.
HPLC of the reaction mixture in geranylation (Scheme 2) of N-methylpapaveroline (12) is shown in Figure 2 (I). Some compounds (peaks a4, b4, and d4) in the reaction mixture changed during prep.
HPLC and were not purified. Only peak c4 was purified by prep. HPLC. The molecular formula of compound 13 (peak c4) was determined to be C37H48NO4 by analysis of its HRSIMS ([M]+, m/z 570.3592), which indicates the presence of two geranyl groups. The 1H NMR spectrum displays two aromatic protons as doublets at δ 8.32 and 8.08 (J = 7.0 Hz), two aromatic protons as singlets at δ 7.70 and 7.60 originating in the isoquinoline moiety, three aromatic protons at δ 6.71 (1H, d, J = 8.5 Hz), 6.36 (1H, dd, J = 8.5, 1.5 Hz), and 6.49 (1H, d, 1.5 Hz), a methylene proton at δ 4.83 (2H) characteristic of a benzyl group, six methyl proton signals at δ 1.83 (3H, s), 1.70 (3H, s), 1.61 (3H, s), 1.59 (3H, s), 1.58 (3H, s), and 1.55 (3H, s), four olefinic protons at δ 5.58 (1H), 5.39 (1H), 5.09 (1H), and 5.02 (1H), and six methylene groups [δ 4.90 (2H), 4.80 (2H), 2.15 (2H), 2.13 (2H), 2.05 (2H), and 1.98 (2H)], assignable to two geranyl groups. The aromatic proton at δ 7.60 shows NOE correlations with both protons at δ 8.08 (H-4) and 4.90 (H-1’’). Thus, the proton at δ 7.60 was assigned to H-5. Therefore, the geranyl group is attached to the oxygen at C-6. The aromatic proton at δ 7.70 displays NOE correlations with protons at δ 4.80 (H-1’’’), indicating that this proton is H-8. This indicates that the other geranyl group is attached to the oxygen at C-7. Based on these evidences, compound 13 was identified as O,O-digeranylpapaveroline (Scheme 2). Moreover, the 13C NMR spectrum of 13 shows four protonated olefinic carbons (δ 124.91; 124.84; 119.65; 119.32), seven protonated aromatic carbons (δ 136.61; 123.58; 120.39; 117.14; 116.13; 108.15; 108.08), 12 quaternary carbons (δ 158.86; 156.93; 154.18; 147.50; 146.17; 144.48; 143.93; 137.50; 132.81; 132.63; 126.47; 125.62), seven methylene carbons (δ 67.71; 67.33; 40.63; 40.46; 34.96; 27.30; 27.29), one N-methyl carbon (δ 46.28), and six methyl carbons (δ 25.91; 25.87; 17.82; 17.81; 16.88; 16.84). The carbon signal at δ 158.86 (C-6) displayed HMBC correlations with the proton signal at δ 4.90 (H-1’’), confirming 6-O-geranylation, which was also suggested by the NOE correlation between H-5 and H-1’’. The carbon signal at δ 154.18 (C-7) displayed HMBC correlations with the proton signal at δ 4.80 (H-1’’’), confirming 7-O-geranylation, which was corroborated by the NOE correlation between H-8 and H-1’’’. Assignments of 1H and 13C signals of 13 were made by 1D and 2D (1H-1H COSY, NOESY, HSQC, and HMBC) spectroscopic data. On the basis of this evidence, the structure of 13 was established to be O, O-digeranylpapaveroline (Scheme 2). The geometries of the disubstituted olefinic bonds (between 2’’ and 3’’ and between 2’’’ and 3’’’) were determined to be E on the basis of the NOE correlation between H-2’’ and H-4’’, and between 2’’’ and 3’’’, respectively.
HPLC of the reaction mixture in geranylation (Scheme 3) of 2, 3, 9, 10-tetrademethyltetrahydropalmatine (14) is shown in Figure 2 (II). The compounds corresponding to peaks a5 and b5 (Figure 2, II) (15 and 16) were purified by prep. HPLC. The molecular formula of compound 15 (peak a5) was determined to be C27H34NO4 by analysis of its HRSIMS ([M]+, m/z 436.2486), which indicates the presence of one geranyl group. The 1H NMR spectrum displays two aromatic proton singlets at δ 6.72 and 6.66, two aromatic proton doublets at δ 6.78 (1H, d, J = 8.0 Hz), 6.57 (1H, d, J = 8.0 Hz), methine and methylene protons at δ 4.58 (3H, brs), three methylene groups [δ 3.81 (1H), 3.54 (1H), 3.28 (1H), 3.18 (2H), 3.12 (1H)] arising from the protoberberine moiety and three methyl proton signals at δ 1.65 (3H, s), 1.59 (3H, s), and 1.58 (3H, s), two olefinic protons at δ 5.44 (1H, t, J = 8.0 Hz) and 5.08 (1H, brs), and three methylene groups [δ 4.08 (2H), 2.22 (2H), and 2.20 (2H)], assignable to the geranyl group. The NOE correlations between a methylene proton (δ 4.08, H-1’) of the geranyl group and the protons at δ 4.58 (H-8 and/or H-13a) indicate that the geranyl group is attached to nitrogen. Based on these evidences, compound 15 was determined to be N-geranyl-2, 3, 9, 10-tetrademethyltetrahydropalmatinium salt. The 13C NMR spectrum of 15 supports this structure, with signals corresponding to 26 (one carbon was overlapped) carbons including four protonated aromatic carbons (δ 119.69; 116.59x2; 114.52), eight quaternary carbons (δ 147.66; 146.25; 144.85; 143.60; 124.76; 121.90; 120.93; 114.80), four methylene carbons (δ 57.06; 51.66; 34.89; 23.75), and a methine carbon (δ 64.34) which arise from the tetrahydroprotoberberine structure, and two protonated olefinic carbons (δ 124.12; 111.74), two quaternary carbons (δ 152.82; 133.47), three methylene carbons (δ 59.93; 41.04; 26.97), three methyl groups (δ 25.78; 17.79; 16.94) assignable to the geranyl group. Assignments of 1H and 13C signals of 15 were made by 1D and 2D (1H-1H COSY, NOESY, HSQC, and HMBC) spectroscopic data. On the basis of these data, the structure of 15 was established to be (±)-N-geranyl-2,3,9,10-tetrademethyl- tetrahydropalmatinium salt with the B/C-cis form as described later (Scheme 3).
The molecular formula of compound 16 (peak b5) was determined to be C27H34NO4 by analysis of its HRSIMS ([M]+, m/z 436.2484), which indicates the presence of one geranyl group. The 1H NMR spectrum displays two aromatic proton singlets at δ 6.84 and 6.71, two aromatic proton doublets at δ 6.85 and 6.77 (J = 8.2 Hz), a methine proton at δ 5.07 (1H, dd, J = 12.5, 5.0 Hz), and four methylene groups [δ 4.72 (1H, d, J = 15.5 Hz), 4.17 (1H, d, J = 15.5 Hz), 3.89 (1H), 3.83 (1H), 3.69 (1H), 3.26 (1H), 3.20 (1H), and 3.05 (1H)] which arise from the tetrahydroprotoberberine structure, and three methyl proton signals at δ 1.72 (3H, s), 1.66 (3H, s), and 1.33 (3H, s), two olefinic protons at δ 5.44 (1H, dd, J = 9.0, 5.5 Hz) and 5.14 (1H, brs), and three methylene groups [δ 3.86 (1H, m), 3.61 (1H, m), and δ 2.20 (4H, m)], assignable to the geranyl group. The NOE correlation between one of the methylene protons (δ 3.86, H-1’) of the geranyl group and one of the methylene protons (δ 4.72, H-8) indicates that the geranyl group is attached to nitrogen. Based on these evidences, compound 16 was postulated to be the isomer of N-geranyl-2,3,9,10-tetrademethyltetrahydropalmatininium salt (15). Moreover, the 13C NMR spectrum of 16 supports this structure, with signals corresponding to 27 carbons, including four protonated aromatic carbons (δ 120.60; 116.84; 116.15; 113.45), eight quaternary carbons (δ 147.37; 146.63, 44.95;
143.66, 122.77; 122.43; 122.06; 115.38), four methylene carbons (δ 58.43; 57.62; 29.60; 24.48), and a methine carbon (δ 68.21) which arise from the tetrahydroprotoberberine structure, and two protonated olefinic carbons (δ 124.71; 114.42), two quaternary carbons (δ 153.83; 133.45), three methylene carbons (δ 46.96; 41.14; 27.10) and three methyl groups (δ 25.97; 17.89; 16.0) assignable to the geranyl group. The proton signals (H-1’) at δ 3.86 and 3.61 display HMBC correlations with the carbons at δ 57.62 (C-8) and δ 58.43 (C-6), respectively, confirming N-geranylation, which was also suggested by the NOE correlation between H-8 and H-1’. Assignments of 1H and 13C signals of 16 were made by 1D and 2D (1H-1H COSY, NOESY, HSQC, and HMBC) spectroscopic data. On the basis of these evidences, the structure of 16 was confirmed to be (±)-N-geranyl-2,3,9,10-tetrademethyltetrahydropalmatininium salt with the B/C-trans form as described below (Scheme 3).
Compounds 15 and 16 are stereoisomers of the B/C ring juncture. The chemical shifts of C-6 in 15 and 16 are 51.66 and 58.43 ppm, respectively. The upfield shift of C-6 in 15 compared with 16 is interpreted as being due to steric compression in the B/C-cis form because C (6)-H and C (13a)-C (13) bonds are 1, 3-diaxial in the B/C-cis form. The chemical shifts at C-13 in 15 and 16 are 34.89 and 29.60 ppm, respectively. The upfield shift of C-13 in 16 compared with 15 arises from steric interaction (1,3-diaxial) between C (13)-H and the N-(C-1’) bonds in the B/C-trans form. Compounds 15 and 16 were established to be (±)-cis- and trans-N-geranyl-2,3,9,10-tetrademethyltetrahydropalmatininium salts (Scheme 3). The geometry of the disubstituted olefinic bond (between 2’ and 3’) was determined to be E in both compounds on the basis of the NOE correlation between H-2’ and H-4’.
The HPLC of the reaction mixture in geranylation (Scheme 3) of 2,3,10,11-tetrademethylpseudo- tetrahydropalmatine (17) is shown in Figure 2 (III). Compound 18, which corresponds to peak b6 (Figure 2, III), was purified by prep. HPLC, although a6 was not purified. The molecular formula of compound 18 was determined to be C27H34NO4 by analysis of its HRSIMS ([M]+, m/z 436.2487), which indicates the presence of one geranyl group. The 1H NMR spectrum displays four aromatic proton singlets at δ 6.83, 6.81, 6.70, 6.55, a methine proton at δ 5.07 and four methylene groups [δ 4.39 (1H), 4.34 (1H), 3.85 (1H), 3.78 (1H), 3.63 (1H), 3.25 (1H), 3.15 (1H), and 3.04 (1H)] which arise from the protoberberine skeleton and three methyl proton signals at δ 1.73 (3H, s), 1.67 (3H, s), and 1.32 (3H, s), two olefinic protons at δ 5.44 (1H) and 5.12 (1H), and three methylene groups [δ 3.87 (1H), 3.64 (1H), and δ 2.20 (4H)], assignable to the geranyl group. From a comparison of the 1H NMR spectrum with that of compounds 15 and 16, compound 18 was postulated to be N-geranyl-2,3,10,11-tetrademethyl- pseudotetrahydropalmatinium salt with a B/C-cis or B/C-trans junction. The 13C NMR spectrum of 18 displays signals corresponding to 27 carbons, including four protonated aromatic carbons (δ 116.26; 116.15; 114.17; 113.46), eight quaternary carbons (δ 147.62; 146.69; 146.63; 147.39; 122.72; 122.53; 122.05; 118.26), four methylene carbons (δ 61.61; 58.27; 29.83; 24.38), a methine carbon (δ 68.55) arising from the tetrahydroprotoberberine structure, and two protonated olefinic carbons (δ 124.86; 111.41), two quaternary carbons (δ 152.91; 133.27), three methylene carbons (δ 46.69; 41.01; 27.04), and three methyl groups (δ 26.0; 17.91; 16.79) assignable to the geranyl group. Compound 18 was identified as (±)-trans-N-geranyl-2,3,10,11-tetrademethylpseudotetrahydropalmatinium salt (Scheme 3) from comparison of the chemical shifts of C-6 (δ 58.27) and C-13a (δ 68.55) with those (δ 51.66 and 64.34) in 15 (B/C-cis) and those (δ 58.43 and 68.21) in 16 (B/C-trans). The geometry of the disubstituted olefinic bond (between 2’ and 3’) was determined to be E on the basis of the NOE correlation between H-2’ and H-4’. Assignments of 1H and 13C signals of 18 were made by 1D and 2D (1H-1H COSY, NOESY, HSQC, and HMBC) spectroscopic data.
Antimicrobial activity.
The synthetic simple isoquinolines [salsolinol (1), N-geranylsalsolinol (2), N,N-digeranylsalsolinol (3), N-methylsalsolinol (4), N,N-dimethylsalsolinol (5), 7-O-geranylsalsolinol (6), 6-O-geranylsalsolinol (7), and 6,7-O-digeranylsalsolinol (8)], the synthetic 1-benzylisoquinolines [tetrahydropapaveroline (9), N-geranyl- and N,N-digeranyltetrahydropapaveroline (10 and 11), N-methylpapaveroline (12), and O, O-digeranyl-N-methylpapaveroline (13)], and the synthetic tetrahydroprotoberberines [2,3,9,10- tetrademethyltetrahydropalmatine (14), cis- and trans-N-geranyl-2,3,9,10-tetrademethyltetrahydro- palmatinium salts (15 and 16), 2,3,10,11-tetrademethylpseudotetrahydropalmatine (17), and trans-N-geranyl-2,3,10,11-tetrademethylpseudotetrahydropalmatininium salt (18)] were tested against Staphylococcus aureus (Gram-positive) and Escherichia coli (Gram-negative) by the liquid dilution method. The minimum inhibitory concentrations (MIC) are presented in Table 1. The results for 1-5 have already been published.13
N-Geranylation increases the activity against both bacteria compared with salsolinol (1), N-methylsalsolinol (4), and N,N-dimethylsalsolinol (5), which are inactive. Notably, N,N-digeranylated salsolinol (3) displays significant activity (7.8 µg/mL) against S. aureus. Thus, according to these data, N-quaternization by N-geranylation (3), not simply N-alkylation (5), appears to be important for enhanced antimicrobial activity.
Enhancement of activity against S. aureus was observed in O-gerany isoquinolines (6-8). Enhanced activity was not observed in N- or O-geranylation of 1-benzylisoquinolines and tetrahydroproto- berberines.
Cytotoxicity evaluation.
The synthetic simple isoquinolines (1-8), 1-benzylisoquinolines (9-13), and tetrahydroprotoberberines (14-18) were assayed for in vitro cytotoxicity against five human tumor cell lines, including lung carcinoma (A-549), prostate carcinoma (DU145), epidermoid carcinoma of the nasopharynx (KB), a drug-resistant KB-subline (KBvin), and human promyelocytic leukemia (HL-60). The cytotoxicity data are given as an ED50 value for each cell line, the concentration of a compound that caused a 50% reduction in absorbance at 562 nm relative to untreated cells using SRB10 and MTT16 and/or WST-8 assays (HL60 is a non-adherent cell line; therefore, the SRB assay could not be used with it), and are shown in Tables 2 and 3. The results for 1-5 have already been published.13
The parent salsolinol (1), N-methylsalsolinol (4), and N,N-dimethylsalsolinol (5) showed no activity in SRB and MTT assays, and N-geranylsalsolinol (2) showed either weak or no activity against all cell lines in both assays. In comparison, N,N-digeranylsalsolinol (3) showed especially increased activity against DU-145, KB, and HL-60 cell lines. Compound 3 exhibited the highest potency (1.2 µg/mL) against the HL-60 cell line, and also displayed high activity (0.77 µg/mL) against this cell line in the WST-8 assay (Table 3).17 Thus, N-geranylation, particularly digeranylation, increased cytotoxicity, while N-methylation, either mono or di, had no effect. The increase in cytotoxicity was found in O- and O,O-digeranyl compounds (6-8) compared with the parent N,N-dimethylsalsolinol (5) in the SRB assay, though activity of O,O-digeranyl compounds (8) was weaker than that of N,N-digeranylsalsolinol (3). Thus, from these data, N-quaternization by N-geranylation, not simply N-alkylation, appears to be important for enhanced cytotoxicity.
In 1-benzyltetrahydroisoquinoline, N- and N,N-digeranylations of tetrahydropapaveroline (9) increased remarkably the activity against three or four cell lines tested in the SRB assay. Compounds 10 and 11 showed a broad spectrum of activity. It was demonstrated that N-geranylation in the 1-benzyltetrahydro- isoquinoline-type alkaloids contributes especially to the increase in cytotoxicity. The increase in cytotoxicity by N,N-digeranylations (11) compared with N-geranylation (10) was not observed to be distinct from that of the simple isoquinolines.
An increase in cytotoxicity was found in the O,O-digeranyl derivative (13) of papaveroline (12), except for the KBvin cell line in SRB and MTT assays. Compound 13 exhibited high potency (1.49 µg/mL) against the HL-60 cell line, and also displayed high activity (1.24 µg/mL) against this cell line in the WST-8 assay.17
In 2,3,9,10-tetrademethyltetrahydropalmatine (14), an increase in cytotoxicity by N-geranylation (15, 16) of the stereostructure was not observed in almost all cell lines, while in the N-geranyl derivative (18) with a B/C-trans junction of 2,3,10,11-tetrademethylpseudotetrahydropalmatine (17), the activity increased, except for the KBvin cell line. Contrary to this, compounds 15 and 16 displayed higher activity than 18 against the HL-60 cell line.
Comparing the activity between the compounds and the cell lines, N- and N,N-geranyl derivatives (10 and 11) of the 1-benzyltetrahydroisoquinolines and N-geranyl compound (16, tetrahydroprotoberberine) with a B/C-trans junction showed high potency (1.31-3.63 µg/mL) in three or four cell lines. The N,N- and N-geranylated compounds (3, simple isoquinoline and 18, tetrahydroprotoberberine) displayed high activity (2.31-3.84 µg/mL) in DU145 and KB cell lines. 2,3,9,10-Tetrademethyltetrahydroproto- berberine (14-16) exhibited high potency (1.33-3.19 µg/mL) in the KBvin cell line, independent of the presence of the geranyl group. In both MTT and WST-8 assays, only two N,N- and O,O-geranylated compounds (3, simple isoquinoline and 13, 1-benzylisoquinoline) displayed high activity in the HL-60 cell line. It was suggested that the structures of the test samples had a strong effect on the HL-60 cell line.
Inhibitory effects on EBV-EA induction.
The Epstein-Barr virus early antigen (EBV-EA) activation assay is considered to be an effective indicator for the evaluation of anti-tumor-promoting activity.11 The inhibitory effects of simple isoquinolines (1-8), 1-benzylisoquinolines (9-13), and tetrahydroprotoberberines (14-18) on EBV-EA activation induced by 12-O-tetradecanoylphorbol-13-acetate (TPA) in Raji cells were examined as a primary screening of anti-tumor-promoting activity.
The inhibitory effects of the test compounds on TPA-induced EBV-EA activation, their effects on the viability of Raji cells, and the 50% inhibitory concentration (IC50) values are shown in Table 4. The results for 1-5 have already been published.13
All simple isoquinolines (1-8) displayed stronger inhibition (IC50 296-384) than that of the reference β-carotene (IC50 400), which has been studied extensively in cancer chemoprevention using animal models.18 The inhibitory activity was more increased by geranylation than by methylation on nitrogen (compared with 3 and 5). Therefore, N-geranylation but not N-dimethylation appears to be important for enhanced activity. N,N-Digeranylsalsolinol (3) displayed the strongest inhibition (IC50 296), and its activity was higher than that of ginsenoside-Rg1, which is known as a strong anti-tumor-promoter.19
The 6,7-O-digeranylated derivative (8) had increased activity (IC50 335) compared with the parent compound (5), though 6- and 7-O-geranylation (6 and 7) scarcely affected the activity. Thus, these compounds, especially N,N- and O,O-digeranylated derivatives, appear to be useful leads for further development of potential cancer chemopreventive agents.
There was little increase in inhibition by N-, and N,N-geranylation (10 and 11) of tetrahydropapaveroline (9). Increased inhibition (IC50 323) was observed in O, O-geranylation (13) of papaveroline (12). All tetrahydroprotoberberines (14-18) displayed strong inhibition (IC50 303-321) comparable to ginsenoside-Rg1 (IC50 310), independent of whether the compound has the geranyl group or not. They might be valuable antitumor promoters. In 2, 3, 9, 10-tetrademethyltetrahydropalmatine (14), there was little increase in inhibition by N-geranylation (15, 16) independent of the stereostructure.
In simple isoquinolines and 1-benzylisoquinolines, digeranylated derivatives (3, 8, and 13) displayed potent activity, suggesting that relative lipophilicity of the geranyl groups may contribute to the inhibitory effect.
Free radical scavenging activity.
Several human illnesses, such as cancer, diabetes, atherosclerosis, etc., can be linked to the damaging action of reactive free radicals.20 The ability of three types of compounds (1–18) to scavenge 1,1-diphenyl-2-picrylhydrazyl (DPPH) free radicals was examined; the results are presented in Table 5. The results for 1-3 have already been published13. To evaluate the free radical scavenging activity of these compounds, the concentration required to scavenge DPPH free radicals by 50% (SC50) was determined.
The antioxidant α-tocopherol was used as a reference compound. Compounds 1-3 displayed similar potency and were more active than α-tocopherol. 6-O- or 7-O-Geranylation (6 or 7) of 5 reduced the activity and 6,7-O-digeranylation (8) further reduced the activity.
All 1-benzyltetrahydroisoquinolines (9-13) are more active than α-tocopherol. N-Geranylation (10, 11) of 1-benzyltetrahydroisoquinoline (9) reduced the activity. The activity also decreased by O,O-digeranylation (13) of papaveroline (12). All tetrahydroprotoberberines (14-18) proved to be more active than α-tocopherol; increased activity by N-geranylation was small.
In all three types of isoquinoline alkaloids, the activity was reduced by N- or O-geranylation. Obviously the phenolic hydroxyl groups on the aromatic rings strongly influenced the activity, without an affect of the geranyl group.
Antimalarial activity.
The simple isoquinolines (1-3), 1-benzylisoquinolines (12, 13), and tetrahydroprotoberberines (14, 16-18) were tested in vitro against human malaria parasite, Plasmodium falciparum FCR-3. The antimalarial activity of each compound was determined as a percentage of reduction compare of control. The compound concentration required to inhibit cell growth by 50% was expressed as EC50. To evaluate the toxicity of the compounds for mammalian cells, the concentration causing a 50% growth reduction (IC50) of mouse mammary FM3A cells, a model of the host, was determined. The IC50/EC50 ratios for the compounds were calculated as an evaluation of antimalarial activity. The results are presented in Table 6. In three types of isoquinoline derivatives, N-geranylation increased the inhibitory activity; however, the geranylated derivatives of the simple isoquinolines and 1-benzylisoquinolines showed only slight inhibitory activity and no selectivity. Only N-geranyl derivatives (16 and 18) of the tetrahydroprotoberberines inhibited P. falciparum with EC50 values in the order of 10-7 M. This is an increase antimalarial activity compared with the parentcompounds (14 and 17), and their selectivity indexes were > 15. Compounds 16 and 18 were potent antimalarial agents with higher selectivity indexes compared with the other test compounds.
In addition, N-geranyl derivatives, 16 and 18, it is noted that the selectivity of the compounds (selectivity; >15) was comparable to that of mefloquine (selectivity; 90). It means that these compounds have safety for human clinical treatment of malaria if these compounds developed new antiamalrial drug.
Anti-HIV activity.
The isoquinoline alkaloids (1-4 and 9-18) were tested against HIV-1 replication in H9 lymphocytes in order to evaluate their anti-HIV activity. However, none of them displayed anti-HIV activity (Table 7). Interestingly, results in the anti-HIV assay did not parallel those in the antimicrobial and cytotoxicity assays.
Conclusions.
In summary, three types of isoquinoline alkaloids (1-18) were tested for antimicrobial, cytotoxic, anti-malarial, anti-oxidant, and anti-HIV activities, as well as inhibitory activity against Epstein-Barr virus early antigen (EBV-EA) activation induced by 12-O-tetradecanoylphorbol-13-acetate (TPA) in Raji cells. N- or O-Geranylation contributed to increased potency in four types of activities except anti-HIV and anti-oxidant. N,N-Geranylation of salsolinol (simple isoquinoline, 1) strongly increased the potency in antimicrobial activity. N,N- and N-Geranylations of tetrahydropapaveroline (1-benzylisoquinoline, 9) strongly enhanced cytotoxic activity. N,N-Geranylation of 1 and N-geranylation of 17 (2,3,10,11- oxygenated protoberberine) increased the same activity to a lesser extent. N,N-Geranylation of 1 and O,O-geranylation of papaveroline (1-benzylisoquinoline, 11) strongly increased cytotoxic activity against the HL-60 cell line. N,N-Geranylsalsolinol (3) showed potent inhibitory effects on EBV-EA induction compared with those of the parent compound (1). O,O-Geranylation of 5 (simple isoquinoline) and N,N-geranylation of 11 (1-benzylisoquinoline) enhanced the inhibitory activity. The protoberberines (14-18) tested also displayed strong inhibitory activity. N-Geranylation of 2,3,9,10- and 2,3,10,11-oxygenated protoberberines increased the antimalarial activity. Among the tested biological activities of the isoquinolines 1-18, N,N-geranylation of salsolinol (1) strongly increased potency in three assays, antimicrobial and cytotoxic activities and inhibitory effects on EBV-EA induction, while N-geranylation increased the same activities to a lesser extent. However, N,N- and N-methylation did not increase the activities in these assays. These simple N-geranylated isoquinolines also have free radical scavenging activity. These findings indicate that the N-geranyl group plays an important role in mediating these biological activities.
Compound 3 shows antimicrobial, cytotoxic, and inhibitory effects on EBV-EA induction, compounds 10, 11, 13, and 18 are cytotoxic, 8, 13, and 14-18 inhibit EBV-EA induction, and 16 and 18 display antimalarial activity. In the present studies, we have identified new biologically active N- or O-geranylated isoquinolines, which may be considered as lead structures for developing potential chemotherapeutic agents. It was first suggested that the addition of a geranyl residue to isoquinoline skeletons may contribute to the enhancement of the biological activities of isoquinoline alkaloids.
EXPERIMENTAL
General procedures. Conventional 1H NMR, NOESY, COSY, HMBC, and HMQC spectra were obtained on a Varian VXR-500 spectrometer (1H: 500 MHz) using CD3OD solvent, except where noted, with TMS as int. standard. 13C NMR and DEPT spectra were measured on a Varian VXR-500 spectrometer (125 MHz). Mass spectra were determined on a Hitachi M-4100 instrument at 75 eV. The secondary ion mass spectra (SIMS) were measured using glycerol as matrix. HPLC and prep. HPLC analyses were performed using a Hitachi M-6200 intelligent pump (1 mL/min) and Hitachi M-6250 or Jasco PU-2089 intelligent pump (6 mL/min), respectively, and a Hitachi L-4000 UV detector (280 nm). Cosmosil 5C18-AR reversed-phase column of small (4.6 i.d. X 150 mm) and large (20 i.d. X 250 mm) sizes were used for HPLC and prep. HPLC, respectively. Analyses with a Hitachi HPLC system were made using a solvent system, (A) 0.1M NH4OAc (0.05% TFA) / (B) MeOH (0.05% TFA) under the following gradient conditions: A/B, initial (75/25), 10 min (50/50), 30 min (20/80) or initial (80/20), 30 min (0/100) or initial (80/20), 20 min (0/100) (flow rate 1 mL/min). Prep. HPLC analyses for purification were performed using a solvent system, (A) H2O (0.05% TFA) / (B) MeOH (0.05% TFA) under the following gradient conditions: A/B 50/50 to 0/100, 30 to 60 min (flow rate 6 mL/min). (±)-Tetrahydropapaverine hydrochloride and azidothymidine (AZT) were purchased (Sigma). (±)-Salsolinol (1),8 (±)-carnegine,9 (±)-N-methylpapaveroline (11),9 (±)-2,3,9,10-tetrademethyl- tetrahydropalmatine (14),9 and (±)-2, 3, 10, 11-tetrademethylpseudotetrahydropalmatine (17)9 have previously been prepared.
Optimized geometry and molecular orbital were calculated by the DFT Method (Density Function Theory) using the Materials Studio DMol3 package of Accelrys Inc.14,15 First, optimized geometry was obtained using the Perdew-Wong LDA functional (PWC)21 and double numerical plus d-functional (DND) basis set. Second, the optimized geometry obtained was further calculated for molecular orbitals using the Becke exchange plus Lee-Young-Parr correlation (BLYP)22, 23 and the double numerical plus polarization (DNP) basis set.
Preparations of (±)-N- and (±)-N,N-geranylsalsolinol (2 and 3). To a stirred suspension of sodium hydride (245 mg, 10.2 mmol) in DMF (15 mL) at room temperature under N2 (±)-salsolinol hydrochloride8 (1, 1 g, 4.65 mmol) was added by portions followed by a catalytic amount of hydroquinone. The mixture was stirred for 30 min. Geranyl bromide (953 mg, 4.39 mmol) was added dropwise and the mixture was stirred for 2 h. NaH (245 mg, 10.2 mmol) and geranyl bromide (953 mg, 4.39 mmol) were added again, and the mixture was stirred for 2 h. After decomposition of excess NaH with MeOH (1 mL), the mixture was poured onto ice-water and extracted with Et2O followed by CHCl3. Et2O and CHCl3 were separately dried and evaporated. The CHCl3 extract was subjected to prep. HPLC [0.1M NH4OAc (0.05% TFA) / MeOH (0.05% TFA) (A/B) initial 60/40, 0/100 (30 min)] to give (±)-N-geranylsalsolinol (2, 205.7 mg, yield 10.3%) and (±)-N,N-geranylsalsolinol (3, 329.9 mg; yield 12.6%) as trifluoroacetate. 2: SIMS m/z [M + H]+ 316; HRMS m/z [M + H]+ 316.2257 (C20H30NO2 requires 316.2271). 3: SIMS m/z [M]+ 452; HRMS m/z [M]+ 452.3527 (C30H46NO2 requires 452.3523). The 1H and 13C NMR spectral data of these compounds have been previously presented.13
Preparation of (±)-N-methylsalsolinol (4). Carnegine-HCl (500 mg, 1.94 mmol) in 47% HBr (2 ml) was refluxed for 2 h at 130° in an oil bath. The solvent was evaporated in vacuo, the residue in MeOH was crystallized to give (±)-N-methylsalsolinol-HBr (4, 398.5 mg, yield 64.8%). SIMS m/z [M + H]+ 194; HRMS m/z [M + H]+ 194.1201 (C11H16NO2 requires 194.1176). The 1H and 13C NMR spectral data of 4 have been previously presented.13
Preparation of (±)-N,N-dimethylsalsolinol (5). A solution of carnegine-HCl (3.1 g, 12.06 mmol) and CH3I (1 ml) in MeOH (25 ml) and (Me)2CO (25 ml) in a glass-stoppered bottle was heated for 75 min at 110 °C in a oil bath. The solvent was evaporated in vacuo, the residue was crystallized in (Me)2CO-Et2O to give N-methylcarnegine iodide (4.75 g, yield 86.5%). The iodide (3.8 g) in 47% HBr (10 ml) was refluxed for 1 h at 140 °C in an oil bath. The solvent was evaporated in vacuo, the residue was crystallized in (Me)2CO-Et2O to give (±)-N,N-dimethylsalsolinol bromide (5, 2.97 g, yield 98.5%). SIMS m/z [M]+ 208; HRMS m/z [M]+ 208.1350 (C12H18NO2 requires 208.1336). The 1H and 13C NMR spectral data of 5 have been previously presented.13
Preparations of (±)-7-O-geranyl-, (±)-6-O-geranyl-, and (±)-6,7-O-digeranyl-N,N-dimethylsalsolinol (6-8). To a stirred suspension of NaH (260 mg, 10.8 mmol) in DMF (15 mL) at rt under N2 (±)-N,N- dimethylsalsolinol hydrobromide (5, 1 g, 3.47 mmol) was added portionwise followed by a catalytic amount of hydroquinone. The mixture was stirred for 30 min. Geranyl bromide (1 g, 4.61 mmol) was added dropwise and the whole mixture was stirred for 3 h. After decomposition of excess NaH with MeOH (1 mL), the mixture was poured onto ice-water and extracted with CHCl3. The combined organic layer was dried and evaporated. The CHCl3 extract was subjected to prep. HPLC [0.1M NH4OAc (0.05% TFA)/MeOH (0.05% TFA) (A/B) initial 100/0, 0/100 (540 min)] to give (±)-7-O-geranyl-N,N-dimethylsalsolinol (6, 102.2 mg, yield 7.7%), (±)-6-O-geranyl-N,N- dimethylsalsolinol (7, 98.8 mg, yield 7.4%) and (±)-6,7-O-digeranyl-N,N-dimethylsalsolinol (8, 154.2 mg, yield 8.9%) as trifluoroacetates. 6: 1H NMR (500 MHz, CD3OD) δ 6.68 (1H, s, H-5), 6.74 (1H, s, H-8), 5.48 (1H, t, J = 6.5 Hz, H-2´), 5.09 (1H, t, J = 6.5 Hz, H-6´), 4.62 (2H, d, J = 6.5 Hz, H-1´), 4.57 (1H, q, J = 6.5 Hz, H-1), 3.77 (1H, dt, J = 12.5, 8.0 Hz, Hax´-3), 3.55 (1H, dt, J = 12.5, 5.0 Hz, Heq´-3), 3.17 (3H, s, ax´NMe), 3.14 (3H, s, eq´NMe), 3.08 (2H, m, H-4), 2.12 (2H, m, H-5´), 2.09 (2H, m, H-4´), 1.75 (3H, s, 3´-Me), 1.69 (3H, d, J = 6.5 Hz, Me-1), 1.65 (3H, s, 8´), 1.59 (3H, s, 7´-Me); 13C NMR (125 MHz, CD3OD) δ 148.79 (C-6), 147.83 (C-7), 142.30 (C-3´), 132.63 (C-7´), 124.90 (C-6´), 124.39 (C-1a), 122.17 (C-4a), 120.94 (C-2´), 116.01 (C-5), 113.25 (C-8), 69.51 (C-1), 67.06 (C-1´), 56.86 (C-3), 51.50 (eq´NMe), 50.11 (ax´NMe), 40.61 (C-4´), 27.41 (C-5´), 25.84 (C-8´), 24.30 (C-4), 18.63 (C-1-Me), 17.72 (7´-Me), 16.69 (3´-Me); SIMS m/z [M]+ 344; HRMS m/z [M + H]+ 344.2606 (C22H34NO2 requires 344.2588), m/z [M-C10H16]+ 208.1355 (C12H18NO2 requires 208.1338) ; 7: 1H NMR (500 MHz, CD3OD) δ 6.79 (1H, s, H-5), 6.66 (1H, s, H-8), 5.50 (1H, m, H-2´), 5.10 (1H, m, H-6´), 4.63 (2H, d, J = 6.5 Hz, H-1´), 4.55 (1H, q, J = 6.5 Hz, H-1), 3.78 (1H, dt, J = 12.5, 7.5 Hz, Hax´-3), 3.56 (1H, dt, J = 12.5, 6.0 Hz, Heq´-3), 3.17 (3H, s, ax´NMe), 3.13 (3H, s, eq´NMe), 3.12 (2H, m, H-4), 2.12 (2H, m, H-5´), 2.07 (2H, m, H-4´), 1.75 (3H, s, 3´-Me), 1.67 (3H, d, J = 6.5 Hz, Me-1), 1.65 (3H, s, Me-8´), 1.60 (3H, s, 7´-Me); 13C NMR (125 MHz, CD3OD) δ 148.62 (C-6), 147.90 (C-7), 142.26 (C-3´), 132.63 (C-7´), 124.90 (C-6´), 124.39 (C-1a), 122.17 (C-4a), 120.94 (C-2´), 116.01 (C-5), 113.25 (C-8), 69.36 (C-1), 66.86 (C-1´), 57.19 (C-3), 51.58 (eq´NMe), 49.80 (ax´NMe), 40.62 (C-4´), 27.39 (C-5´), 25.86 (C-8´), 24.51 (C-4), 18.36 (C-1-Me), 17.74 (7´-Me), 16.67 (3´-Me); SIMS m/z [M]+ 344; HRMS m/z [M + H]+ 344.2603 (C22H34NO2 requires 344.2588), m/z [M-C10H16]+ 208.1317 (C12H18NO2 requires 208.1338); 8: 1H NMR (500 MHz, CD3OD) δ 6.83 (1H, s, H-5), 6.79 (1H, s, H-8), 5.48 (2H, m, H-2´, H-2´´), 5.10 (2H, m, H-6´, H-6´´), 4.59 (2H, m, H-1´´), 4.58 (2H, m, H-1´), 4.54 (1H, q, J = 6.5 Hz, H-1), 3.79 (1H, dt, J = 12.5, 8.0 Hz, Hax´-3), 3.57 (1H, dt, J = 12.5, 5.0 Hz, Heq´-3), 3.17 (3H, s, ax´NMe), 3.15 (3H, s, eq´NMe), 3.14 (2H, m, H-4), 2.12 (4H, m, H-5´, H-5´´), 2.07 (4H, m, H-4´, H-4´´), 1.742 (3H, s, 3´´-Me), 1.736 (3H, s, 3´-Me), 1.69 (3H, d, J = 6.5 Hz, Me-1), 1.66 (6H, s, 8´, 8´´), 1.60 (6H, s, 7´-Me, 7´´-Me); 13C NMR (125 MHz, CD3OD) δ 150.79 (C-6), 149.78 (C-7), 142.58(C-3´´), 142.56 (C-3´), 132.68 (C-7´), 125.09 (C-1a), 124.96 (C-6´), 122.33 (C-4a), 120.94 (C-2´´), 120.85 (C-2´), 115.05 (C-5), 114.41 (C-8), 69.60 (C-1), 67.38 (C-1´), 67.04 (C-1´´), 56.83 (C-3), 51.55 (eq´NMe), 50.25 (ax´NMe), 40.66 (C-4´, C-4´´), 27.48 (C-5´), 27.44 (C-5´´), 25.93 (C-8´), 24.56 (C-4), 18.63 (C-1-Me), 17.81 (7´-Me), 16.77 (3´-Me), 16.74 (3´´-Me); SIMS m/z [M]+ 480; HRMS m/z [M]+ 480.3858 (C32H50NO2 requires 480.3837), m/z [M-C10H16]+ 344.2586 (C22H34NO2 requires 344.2588).
Preparation of (±)-tetrahydropapaveroline (9). A solution of (±)-tetrahydropapaverine hydrochloride (Sigma, 2 g, 5.26 mmol) in 47% HBr (10 ml) was refluxed for 2.5 h. The solvent was evaporated in vacuo. The crystalline product was recrystallized from MeOH to give (±)-tetrahydropapaveroline hydrobromide 9 (1.84 g, yield 95.1%) which was identified by comparing its 1H NMR and HPLC with data of an authentic sample.
Preparations of (±)-N- and (±)-N,N-digeranyltetrahydropapaveroline (10 and 11). To a stirred suspension of NaH (65 mg, 2.71 mmol) in DMF (15 mL) at rt under N2 (±)-tetrahydropapaveroline hydrobromide (9, 1 g, 2.72 mmol) was added portionwise followed by a catalytic amount of hydroquinone. The mixture was stirred for 30 min. Geranyl bromide (590 mg, 2.72 mmol) was added dropwise, and the mixture was stirred for 10 h. Geranyl bromide (295 mg, 1.36 mmol) was added dropwise and the mixture was further stirred for 12 h. After decomposition of excess NaH with MeOH (1 mL), the mixture was poured onto ice-water and extracted with Et2O followed by CHCl3. The Et2O and CHCl3 phases were separately dried and evaporated. The CHCl3 extract was subjected to prep. HPLC [0.1M NH4OAc (0.05% TFA)/MeOH (0.05% TFA) (A/B) initial 80/20, 0/100 (60 min)] to give (±)-N-geranyltetrahydropapaveroline (10, 274 mg, yield 18.7%) and (±)-N,N-digeranyltetrahydro- papaveroline (11, 175 mg, yield 9.5%) as trifluoroacetate. 10: 1H NMR (500 MHz, CD3OD) δ 6.75 (1H, d, J = 8.5 Hz H-5´), 6.65 (1H, s, H-5), 6.58 (1H, brs, H-2´), 6.50 (1H, brd, J = 8.5 Hz, H-6´), 6.18 (1H, s, H-8), 5.26 (1H, m, H-2´´), 5.12 (1H, m, H-6´´), 4.42 (1H, t, J = 6.5 Hz, H-1), 3.83 (1H, dd, J = 13.0, 8.5 Hz, H-1´´), 3.71 (1H, m, H-1´´), 3.68 (1H, m, H-3), 3.38 (1H, m, H-3), 3.14 (1H, m, H-9), 3.04 (1H, m, H-9), 2.97 (2H, m, H-4), 2.18 (4H, s, H-4´´, H-5´´), 2.12 (2H, m, H-5´), 2.07 (2H, m, H-4´), 1.70 (3H, s, Me-8´´), 1.64 (3H, s, 7´´-Me), 1.57 (3H, s, 3´´-Me); 13C NMR (125 MHz, CD3OD) δ 149.65 (C-3´´), 147.30 (C-6), 146.92 (C-3´), 146.04 (C-4´), 145.65 (C-7), 133.29 (C-7´´), 127.63 (C-1´), 124.56 (C-6´´), 121.93 (C-4a), 121.87 (C-1ª, C-6´), 117.67 (C-2´), 116.76 (C-5´), 116.12 (C-5), 115.75 (C-8), 114.15 (C-2´´), 63.01 (C-1), 51.86 (C-1´´), 44.80 (C-3), 40.74 (C-4´´, C-9), 27.14 (C-5´´), 25.94 (C-8´´), 22.90 (C-4), 17.84 (7´´-Me), 16.80 (3´´-Me); SIMS m/z [M + H]+ 424; HRMS m/z [M + H]+ 424.2494 (C26H34NO4 requires 424.2482); 11: 1H NMR (500 MHz, CD3OD) δ 6.67 (1H, d, J = 8.0 Hz H-5´), 6.64 (1H, s, H-5), 6.38 (1H, brs, H-2´), 6.30 (1H, dd, J = 8.0, 2.0 Hz, H-6´), 5.89 (1H, s, H-8), 5.61 (1H, d, J = 7.0 Hz H-2´´´), 5.42 (1H, t, J = 7.0 Hz, H-2´´), 5.15 (1H, m, H-6´´´) 4.99 (1H, m, H-6´´), 4.42 (1H, dd, J = 9.5, 3.0 Hz, H-1), 4.20 (1H, dd, J = 14.5, 7.0 Hz, H-1´´´) 4.13 (1H, dd, J = 14.5, 7.0 Hz, H-1´´´), 3.96 (1H, dd, J = 14.5, 9.0 Hz, H-1´´), 3.78 (1H, dd, J = 14.5, 6.0 Hz, H-1´´), 3.68 (1H, m, Hax´-3), 3.53 (1H, m, Heq´-3), 3.45 (1H, dd, J = 13.5, 3.5 Hz, H-9), 2.88 (1H, dd, J = 13.5, 9.5 Hz, H-9), 2.97 (2H, m, H-4), 2.28 (4H, s, H-4´´´, H-5´´´), 2.18 (4H, s, H-4´´, H-5´´), 2.07 (2H, m, H-4´), 1.85 (3H, s, 3´´´-Me), 1.69 (3H, s, Me-8´´), 1.68 (3H, s, Me-8´´´), 1.65 (3H, s, 7´´´-Me), 1.62 (3H, s, 7´´-Me) , 1.46 (3H, s, 3´´-Me); 13C NMR (125 MHz, CD3OD) δ 151.18 (C-3´´), 150.09 (C-3´´´), 147.52 (C-6), 146.65 (C-3´), 145.82 (C-4´), 145.24 (C-7), 133.37 (C-7´´), 133.32 (C-7´´´), 127.88 (C-1´), 124.70 (C-6´´), 124.65 (C-6´´´), 121.42 (C-4a), 122.52 (C-1a), 122.25 (C-6´), 118.04 (C-2´), 116.51 (C-5´), 115.85 (C-5), 116.81 (C-8), 112.11 (C-2´´, C-2´´´), 70.72 (C-1), 58.30 (C-1´´´), 55.79 (C-1´´), 52.01 (C-3), 41.06 (C-4´´, C-4´´´), 38.32 (C-9), 27.14 (C-5´´´), 27.05 (C-5´´), 25.98 or 25.99 (C-8´´or C-8´´´), 24.0 (C-4), 17.87 or 17.89 (7´´-Me or 7´´´-Me), 17.28 (3´´´-Me), 16.90 (3´´-Me);SIMS m/z [M]+ 560; HRMS m/z [M]+ 560.3746 (C36H50NO4 requires 560.3746), m/z [M-C10H16]+ 424.2498 (C26H34NO2 requires 424.2482).
Preparation of 6,7-O,O-digeranyl-N-methylpapaveroline (13). To a stirred suspension of NaH (115 mg, 4.79 mmol) in DMF (15 mL) at rt under N2 N-methylpapaveroline bromide9 (12, 1 g, 2.66 mmol) was added portionwise followed by a catalytic amount of hydroquinone. The mixture was stirred for 30 min. Geranyl bromide (240 mg, 1.11 mmol) was added dropwise and the mixture was stirred for 10 h. Geranyl bromide (295 mg, 1.36 mmol) and NaH (70 mg, 2.92 mmol) were added dropwise and the mixture was stirred for a further 5 h. The reaction mixture was treated and separated by prep. HPLC as described in preparation of 10 and 11 to give 6,7-O,O-digeranyl-N-methylpapaveroline (13, 31 mg; yield 4.1%) as trifluoroacetate. 13: 1H NMR (500 MHz, CD3OD) δ 8.32 (1H, d, J = 7.0 Hz, H-3), 8.08 (1H, d, J = 7.0 Hz, H-4), 7.70 (1H, s, H-8), 7.60 (1H, s, H-5), 6.71 (1H, d, J = 8.5 Hz, H-5´), 6.49 (1H, d, J = 1.5 Hz, H-2´), 6.36 (1H, dd, J = 8.5, 1.5 Hz, H-6´), 5.58 (1H, t, J = 6.5 Hz, H-2´´), 5.39 (1H, t, J = 6.5 Hz, H-2´´´), 5.09 (1H, m, H-6´´), 5.02 (1H, m, H-6´´´), 4.90 (2H, d, J = 6.5 Hz, H-1´´), 4.83 (2H, s, H-9), 4.80 (2H, d, J = 6.5 Hz, H-1´´´), 4.30 (3H, s, N-Me), 2.15 (H-5´´), 2.13 (4H, s, H-4´´), 2.05 (2H, m, H-5´´´), 1.98 (2H, m, H-4´´´), 1.83 (3H, s, 3´´-Me), 1.70 (3H, s, 3´´´-Me), 1.61 (3H, s, 8´´), 1.59 (3H, s, 8´´´), 1.58 (3H, s, 7´´-Me), 1.55 (3H, s, 7´´´-Me); 13C NMR (125 MHz, CD3OD) δ 158.86 (C-6), 156.93 (C-1), 154.18 (C-7), 147.50 (C-3´), 146.17 (C-4´), 144.48 (C-3´´), 143.93 (C-3´´´), 137.50 (C-4a), 136.61 (C-3), 132.81 (C-7´´), 132.63 (C-7´´´), 126.47 (C-1´), 125.62 (C-1a), 124.91 or 124.84 (C-6´´or C-6´´´), 123.58 (C-4), 120.39 (C-6´), 119.65 (C-2´´´), 119.32 (C-2´´), 117.14 (C-5´), 116.13 (C-2´), 108.15 (C-8), 108.08 (C-5), 67.71 (C-1´´), 67.33 (C-1´´´), 46.28 (N-Me), 40.63 (C-4´´) , 40.46 (C-4´´´), 34.96 (C-9), 27.30 or 27.29 (C-5´´or C-5´´´), 25.91 (C-8´´), 25.87 (C-8´´´), 17.82 or 17.81 (7´´ or 7´´´-Me), 16.88 or 16.84 (3´´ or 3´´´-Me); SIMS m/z [M]+ 570; HRMS m/z [M]+ 570.3592 (C37H48NO4 requires 570.3583).
Preparations of (±)-(cis) and (±)-(trans)-N-geranyl-2,3,9,10-tetrademethyltetrahydropalmatinium salts (15 and 16). To a stirred suspension of NaH (126 mg, 5.25 mmol) in DMF (15 mL) at room temperature under N2 (±)-2,3,9,10-tetrademethyltetrahydropalmatine hydrobromide9 (14, 1 g, 2.63 mmol) was added by portion followed by a catalytic amount of hydroquinone. The mixture was stirred for 30 min. Geranyl bromide (571 mg, 2.38 mmol) was added dropwise and the mixture was stirred for 2.5 h. Geranyl bromide (220 mg, 1.01 mmol) and NaH (50 mg, 2.08 mmol) were added again and the mixture was further stirred for 2.5 h. After decomposition of excess NaH with MeOH (1 mL), the mixture was poured onto ice-water and extracted with Et2O followed by CHCl3. The Et2O and CHCl3 phases were separately dried and evaporated. The CHCl3 extract was subjected to preparative HPLC [0.1M NH4OAc (0.05% TFA)/MeOH (0.05% TFA) (A/B) initial 60/40, 0/100 (30 min)] to give (±)-(cis)-N-geranyl- 2,3,9,10-tetrademethyltetrahydropalmatinium salt (15, 29.5 mg, yield 2.0%) and (±)-(trans)-N-geranyl- 2,3,9,10-tetrademethyltetrahydropalmatinium salt (16, 137.6 mg, yield 9.5%) as trifluoroacetates. 15: 1H NMR (500 MHz, CD3OD) δ 6.78 (1H, d, J = 8.0 Hz, H-11), 6.72 (1H, s, H-4), 6.66 (1H, s, H-1), 6.57 (1H, d, J = 8.0 Hz, H-12), 5.44 (1H, t, J = 8.0 Hz, H-2´), 5.08 (1H, brs, H-6´), 4.58 (3H, brs, H-8, H-13a), 4.08 (2H, m, H-1´), 3.81 (1H, m, H-6), 3.54 (1H, m, H-6), 3.28 (1H, m, H-13), 3.18 (2H, m, H-5), 3.12 (1H, m, H-13), 2.22 (2H, m, H-4´), 2.20 (2H, m, H-5´), 1.65 (3H, s, 3´-Me), 1.59 (3H, s, 8´), 1.58 (3H, s, 7´-Me); 13C NMR (125 MHz, CD3OD) δ 152.82 (C-3´), 147.66 (C-3), 146.25 (C-2), 144.85 (C-10), 143.60 (C-9), 133.47 (C-7´), 124.76 (C-1a), 124.12 (C-6´), 121.90 (C-12a), 120.93 (C-4a), 119.69 (C-12), 116.59 (C-4, C-11), 114.80 (C-8a), 114.52 (C-1), 111.74 (C-2´), 64.34 (C-13a), 59.93 (C-1´), 57.06 (C-8), 51.66 (C-6), 41.04 (C-4´), 34.89 (C-13), 26.97 (C-5´), 25.78 (C-8´), 23.75 (C-5), 17.79 (7´-Me), 16.94 (3´-Me);SIMS m/z [M]+ 436; HRMS m/z [M]+ 436.2486 (C27H34NO4 requires 436.2482), m/z [M-C10H16]+ 300.1230 (C17H18NO4 requires 300.1235); 16: 1H NMR (500 MHz, CD3OD) δ 6.85 (1H, d, J = 8.2 Hz, H-11), 6.84 (1H, s, H-1), 6.77 (1H, d, J = 8.2 Hz, H-12), 6.71 (1H, s, H-4), 5.44 (1H, dd, J = 9.5, 5.5 Hz, H-2´), 5.14 (1H, brs, H-6´), 5.07 (1H, dd, J = 12.5, 5.0 Hz, H-13a), 4.72 (1H, d, J = 15.5 Hz, H-8), 4.17 (1H, d, J = 15.5 Hz, H-8), 3.89 (1H, m, H-6), 3.86 (1H, m, H-1´), 3.83 (1H, m, H-13), 3.69 (1H, m, H-6), 3.61 (1H, m, H-1´), 3.54 (1H, m, H-6), 3.26 (1H, m, H-5), 3.20 (1H, dd, J = 12.5, 5.0 Hz, H-13), 3.05 (1H, brs, H-5), 2.20 (4H, m, H-4´, H-5´), 1.72 (3H, s, 8´), 1.66 (3H, s, 7´-Me), 1.33 (3H, s, 3´-Me); 13C NMR (125 MHz, CD3OD) δ 153.83 (C-3´), 147.37 (C-3), 146.63 (C-2), 144.95 (C-10), 143.66 (C-9), 133.45 (C-7´), 124.71 (C-6´), 122.77 (C-4a), 122.43 (C-12a), 122.06 (C-1a), 120.60 (C-12), 116.84 (C-11), 116.15 (C-4), 115.38 (C-8a), 113.45 (C-1), 111.42 (C-2´), 68.21 (C-13a), 58.43 (C-6), 57.62 (C-8), 46.96 (C-1´), 41.14 (C-4´), 29.60 (C-13), 27.10 (C-5´), 25.97 (C-8´), 24.48 (C-5), 17.79 (7´-Me), 16.0 (3´-Me);SIMS m/z [M]+ 436; HRMS m/z [M]+ 436.2484 (C27H34NO4 requires 436.2482), m/z [M-C10H16]+ 300.1230 (C17H18NO4 requires 300.1235).
Preparation of (±)-(trans)-N-geranyl-2,3,10,11-tetrademethylpseudotetrahydropalmatinium salts (18). To a stirred suspension of NaH (130 mg, 5.42 mmol) in DMF (15 mL) at room temperature under N2 (±)-2, 3, 10, 11-tetrademethylpseudotetrahydropalmatine hydrobromide9 (17, 1 g, 2.63 mmol) was added by portions followed by a catalytic amount of hydroquinone. The mixture was stirred for 30 min. Geranyl bromide (580 mg, 2.67 mmol) was added dropwise and the mixture was stirred for 4.5 h. After decomposition of excess NaH with MeOH (1 mL), the mixture was poured onto ice-water. The precipitated crystals (703 mg) were subjected to prep. HPLC [0.1M NH4OAc (0.05% TFA)/MeOH (0.05% TFA) (A/B) initial 60/40, 0/100 (30 min)] to give (±)-(trans)-N-geranyl-2,3,10,11- tetrademethylpseudotetrahydropalmatinium trifluoroacetate (18; 318.8 mg; yield 21.9%). 18: 1H NMR (500 MHz, CD3OD) δ 6.83 (1H, s, H-1), 6.81 (1H, s, H-12), 6.70 (1H, s, H-4), 6.55 (1H, s, H-9), 5.44 (1H, m, H-2´), 5.12 (1H, brs, H-6´), 5.07 (1H, m, H-13a), 4.39 (1H, d, J = 15.0 Hz, H-8), 4.34 (1H, d, J = 15.0 Hz, H-8), 3.87 (1H, m, H-1´), 3.85 (1H, m, H-6), 3.78 (1H, m, H-13), 3.64 (1H, m, H-1´), 3.63 (1H, m, H-6), 3.25 (1H, m, H-5), 3.15 (1H, d, J = 13.0 Hz, H-13), 3.04 (1H, m, H-5), 2.20 (4H, m, H-4´, H-5´), 1.73 (3H, s, 8´), 1.67 (3H, s, 7´-Me), 1.32 (3H, s, 3´-Me); 13C NMR (125 MHz, CD3OD) δ 152.91 (C-3´), 147.62 (C-11), 147.39 (C-3), 146.69 (C-10), 146.63 (C-2), 133.27 (C-7´), 124.86 (C-6´), 122.72 (C-4a), 122.53 (C-12a), 122.05 (C-1a), 118.26 (C-8a), 116.26 (C-12), 116.15 (C-4), 114.17 (C-9), 113.46 (C-1), 111.41 (C-2´), 68.55 (C-13a), 61.61 (C-8), 58.27 (C-6), 46.69 (C-1´), 41.01 (C-4´), 29.83 (C-13), 27.04 (C-5´), 26.0 (C-8´), 24.38 (C-5), 17.91 (7´-Me), 16.79 (3´-Me); SIMS m/z [M]+ 436; HRMS m/z [M]+ 436.2487 (C27H34NO4 requires 436.2482), m/z [M-C10H16]+ 300.1241 (C17H18NO4 requires 300.1235).
Antimicrobial assay. Antibacterial activities against E. coli (ATCC 25923) and S. aureus (ATCC 25922) were determined by means of the minimum inhibitory concentration (MIC) using the two-fold serial broth dilution test7 in liquid nutrient medium and 24-well microplates. MIC was defined as the lowest concentration of the test substance which did not induce visible growth in comparison with a blank experiment. The substances were dissolved in DMSO (final concentration 2.5%). Dilutions with the test medium furnished concentrations from 3.9-250 µg/mL. Benzalkonium and benzethonium chloride were used as standards. The several 24-well plates in which each well contained an appropriate growth medium with a different concentration of the respective test samples were incubated with the test organism. The 24-well plate was incubated at 37°C for 24 h for bacteria. Bacteria tested were preliminarily cultivated in 3% nutrient broth ('Nissui', Japan) at 37°C. All experiments were run in duplicate or triplicate.
In vitro cytotoxicity assay (SRB assay). Cytotoxicity was evaluated using a standard HTCL assay. The assay was carried out according to the standard SRB assay procedure described by Rubinstein et al.10 Samples were tested first by prescreening against KB at 40, 4, and 0.4 µg/mL for a two-day exposure period. Active compounds that inhibited KB cell growth ≥ 40% relative to control at 4 µg/mL were re-tested in a dose-response study against an HTCL panel. Drug stock solutions were prepared in DMSO, and the final solvent concentration was 2% DMSO (v/v), a concentration without effect on cell replication. The human tumor cell line panel constituted of lung carcinoma (A-549), prostate carcinoma (DU-145), epidermoid carcinoma of the nasopharynx (KB), and KBvin (drug resistant), and human promyelocytic leukemia (HL-60). Cells were cultured at 37°C in RPMI-1640 with 100 µg/mL kanamycin and 10% (v/v) fetal bovine serum in a humidified atmosphere containing 5% CO2. Initial seeding densities varied among the cell lines to ensure a final absorbance reading in control cultures in the range 1-2.5 A562 units. Drug exposure was for 3 days, and the ED50 value (µg/mL), the drug concentration that reduced the absorbance by 50%, was interpolated from dose-response data. Each test was performed in triplicate, and absorbance readings varied no more than 5%. ED50 values determined in independent tests varied no more than 30%.
In vitro cytotoxicity assay (MTT assay). All cell lines were propagated in RPMI-1640 medium supplemented with 10% (v/v) FBS, 100 U/mL penicillin and 100 µg/mL streptomycin at 37°C in a humidified atmosphere of 5% CO2 and 95% air. Cell viability was measured by the MTT [3-(4,5- dimethythiazol-2-yl)-2,5-diphenyltetrazolium bromide] colorimetric method. Cells were seeded at densities of 5,000–10,000 cells/well in 96-well tissue culture plates. On day two, cells were treated with test compounds for another 72 h. After drug treatment, attached cells were incubated with MTT (0.5 mg/mL, 1 h) and subsequently solubilized in DMSO. The absorbancy at 550 nm was then measured using a microplate reader. The ED50 (µg/mL) is the concentration of agent that reduced cell viability by 50% under the experimental conditions.
In vitro cytotoxicity assay (WST-8 assay). Cell Cultures. Human promyelocytic leukemia HL-60 cells were purchased from Dainippon Pharmaceutical Co., Ltd., and maintained in RPMI-1640 medium (Sigma), supplemented with 10% heat-inactivated fetal bovine serum (Sigma) and 2 mM L-glutamine (Sigma) at 37 °C in a humidified atmosphere containing 5% CO2. The doubling time of cells was approximately 24 h. WST-8 Assay.17 The test compounds were dissolved in DMSO at 50 mM and stored at -20°C. These stock solutions were further diluted with medium from 50 to 0.6 µM prior to use. The final concentrations of DMSO in the culture medium were less than 0.1%. 0.1% DMSO-treated cells were used as the control for all experiments. Cytotoxic effects of test compounds on HL-60 cells were detected by the WST-8 assay. HL-60 cells were plated at 5 × 103 cells/90 µL medium/well into 96-well plates. After overnight growth, cells were treated with various concentrations of test samples for 48 h. Following incubation with test compounds, cell viability was assayed with a Cell Counting Kit-8 (Dojindo Molecular Technologies). Ten microliters of WST-8 solution (5 mM) was added to each well and then incubated for 3 h. The relative viability of cells was determined by measuring the absorbance at 450 nm (reference at 650 nm) with a micro plate reader Anthos Lucy 2 (Aloka Co., Ltd.,). The IC50 (µg/mL) is the concentration of agent that reduced cell viability by 50% under the experimental conditions.
In vitro EBV-EA activation experiment.24 The inhibition of EBV-EA activation was assayed using Raji cells (virus non-producer) which were cultivated in 8% FBS RPMI 1640 medium. The indicator Raji cells (1×106 cells/ml) were incubated at 37 °C for 48 h in 1 ml of the medium containing n-butyric acid (4 mM, inducer), 32 pmol of TPA (20 ng/ml in DMSO), and 32, 16, 3.2, and 0.32 nmol of the test compound (DMSO solution). Smears were made from the cell suspension. The activated cells were stained by high-titer EBV-EA positive sera from nasopharyngeal carcinoma (NPC) patients and detected by a conventional indirect immunofluorescence technique. In each assay, at least 500 cells were counted, and the experiments were repeated three times. The average extent of EA induction was compared with that of positive control experiments with n-butyric acid (4 mM) plus TPA (32 pmol) in which EA induction was ordinarily around 40%. In this screening method, the cell viability required for the judgment of inhibitory effects was more than 60%.
Determination of the scavenging effect on DPPH radicals.25 Ethanol (100 µl) was added to individual wells of a 96-well plate. The test compounds were dissolved in DMSO and diluted with EtOH to adjust to 500 µM concentration. The final solvent concentration was 0.25% DMSO (v/v). The sample solution (100 µl) was added to individuals by the 2-fold dilution, and EtOH solution (100 µl) of DPPH radical (final concentration was 100 µM) was also added. The final concentration of the test compounds was from 0.24 to 250 µM. A control sample containing EtOH solution (100 µl) of DPPH radical and EtOH (100 µl) was prepared in the 96-well plate, which was incubated at 25 °C for 30 min in the dark. After incubation, the decrease in absorbance was determined by measuring the optical density change at 550 nm with a microplate luminescence reader Lucy 2 (ALOKA). The radical-scavenging activity expressed as % inhibition against DPPH radical, was calculated according to Yen and Duh (1994): % Inhibition = [(AB-AA)/AB]×100, (AA is the absorbance of the tested sample after 30 min; AB is the absorbance of the blank sample). The data presented are the average from two or three independent experiments.
In vitro antimalaria screening. Parasites. In all of the studies described in this report, P. falciparum strain FCR-3(ATCC 30932) was used.26, 27 Human serum and erythrocytes were obtained from healthy donors, stored at 4°C and used within 10-14 days from donation. Parasites were cultured in 10% heat inactivated A+ human erythrocytes and suspended at a 5% hematocrit in RPMI 1640 medium (Gibco, NY) which contained 50 mg of gentamicin per liter, and 10% group A+ human serum and was buffered with 25 mM N-2-hydroxyethylpiperazine-N'-2-ethansulfonic acid (HEPES, pH 7.4) and 25 mM NaHCO3. Cultures were maintained at 37 °C in a gas mixture of 5% O2-5% CO2-90% nitrogen.28 Drug testing. Various concentrations of compounds, suspended in 10 µl of distilled water, were added to individual wells of a 24-well plate. Erythrocytes with 0.3% parasitemia were added to each well in 990 µl of culture medium to give a final hematocrit of 3%. The plates were incubated at 37 °C for 72 h under 5% O2-5% CO2-90% nitrogen. Parasite morphology in drug-treated culture after 72 hrs was measured by staining with Giemsa, and the number of parasitized red blood cells per 10,000 erythrocytes was counted and growth rates were calculated. All compounds were run in duplicate at each concentration. Drug-free control cultures were run simultaneously. All data points represent means of at least two experimental tests. The 50% inhibitory concentration (EC50) was defined by comparison with drug-free controls incubated under the same conditions.29,30 Mammalian cells. A wild-type mouse FM3A cell line (subclone F-28-7) was supplied by the Health Sciences Research Resources Bank (Osaka, Japan). FM3A cells were maintained in suspension culture at 37°C in a 5% CO2 atmosphere in plastic bottles containing ES medium (Nissui Pharmaceutical Co., Ltd., Tokyo, Japan) supplemented with 2% heat-inactivated fetal bovine serum (Gibco, NY).31, 32 Toxicity to mammalian cells. The cell line grew with a doubling time of about 12 h. Before being exposed to drugs, cells were seeded at 990 µl of a density of 5 × 104 cells/ml, and various concentrations of compounds dispensed in 10 µl of distilled water were added to individual wells of a 24-well plate. The plates were incubated at 37 °C in a 5% CO2 atmosphere for 48 hrs. Cell numbers were measured using a blood cell counter CC-108 (Toa Medical Electric Co., Japan). All data points represent means of at least two experimental tests. The 50% inhibitory concentration (IC50) is defined by comparison with that of drug-free controls incubated under the same conditions. Cell growth inhibition is the index of cytotoxicity including cytostatic activity of the test compounds. Selective toxicity. Selective toxicity was estimated from the ratio (IC50/EC50) of the drug concentrations necessary to inhibit the growth rate of cells to 50% of the growth value between the malaria parasites and mouse mammary FM3A cells which served as a model host.33
Anti-HIV assay. The T cell line H9 was maintained in continuous culture with complete medium (RPMI 1640 with 10% fetal calf serum [FCS] supplemented with L-glutamine) at 5% CO2 and 37 °C. Aliquots of this cell line were used in experiments only when in the log-phase of growth. Test samples were first dissolved in DMSO. The following are the final drug concentrations routinely used for screening: 100, 20, 4, and 0.8 µg/mL. For active agents, additional dilutions are prepared for subsequent testing so that accurate EC50 values (see definition below) could be achieved. As the test samples were being prepared, an aliquot of H9 cells was infected with HIV-1 (IIIB isolate), while another aliquot was mock-infected with complete medium. The mock-infected sample was used for toxicity determinations (IC50, see definition below). The stock virus used for these studies typically had a TCID50 value of 104 Infectious Units (IU)/mL. The appropriate amount of virus for a multiplicity of infection between 0.1 and 0.01 IU/cell was added to the first aliquot of cells. The other aliquot of cells received only culture medium and was then incubated under identical conditions to the HIV-infected cells. After 4 h incubation at 37 °C and 5% CO2, both cell populations were washed three times with fresh medium and then added to the appropriate wells of a 24-well plate containing various concentrations of the test drug or culture medium (positive infected control/negative-control drug). In addition, AZT was also assayed during each experiment as a positive-control drug. The plates were incubated at 37 °C and 5% CO2 for 4 days. Cell-free supernatants were collected on day 4 and tested by an in-house p24 antigen ELISA assay; p24 antigen is a core protein of HIV and, therefore, is an indirect measure of virus present in the supernatants. Toxicity was determined by performing cell counts by a coulter counter on the mock-infected cells, which had received either culture medium (no toxicity), test sample or AZT. If a test sample had suppressive capability and was not toxic, its effects wre reported in the following terms: IC50, the concentration of test sample that was toxic to 50% of the mock-infected cells; EC50, the concentration of the test sample that was able to suppress HIV replication by 50%; and therapeutic index (TI), the ratio of IC50 to EC50.
ACKNOWLEDGMENT
This work was supported in part by a grant from the National Cancer Institute Grant CA-17625 awarded to K. H. Lee.
References
1. F. Pojer, E. Wemakor, B. Kammerer, H. Chen, C. T. Walsh, S.-M. Li, and L. Heide, Proc. Natl. Acad. Sci., 2003, USA 100, 2316.
2. D. J. Edwards and W. H. Gerwick, J. Am. Chem. Soc., 2004, 126, 11432. CrossRef
3. T. Kuzuyama, J. P. Noel, and S. B. Richard, Nature, 2005, 435, 983. CrossRef
4. M. Tello, T. Kuzuyama, L. Heide, J. P. Noel, S. B. Richard, Cell Mol. Life Sci., 2008, 65, 1459. CrossRef
5. S.-M. Li, Phytochemistry, 2009, 70, 1746. CrossRef
6. T. Usui, M. Kondoh, C. B. Cui, T. Mayumi, and H. Osada, Biochem. J., 1998, 333, 543.
7. B. Botta, A. Vitali, P. Menendez, D. Misiti, and M. G. Delle, Curr. Med. Chem., 2005, 12, 717.
8. K. Iwasa, M. Moriyasu, Y. Tachibana, H.-S Kim, Y. Wataya, W. Wiegrebe, K. F. Bastow, L. M. Cosentino, M. Kozuka, and K. H. Lee, Bioorg. Med. Chem., 2001, 9, 2871. CrossRef
9. W. H. Cui, K. Iwasa, H. Tokuda, A. Kashihara, Y. Mitani, T. Hasegawa, Y. Nishiyama, M. Moriyasu, H. Nishino, M. Hanaoka, C. Mukai, and K. Takeda, Phytochemistry, 2006, 67, 70. CrossRef
10. L. V. Rubinstein, R. H. Shoemaker, K. D. Paull, R. M. Simo, R. S. Tosini, P. Skehan, P. A.Scudiero, A. Monks, and M. R. Boyd, J. Natl. Cancer Inst., 1990, 82, 1113. CrossRef
11. Y. Ito, S. Yanase, J. Fujita, T. Harayama, T. Takashima, and H. Imanaka, Cancer Lett., 1981, 13, 29. CrossRef
12. Y. Kashiwada, F. Hashimoto, L. M. Cosentino, C. H. Chen, P. E. Garrett, and K. H. Lee, J. Med. Chem., 1996, 39, 1016. CrossRef
13. K. Iwasa, S. Okada, Y. Nishiyama, S. Takeuchi, M. Moriyasu, C. Tode, M. Sugiura, A. Takeuchi, H. Tokuda, K. Takeda, Y.-N. Liu, P-.C. Wu, K. F. Bastow, T. Akiyama, and K. H. Lee, Heterocycles, 2009, 77, 1355. CrossRef
14. B. Delley, J. Chem. Phys., 1990, 92, 508. CrossRef
15. B. Delley, J. Chem. Phys., 2000, 113, 7756. CrossRef
16. T. Mosmann, J. Immunol. Methods, 1983, 16, 55. CrossRef
17. M. Ishiyama, Y. Miyazono, K. Sasamoto, Y. Ohkura, and K. Ueno, Taranta, 1997, 44, 1299.
18. A. Murakami, H. Ohigashi, and K. Koshimizu, Biosci. Biotechnol. Biochem., 1996, 60, 1. CrossRef
19. K. Yasukawa, M. Takido, T. Matsumoto, M. Takeuchi, and S. Nakagawa, Oncology, 1991, 48, 72. CrossRef
20. T. Konoshima, M. Takasaki, M. Kozuka, T. Nagao, H. Okabe, N. Irino, T. Nakasumi, H. Tokuda, and H. Nishino, Biol. Pharm. Bull., 1995, 18, 284.
21. J. P. Perdew and Y. Wang, Phys. Rev. B, 1992, 45, 13244. CrossRef
22. A. D. Becke, J. Chem. Phys., 1988, 88, 2547. CrossRef
23. C. Lee, W. Yang, and R. G. Parr, Phys. Rev. B, 1988, 37, 786.
24. Y. Takemura, M. Ju-ichi, C. Ito, H. Furukawa, and H. Tokuda, Planta Med., 1995, 61, 366. CrossRef
25. M. S. Blois, Nature, 1958, 181, 1199. CrossRef
26. W. Trager and J. B. Jensen, Science, 1976, 193, 673. CrossRef
27. J. B. Jensen, W. Trager, and J. Doherty, Exp. Parasitol, 1979, 48, 36. CrossRef
28. R. W. Winter, K. A. Cornell, L. L. Johnson, M. Ignatushchenko, D. J. Hinrivhs, and M. K. Riscoe, Antimicrob Agents Chemother., 1996, 40, 1408.
29. A. V. O. Ofulla, V. C. N. Okoye, B. Khan, J. I. Githure, C. R. Roberts, A. J. Johnson, and S. K. Martin, Am. J. Trop. Med. Hyg., 1993, 49, 335.
30. H. Asahi and T. Kanazawa, Parasitology, 1994, 109, 397. CrossRef
31. A. Yoshioka, S. Tanaka, O. Hiraoka, Y. Koyama, Y. Hirota, D. Ayusawa, T. Seno, C. Garrett, and Y. Wataya, J. Biol. Chem., 1987, 262, 8235.
32. Y. Wataya and O. Hiraoka, Biochem. Biophys. Res. Commun, 1984, 123, 677. CrossRef
33. I. S. Shin, H. Tanifuji, Y. Arata, Y. Morizawa, T. Nakayama, and Y. Wataya, Parasitol. Res., 1995, 81, 622. CrossRef