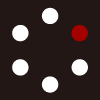
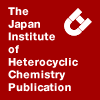
HETEROCYCLES
An International Journal for Reviews and Communications in Heterocyclic ChemistryWeb Edition ISSN: 1881-0942
Published online by The Japan Institute of Heterocyclic Chemistry
e-Journal
Full Text HTML
Received, 27th February, 2010, Accepted, 8th April, 2010, Published online, 9th April, 2010.
DOI: 10.3987/COM-10-11934
■ Rod-Like Conjugated Molecules with Ethynylene Linkage and Pyridazines Moieties: Synthesis and Light Emitting Properties
Caroline Hadad, Catherine Fiol-Petit, Anne-Sophie Cornec, Georges Dupas, Yvan Ramondenc, and Nelly Plé*
Department of Heterocyclic Chemistry (LCOFH), UMR 6014 CNRS, INSA and University of Rouen, 8 rue Tenière, 76131 Mont-Saint-Aignan Cedex, France
Abstract
In this paper, we describe the synthesis of various rod-like conjugated molecules with ethynyl and vinylpyridazines units. The key steps involve Sonogashira cross-coupling and condensation reactions. The light-emitting properties of some molecules are investigated in terms of absorption and emission spectra.INTRODUCTION
Linear arylethynyl molecules with extended π-conjugation have received a considerable research interest due to their potential applications as molecular wires,1 liquid crystals,2 components of organic light emitting devices (OLEDs) for displays and lighting,3 non-linear optical materials,4 and more particularly for two-photon absorption (TPA).5 Incorporation of one π-deficient heterocycle such as pyridine,6 1,3,5-triazine7 or diazine8-10 into the backbone of such molecules gives rise to new materials with interesting properties of electron conductivity and transport compared to their phenylene analogues. Ethynyl moieties added in the backbone lead to ethynyl aromatic/heteroaromatic systems which are versatile rigid-rod molecules. The main advantage of them compared to their arylenevinylene counterparts is the lack of possible Z/E photoisomerization which is important (because the TPA materials are photoexcited by the two-photon process) and their higher stability.11
With the aim to synthesize new conjugated structures containing ethynyl aromatic/heteroaromatic moieties, a highly electronegative pyridazine ring was utilized as the dipolar moiety for incorporation into the conjugated backbone. We report therein the synthesis of a new family of rod-like chromophores of the A-π-D, A-π-D-π-A and D-π-A-π-D types with donors (D) and pyridazines units (A) linked by a conjugated bridge (Scheme 1).
Such molecules could present several interesting applications; we report here the light emitting properties of some of them. This study is a part of our work dedicated to take advantages of diazines as building blocks for the synthesis of organic molecular materials.12
RESULTS AND DISCUSSION
The ethynylene moiety could be introduced using Sonogashira coupling reactions with chloro, triflate and iodo derivatives as starting materials.13 Among these methods, iodopyridazines were the most efficient and convenient starting materials chosen to react with trimethylsilylacetylene under modified Sonogashira conditions (Pd[PPh3]2Cl2, CuI, triethylamine in THF).14 Moreover, due to the π-deficient character of the pyridazine ring, the iodo derivatives can be easily obtained by nucleophilic substitution of a chlorine atom by an iodine one starting from commercially available chloro derivatives.15 This methodology was used to access to mono and bisalkynylpyridazines from the corresponding 3-iodo-6-methylpyridazine16 (1) (Scheme 2).
Starting from the 3,6-diiodopyridazine17 (2) and using double Sonogashira coupling reactions, centrosymmetric dialkynylpyridazines 7, 8 were obtained in moderate yields. A further coupling reaction of the julolidinylacetylene18 with the iodo compound 4 led to 9, which compared to the compound 5 presents a longer conjugated system (Scheme 3).
The synthetic ways used allowed us to access to chromophores of type A-π-D for 5 and 9 and chromophores of type A-π-D-π-A for 6 and D-π-A-π-D for 8. For this last compound, to appreciate the effect of the conjugation on the photophysical properties, it would be interesting to modify the nature of one of the conjugated linker by replacement of the ethynylene bridge by a vinylene one. In general, ethynylene-linked (-C≡C-) systems are less conjugated than the corresponding vinylene-linked (-C=C-) systems, because there are π- π and π*- π* energy mismatches at C(sp1)-C(sp2) connections.5
To incorporate a vinylene bridge in the backbone, a condensation reaction could be envisaged between the methyl group of the pyridazine ring and various commercially available aldehydes, providing an efficient synthetic way under environmentally friendly conditions.
The compound 5 substituted by a julolidinyl group, known to be an efficient electron-donating group D, has been chosen as a starting material. Condensation reaction was carried out in boiling aqueous 5M aqueous NaOH using Aliquat® 336 as a phase transfer agent, according to a previously reported procedure19a-d (Scheme 4).
The compound 12 possessing a terminal alkoxy group with a long chain reveals a liquid crystal behavior with a transition phase at 102 °C and a clearing temperature at 200 °C.
Geometrical, electronic and spectral properties
Geometry
We performed quantum mechanics calculations of the geometries and electronic structures using the ab-initio DFT method at the B3LYP level of theory20 with the 6-31G* basis set21 for compounds 5, 8, 9, 11-13. In all cases, the computed geometry reveals a null dihedral angle between the pyridazine ring and the vinylene or ethynylene chains; allowing a high charge transfer between the electron-withdrawing pyridazine ring and the alkoxy, dimethylamino or julolidinyl electron-donating groups (Table 1). Comparison of compounds 5 and 9 of type A-π-D highlights that incorporation in the chain of a phenyl group which induces a π-extended conjugation provides a lowering of the LUMO level. Comparison of data for compounds 5, 8, 11, 12, 13 clearly shows that incorporation of an electron donating group results in a decreasing of the HOMO-LUMO gap. The observed values for the alkyloxy (3.135), dimethylamino (3.124) and julolidinyl (3.057) groups are in good agreement with the generally admitted order of electron donating abilities and might correspond to a slight increasing of the absorption wavelengths (see further explanations related to Table 2).
UV/Vis and PL Spectroscopy
The optical properties of some of the synthesized compounds were investigated by UV/Vis and photoluminescence (PL) spectroscopy in CH2Cl2 at room temperature. The data obtained are summarized in Table 2 and their absorption and emission spectra in Figure 1.
Compounds 5 and 9 of type A-π-D have their absorption wavelengths (λabs) located in UV (374-393 nm) and their emission wavelengths (λem) in visible. Both compounds exhibit a high Stokes Shift (7492- 8064 cm-1) and for 9, possessing the most conjugated backbone, we observed a red shift in agreement with the lower calculated HOMO-LUMO gap (Table 1). The other compounds of type D-π-A-π-D with a larger number of π electrons (8, 11-13) have their absorption wavelengths (λabs) located in blue (401-439 nm) and their λem (528-562 nm) in visible. Comparison of compounds 11-13 allows us to appreciate the influence of the electron-donating groups on the phenyl ring. Replacement of an alkoxy group by an amino group (dimethylamino or julolidinyl) increases the absorption and emission wavelengths (λabs, λem), the highest bathochromic effect being observed for the julolidinyl group which is prone to have a stronger donating effect. Comparison of compounds 8 and 13 allows us to appreciate the relative influence of a vinylene or an ethynylene linker. No significant influence is observed on the absorption wavelengths, whereas the vinyl compound 13 has the highest λem and Stokes shifts but the centrosymmetric compound 8 exhibits the best quantum yield (φF).
CONCLUSION
The synthesis of various rod-like conjugated molecules with ethynyl and vinylpyridazines units has been performed using as key steps Sonogashira cross-coupling and aldol condensations reactions. These compounds have interesting light-emitting properties which are investigated in terms of absorption and emission spectra and high Stokes shifts. Presence of vinylene or ethynylenbridges allows an internal charge transfer along these molecules making them good candidates for two-photons dyes.
EXPERIMENTAL
Melting points were determined on a Kofler hot-stage with a 2 degrees accuracy (± 2 °C). The 1H and 13C NMR spectra were recorded on a Bruker AC 300 (300 MHz 1H, 75 MHz 13C) instrument. Microanalyses were performed on a Carlo Erba CHNOS 1160 apparatus, measurement accuracy is about ± 0.4% on carbon. The IR spectra were obtained as potassium bromide pellets with a Perkin-Elmer Paragon 500 spectrometer. Mass spectra were recorded on a GCMS-QP2010 Shimazu spectrometer. UV-Visible spectra were obtained on a Varian Can 50 scan spectrophotometer. Fluorescence spectroscopic studies were performed in a semi-micro fluorescence cell (Hellma®, 104F-QS, 10 x 4 mm, 1400 µL) with a Varian Cary Eclipse spectrophotometer. Tetrahydrofuran (THF) and diethyl ether (Et2O) were distilled from benzophenone sodium and used immediately. Water content of the solvent was estimated by the modified Karl Fisher method (less than 60 ppm of water). Toluene, dichloromethane (DCM), dimethylformamide (DMF) and triethylamine (NEt3) were distilled on CaH2. All reagents were purchased from Aldrich chemical Co., Acros or Alpha Aeser.
3-((4-Iodophenyl)ethynyl)-6-methylpyridazine (4)
To a solution of diiodobenzene (372 mg, 1.13 mmol), PdCl2(PPh3)2 (28 mg, 0.04 mmol) and CuI (14 mg, 0.07 mmol) in Et3N (7 mL) was added 3-ethynyl-6-methylpyridazine (3) (160 mg, 1.36 mmol). The reaction mixture was stirred under argon for 48 h at room temperature. The crude product was filtered over Celite and evaporated under reduced pressure. The residue was purified by silica gel column chromatography (eluent: petroleum ether/EtOAc 1/1) to give 188 mg (52%) of 4 as a dark orange solid. mp 179 °C. 1H NMR (CDCl3): δ 2.66 (s, 3H, CH3), 7.22-7.28 (m, 3H, 3 × CH), 6.44 (d, 1H, J = 8.5 Hz, CH), 7.64 (d, 2H, J = 8.5 Hz, 2 × CH). 13C (CDCl3): δ 22.5 (CH3), 87.2 (Cq), 92.1 (Cq), 95.9 (Cq), 121.2 (Cq), 126.3 (CH), 129.2 (CH), 133.5 (2 × CH), 137.7 (2 × CH), 145.9 (Cq), 158.6 (Cq). IR: 2221, 1576, 1538, 1482, 1412, 1388, 1087, 1055, 1006, 844, 812, 515 cm-1. Anal. Calcd for C13H9IN2 (319.89): C, 48.77; H, 2.83; N, 8.75. Found: C, 48.66; H, 2.90; N, 8.89. CI-MS (isobutane). Calcd for C13H9IN2: [M]+ = 320. Found: 321 [M+1].
3-(9-(Julolidinyl)ethynyl)-6-methylpyridazine (5)
To a solution of 3-iodo-6-methylpyridazine (1) (102 mg, 0.463 mmol), PdCl2(PPh3)2 (12 mg, 0.016 mmol) and CuI (6 mg, 0.030 mmol) in Et3N (3 mL) was added ethynyljulolidine18 (120 mg, 0.603 mmol). The reaction mixture was stirred under argon for 8 h at 95 °C. After cooling, the crude product was filtered over Celite and evaporated under reduced pressure. The residue was purified by silica gel column chromatography (eluent: DCM/EtOAc 1/1) to give 80 mg (59%) of 5 as a brown solid. mp 124 °C. 1H NMR (CDCl3): δ 1.93 (m, 4H, 2 × CH2), 2.63-2.78 (m, 7H, CH3 and 2 × CH2), 3.19 (t, 4H, J = 5.7 Hz, 2 × CH2), 7.04 (s, 2H, 2 × CH), 7.23 (d, 1H, J = 8.7 Hz, CH), 7.42 (d, 1H, J = 8.7 Hz, CH). 13C (CDCl3): δ 21.6 (2 × CH2), 22.4 (CH3), 27.6 (2 × CH2), 49.9 (2 × CH2), 84.2 (Cq), 96.2 (Cq), 106.7 (Cq), 121.0 (2 × Cq), 126.1 (CH), 129.2 (CH), 131.0 (2 × CH), 141.9 (Cq), 146.9 (Cq), 157.3 (Cq). IR: 2932, 2850, 2185, 1600, 1577, 1519, 1458, 1401, 1341, 1312, 1239, 1203, 1138, 1088, 1047, 885, 834 cm-1. CI-MS (isobutane). Calcd for C19H19N3: [M]+ = 289. Found: 290 [M+1].
1,4-Bis((6-methylpyridazin-3-yl)ethynyl)-2,5-dioctyloxybenzene (6)
To a solution of 3-iodo-6-methylpyridazine (1) (63 mg, 0.287 mmol) and 1,4-diethynyl-2,5-dioctyloxybenzene (50 mg, 0.130 mmol) in Et3N (3 mL) were added PdCl2(PPh3)2 (6 mg, 0.009 mmol) and CuI (3 mg, 0.013 mmol). The reaction mixture was stirred under argon for 3 days at room temperature. Water (20 mL) was added and the aqueous phase was extracted with DCM (3 × 20 mL). The combined organic extracts were dried over anhydrous MgSO4, filtered and evaporated under reduced pressure. The residue was purified by silica gel column chromatography (eluent: petroleum ether/EtOAc 1/1) to give 23 mg (36%) of 6 as a yellow solid. mp 150-151 °C. 1H NMR (CDCl3): δ 0.72-0.86 (m, 6H, 2 × CH3), 1.16-1.52 (m, 20H, 10 × CH2, 5 × CH), 1.85 (m, 4H, 2 × CH2), 2.70 (s, 6H, 2 × CH3), 3.96 (t, 4H, J = 6.6 Hz, 2 × CH2), 7.08 (s, 2H, 2 × CH), 7.22 (d, 2H, J = 8.7 Hz, 2 × CH), 7.44 (d, 2H, J = 8.7 Hz, 2 × CH). 13C (CDCl3): δ 14.3 (2 × CH3), 22.4 (2 × CH3), 22.8 (2 × CH2), 26.0 (2 × CH2), 29.3 (2 × CH2), 29.4 (2 × CH2), 29.5 (2 × CH2), 31.9 (2 × CH2), 69.7 (2 × CH2), 89.8 (2 × Cq), 91.5 (2 × Cq), 113.6 (2 × Cq), 117.3 (2 × CH), 126.2 (2 × CH), 129.7 (2 × CH), 146.2 (2 × Cq), 154.1 (2 × Cq), 158.4 (2 × Cq). IR: 2947, 2922, 2217, 1505, 1471, 1401, 1389, 1282, 1219, 864, 724 cm-1. CI-MS (isobutane). Calcd for C36H46N4O2: [M]+ = 566. Found : 567 [M+1].
3,6-Bis((4,6-dimethylpyrimidin-2-yl)ethynyl)pyridazine (7)
To a solution of 3,6-diiodopyridazine (2) (118 mg, 0.358 mmol) and 2-ethynyl-4,6-dimethylpyrimidine (102 mg, 0.770 mmol) in a mixture of Et3N and DMSO (2.5/1 mL) were added PdCl2(PPh3)2 (9 mg, 0.012 mmol) and CuI (3 mg, 0.012 mmol). The reaction mixture was stirred under argon for 48 h at room temperature. Solvents were evaporated under reduced pressure and DCM (20 mL) was added. The organic phase was extracted with water (2 × 20 mL), dried over anhydrous MgSO4, filtered and evaporated under reduced pressure. The residue was purified by silica gel column chromatography (eluent: DCM/MeOH 9/1) to give 65 mg (53%) of 7 as a brown solid. mp 224-226 °C. 1H NMR (CDCl3): δ 2.51 (s, 12H, 4 × CH3), 7.05 (s, 2H, 2 × CH), 7.71 (s, 2H, 2 × CH). 13C (CDCl3): δ 24.0 (4 × CH3), 81.6 (2 × Cq), 93.0 (2 × Cq), 120.2 (2 × CH), 129.4 (2 × CH), 145.4 (2 × Cq), 151.5 (2 × Cq), 167.5 (4 × Cq). IR: 2226, 1587, 1530, 1437, 1408, 1385, 1369, 1352 cm-1. CI-MS (isobutane). Calcd for C20H16N6: [M]+ = 340. Found: 341 [M+1].
3,6-Bis(9-julolidinylethynyl)pyridazine (8)
To a solution of 3,6-diiodopyridazine (2) (108 mg, 0.325 mmol) and ethynyljulolidine18 (141 mg, 0.715 mmol) in a mixture of Et3N (2.5 mL) and DMSO (1 mL) were added PdCl2(PPh3)2 (8 mg, 0.011 mmol) and CuI (3 mg, 0.011 mmol). The reaction mixture was stirred under argon for 48 h at room temperature. Solvents were evaporated under reduced pressure and DCM (20 mL) was added. The organic phase was extracted with water (2 × 20 mL), dried over anhydrous MgSO4, filtered and evaporated under reduced pressure. The residue was purified by silica gel column chromatography (eluent: petroleum ether/EOAct 1/1) to give 102 mg (67%) of 8 as a dark orange solid. mp 200-202 °C. 1H NMR (CDCl3): δ 1.95 (m, 8H, 4 × CH2), 2.76 (t, 8H, J = 6.4 Hz, 4 × CH2), 3.22 (t, 8H, J = 5.7 Hz, 4 × CH2), 7.07 (s, 4H, 4 × CH), 7.42 (s, 2H, 2 × CH). 13C (CDCl3): δ 21.7 (4 × CH2), 27.7 (4 × CH2), 50.0 (4 × CH2), 84.9 (2 × Cq), 98.3 (2 × Cq), 106.6 (2 × Cq), 121.0 (4 × Cq), 128.2 (2 × CH), 132.1 (4 × CH), 144.1 (2 × Cq), 145.8 (2 × Cq). IR: 2936, 2190, 1651, 1601, 1527, 1481, 1435, 1376, 1319, 1239, 1142, 888, 839, 768 cm-1. Anal. Calcd for C32H30N4 (470.25): C, 81.67; H, 6.43; N, 11.91. Found: C, 81.56; H, 6.27; N, 11.75.
[4-(6-Methylpyrazin-3-yl)phenyl]julolidinylacetylene (9)
To a solution of 3-((4-iodophenyl)ethynyl)-6-methylpyridazine (4) (166 mg, 0.519 mmol), PdCl2(PPh3)2 (13 mg, 0.018 mmol) and CuI (6 mg, 0.034 mmol) in Et3N (3 mL) was added ethynyljulolidine (153 mg, 0.777 mmol). The reaction mixture was stirred under argon at 95 °C for 48 h. The crude product was filtered over Celite and evaporated under reduced pressure. The residue was purified by silica gel column chromatography (eluent: petroleum ether/EtOAc 1/1) to give 174 mg (86%) of 9 as an orange-brown solid. mp 207 °C. 1H NMR (CDCl3): δ 1.96 (q, 4H, J = 5.4 Hz, 2 × CH2), 2.64-2.80 (m, 7H, 2 × CH2 and CH3), 3.19 (t, 4H, J = 5.4 Hz, 2 × CH2), 6.98 (s, 2H, 2 × CH), 7.31 (d, 1H, J = 8.7 Hz, CH), 7.40-7.58 (m, 5H, 5 × CH). 13C (CDCl3): δ 21.8 (2 × CH2), 22.6 (CH3), 27.7 (2 × CH2), 50.0 (2 × CH2), 86.8 (Cq), 87.2 (Cq), 93.3 (Cq), 94.4 (Cq), 108.2 (Cq), 120.2 (Cq), 121.1 (2 × Cq), 125.9 (Cq), 126.3 (CH), 129.6 (CH), 130.5 (2 × CH), 131.2 (2 × CH), 132.1 (2 × CH), 143.4 (Cq), 146.2 (Cq), 158.4 (Cq). CI-MS (isobutane). Calcd for C27H23N3: [M]+ = 389. Found: 390 [M+1].
3-[4-(9-Julolidinylethynyl)phenyl]-6-(4-bromostyryl)pyrazine (10)
In a 25 mL round-bottomed flask were added Aliquat 336 (7 mg, 0.016 mmol)4-bromobenzaldehyde (34 mg, 0.181 mmol), [4-(6-methylpyrazin-3-yl)phenyl]julolidinylacetylene (9) (41 mg, 0.140 mmol) and sodium hydroxide aqueous solution (5 M, 5 mL). The reaction vessel was heated to reflux under stirring for 24 h. After cooling, water (30 mL) was added and the aqueous phase was extracted with DCM (3 × 20 mL). The combined organic extracts were dried over anhydrous MgSO4, filtered and evaporated under reduced pressure. The residue was purified by silica gel column chromatography (eluent: petroleum ether/EtOAc 6/4) to give 24 mg (38%) of 10 as a yellow solid. mp 215-217 °C. 1H NMR (CDCl3): δ 1.95 (m, 4H, 2 × CH2), 2.72 (t, 4H, J = 6.4 Hz, 2 × CH2), 3.22 (t, 4H, J = 5.7 Hz, 2 × CH2), 7.08 (s, 2H, 2 × CH), 7.36 (d, 1H, J = 16.4 Hz, CH), 7.42-7.59 (m, 7H, 7 × CH). Anal. Calcd for C26H22BrN3 (455.10): C, 68.43; H, 4.86; N, 9.21. Found C, 68.29; H, 4.56; N, 8.95.
3-[4-(9-Julolidinylethynyl)phenyl]-6-(4-dimethylaminostyryl)pyrazine (11)
In a 25 mL round-bottomed flask were added Aliquat 336 (5 mg, 0.013 mmol 4-N,N-(dimethylamino)benzaldehyde (26 mg, 0.174 mmol), [4-(6-methylpyrazin-3-yl)phenyl]julolidiny lacetylene (9) (39 mg, 0.135 mmol) and sodium hydroxide solution (5 M, 6 mL). The reaction vessel was heated to reflux under stirring for 24 h. After cooling, water (30 mL) was added and the aqueous phase was extracted with DCM (3 x 20 mL). The combined organic extracts were dried over anhydrous MgSO4, filtered and evaporated under reduced pressure. The residue was purified by silica gel column chromatography (eluent: petroleum ether/EtOAc 1/1) to give 39 mg (70%) of 11 as a dark red solid. mp> 260 °C. 1H NMR (CDCl3): δ 1.95 (m, 4H, 2 × CH2), 2.75 (t, 4H, J = 6.2 Hz, 2 × CH), 3.01 (s, 6H, 2 × CH3), 3.21 (t, 4H, J = 5.7 Hz, 2 × CH2), 6.0 (d, 2H, J = 8.4 Hz, 2 × CH), 7.07 (s, 2H, 2 × CH), 7.20 (d, 1H, J = 16.4 Hz), 7.41-7.55 (5H, 5 × CH). 13C (CDCl3): δ 21.7 (2 × CH2), 27.7 (2 × CH2), 40.4 (2 × CH3), 50.0 (2 × CH2), 85.0 (Cq), 98.8 (Cq), 107.0 (Cq), 112.2 (2 × CH), 120.6 (CH), 121.0 (2 × Cq), 122.2 (CH), 124.3 (Cq), 128.8 (2 × CH), 129.0 (CH), 131.1 (2 × CH), 135.2 (CH), 143.8 (Cq), 146.2 (Cq), 151.0 (Cq), 156.3 (Cq). IR: 2927, 2835, 2181, 1599, 1518, 1459, 1430, 1349, 1307, 1241, 1187, 1161, 1143, 970, 887, 839, 811 cm-1. CI-MS (isobutane). Calcd for C28H28N4: [M]+ = 420. Found: 421 [M+1].
3-[4-(9-Julolidinylethynyl)phenyl]-6-(4-octyloxystyryl)pyrazine (12)
In a 25 mL round-bottomed flask were added Aliquat 336 (6.5 mg, 0.016 mmol), 4-(octyloxy)benzaldehyde (50 mg, 0.211 mmol), [4-(6-methylpyrazin-3-yl)phenyl]julolidinylacetylene (9) (47 mg, 0.163 mmol) and aqueous sodium hydroxide solution (5 M, 6 mL). The reaction vessel was heated to reflux under stirring for 24 h. After cooling, water (30 mL) was added and the aqueous phase was extracted with DCM (3 × 20 mL). The combined organic extracts were dried over anhydrous MgSO4, filtered and evaporated under reduced pressure. The residue was purified by silica gel column chromatography (eluent: petroleum ether/EtOAc 6/4) to give 50 mg (61%) of 12 as an orange solid mp 200-202 °C. 1H NMR (CDCl3): δ 0.82-0.96 (m, 3H, CH3), 1.20-1.51 (m, 10H, 5 × CH2), 1.79 (q, 2H, J = 6.6 Hz, CH2), 1.95 (q, 4H, J = 6.4 Hz, 2 × CH2), 2.73 (t, 4H, J = 6.4 Hz, 2 × CH2), 3.21 (t, 4H, J = 5.8 Hz, 2 × CH2), 3.99 (t, 2H, J = 6.6 Hz, CH2), 6.91 (d, 2H, J = 8.7 Hz, 2 × CH), 7.08 (s, 2H, 2 × CH), 7.26 (d, 1H, J = 16.4 Hz, CH), 7.40-7.57 (5H, 5 × CH). 13C (CDCl3): δ 14.2 (CH3), 21.7 (CH2), 22.8 (2 × CH2), 26.1 (CH2), 27.7 (2 × CH2), 29.3-29.4 (4 × CH2), 31.9 (CH2), 50.0 (2 × CH2), 68.3 (CH2), 84.9 (Cq,), 97.3 (Cq,), 106.8 (Cq), 114.9 (2 × CH), 121.0 (2 × Cq), 122.6 (CH), 122.9 (CH), 128.7 (Cq), 129.0 (2 × CH), 129.1 (CH), 131.1 (2 × CH), 134.6 (CH), 144.0 (Cq), 146.7 (Cq), 155.7 (Cq), 160.1 (Cq). IR: 2926, 2853, 2198, 1667, 1631, 1603, 1573, 1510, 1466, 1425, 1344, 1309, 1252, 1174, 1104, 1031, 976, 839 cm-1. Anal. Calcd for C35H39N3O (505.31): C, 80.75; H, 7.77; N, 8.31. Found C, 80.47; H, 7.56; N, 8.18.
3-[4-(9-Julolidinylethynyl)phenyl]-6-julolidinyl)pyrazine (13)
In a 25 mL round-bottomed flask were added Aliquat 336 (5 mg, 0.012 mmol),julolidinylaldehyde (37 mg, 0.185 mmol), [4-(6-methylpyrazin-3-yl)phenyl]julolidinylacetylene (9) (36 mg, 0.124 mmol) and aqueous sodium hydroxide solution (5 M, 6 ml). The reaction vessel was heated to reflux under stirring for 24 h. After cooling, water (30 mL) was added and the aqueous phase was extracted with DCM(3 × 20 mL). The combined organic extracts were dried over anhydrous MgSO4, filtered and evaporated under reduced pressure. The residue was purified by silica gel column chromatography (eluent: petroleum ether/EtOAc 6/4) to give 10 mg (17%) of 13 as an orange solid. mp > 260 °C decomposition. 1H NMR (CDCl3): δ 1.88-2.02 (m, 8H, 4 × CH2), 2.27-2.81 (m, 8H, 4 × CH2), 3.21 (t, 8H, J = 5.7 Hz, 4 × CH2), 7.02-7.08 (m, 4H, 4 × CH), 7.12 (d, 1H, J = 16.4 Hz), 7.36 (d, 1H, J = 7.0 Hz, CH), 7.39-7.51 (m, 2H, 2 × CH). IR: 2920, 2842, 2206, 1651, 1479, 1435, 1374, 1263, 1199, 969, 768 cm-1. HRMS (ESI) calcd for [C32H32N4+H]: 473.2705. Found: 473.2722.
References
1. a) J. M. Tour, Acc. Chem. Res., 2000, 33, 791; CrossRef b) J. M. Tour, Chem. Rev., 1996, 96, 537; CrossRef c) J. M. Lehn, ’Supramolecular Chemistry: Concepts and Perspectives’ VCH: Weinheim 1995; d) C. Wang, A. S. Batsanov, and M. R. Bryce, J. Org. Chem., 2006, 71, 108. CrossRef
2. a) G. W. Gray, M. Hird, and K. J. Toyne, Mol. Cryst. Liq. Cryst., 1991, 195, 221; CrossRef b) L. K. M. Chan, G. W. Gray, D. Lacey, T. Shrithanratana, and K. J. Toyne, Mol. Cryst. Liq.Cryst., 1987, 150B, 335; CrossRef c) L. K. M. Chan, G. W. Gray, D. Lacey, and K. J. Toyne, Mol. Cryst. Liq. Cryst., 1988, 158B, 209; CrossRef d) G. W. Gray, M. Hird, D. Lacey, and K. J. Toyne, J. Chem. Soc., Perkin Trans. 2, 1989, 2041; CrossRef e) M. Hird, K. J. Toyne, G. W. Gray, D. G. McDonnel, and I. C. Sage, Liq. Cryst., 1995, 18, 1. CrossRef
3. a) A. Kraft, A. C. Grimsdale, and A. B. Holmes, Angew. Chem. Int. Ed., 1998, 37, 402; CrossRef b) U. Mitschke and P. Bäuerle, J. Mater. Chem., 2000, 10, 1471. CrossRef
4. a) M. Albota, D. Beljonne, J.-L. Brédas, J. E. Erhlich, J.-Y. Fu, A. A. Heikel, S. E. Hess, T. Kogej, M. D. Levin, S. R. Marder, D. McCord-Maughon, J. W. Perry, H. Röckel, M. Rumi, G. Subramaniam, W. W. Webb, X.-L. Wu, and C. Xu, Science, 1998, 281, 1653; CrossRef b) A. Hayek, F. Bolze, J.-F. Nicoud, P. L. Baldeck, and Y. Mély, Photochem. Photobiol. Sci., 2000, 5, 102; CrossRef c) F. Cherioux, A.-J. Attias, and H. Maillotte, Adv. Funct. Mater., 2002, 12, 20; CrossRef d) A.-L. Roy, M. Chavarot, E. Rose, F. Rose-Munch, A.-J. Attias, D. Kréher, J.-L Fave, and C. Kamierszky, C. R. Chimie, 2005, 8, 1256. CrossRef
5. M. Pawlicki, H. A. Collins, R. G. Denning, and H. L. Anderson, Angew. Chem. Int. Ed., 2009, 48, 3244. CrossRef
6. a) J. K. Kim, J. W. Yu, J. M. Hong, H. N. Cho, D. Y. Kim, and C. Y. Kim, J. Mater. Chem., 1999, 9, 2171; CrossRef b) J. A. Irvin, C. J. Dubois, and J. R. Reynolds, Chem. Commun., 1999, 2121; CrossRef c) A. P. Monkman, L.-O. Pålsson, R. W. T. Higgins, C. Wang, M. R. Bryce, and J. A. K. Howard, J. Am. Chem. Soc., 2002, 124, 6049. CrossRef
7. a) J. M. Lupton, L. R. Hemingway, I. D. W. Samuel, and P. L. Burn, J. Mater. Chem., 2000, 10, 867; CrossRef b) J. Pang, Y. Tao, S. Freiberg, X.-P. Yang, M. D’Iorio, and S. Wang, J. Mater. Chem., 2002, 12, 206. CrossRef
8. a) G. Hugues, C. Wang, A. S. Batsanov, M. Fern, S. Frank, M. R. Bryce, I. F. Perepichka, A. P. Monkman, and P. Lyons, Org. Biomol. Chem., 2003, 1, 3069; CrossRef b) K. T. Wong, T. S. Hung, Y. Lin, C.-C. Wu, G.-H. Lee, S.-M. Peng, C.H. Chou, and Y. O. Su, Org. Lett., 2002, 4, 513; CrossRef c) C. C. Wu, Y. T. Lin, H. H. Chiang, T. Y. Cho, C. W. Chen, K. T. Wong, Y. L. Liao, G. H. Lee, and S. M. Peng, Appl. Phys. Lett., 2002, 81, 577. CrossRef
9. L. Zhao, I. F. Perepichka, F. Türsksoy, A. S. Batsanov, A. Beeby, K. S. Findlay, and M. R. Bryce, New J. Chem., 2004, 28, 912. CrossRef
10. S. Achelle, N. Plé, D. Kreher, F. Mathevet, A. Turck, and A.-J. Attias, Heterocycles, 2008, 75, 357. CrossRef
11. W. J. Yang, C. H. Kim, M. Y. Jeong, S. K. Lee, M. J. Piao, S. J. Jeon, and B. R. Cho, Chem. Mater., 2004, 16, 2783. CrossRef
12. a) V. Gautheron Chapoulaud, N. Plé, A. Turck, and G. Quéguiner, Tetrahedron, 2000, 30, 5499; CrossRef b) F. Toudic, A. Heynderickx, N. Plé, A. Turck, and G. Quéguiner, Tetrahedron, 2003, 59, 6375; CrossRef c) A. Busch, V. Gautheron Chapoulaud, J. Audoux, N. Plé, and A. Turck, Tetrahedron, 2004, 60, 5373; CrossRef d) S. Achelle, N. Plé, A. Turck, J.-P. Bouillon, and C. Portella, J. Heterocycl. Chem., 2006, 43, 1243; CrossRef e) A. Busch, A. Turck, K. Nowicka, A. Barcella, C. Andraud, and N. Plé, Heterocycles, 2007, 71, 1723; CrossRef f) N. Hebbar, Y. Ramondenc, G. Plé, G. Dupas, and N. Plé, Tetrahedron, 2009, 65, 4190. CrossRef
13. a) Y. Abe, A. Ohsawa, H. Arai, and H. Igeta, Heterocycles, 1978, 9, 1397; CrossRef b) A. Ohsawa, Y. Abe, and H. Igeta, Chem. Lett., 1979, 241; CrossRef c) G. Heinisch and W. Holzer, Can. J. Chem., 1991, 69, 972; CrossRef d) D. Tousaint, J. Suffert, and C. G. Wermuth, Heterocycles, 1994, 38, 1273; CrossRef e) D. S. Chekmarev, A. E. Stepanov, and A. N. Kasatkin, Tetrahedron Lett., 2005, 46, 1303; CrossRef f) B. U. W. Maes, P. Tapolcsányi, C. Meyers, and P. Mátyus, Curr. Org. Chem., 2006, 10, 377. CrossRef
14. T. L. Draper and T. R. Bailey, J. Org. Chem., 1995, 60, 748. CrossRef
15. a) P. Coad, R. A. Coad, S. Clough, J. Hyepock, R. Salisbury, and C. Wilkins, J. Org. Chem., 1963, 28, 218; CrossRef b) M. S. Shin, Y. J. Kang, H. A. Chung, J. W. Park, D. H. Kweon, W. S. Lee, Y. J. Yoon, and S. K. Kim, J. Heterocycl. Chem., 1999, 36, 1135. CrossRef
16. a) N. K. Basu and F. L. Rose, J. Chem. Soc., 1963, 5660; CrossRef b) M. Ogata, Chem. Pharm. Bull., 1963, 11, 1522.
17. G. Heinisch and W. Holzer, Can. J. Chem., 1991, 69, 972. CrossRef
18. N. Hebbar, Ph.D. University of Rouen 2008.
19. a) J. J. Vanden Eynde, L. Pascal, Y. Van Haverbeke, and P. Dubois, Synth. Commun., 2001, 31, 3167; CrossRef b) L. Pascal, J. J. Vanden Eynde, Y. Van Haverbeke, and P. Dubois, Lett. Org. Chem., 2004, 1, 112; CrossRef c) M. Mainil, L. Pascal, Vanden Eynde, Y. Van Haverbeke, and P. Dubois, e-Polymers, 2003, 056; d) D. M. Brown and A. A. R. Kon, J. Chem. Soc., 1948, 2147. CrossRef
20. a) A. D. Becke, J. Chem. Phys., 1993, 98, 5648; CrossRef b) C. T. Lee, W. T. Yang, and R. G. Parr, Phys. Rev. B, 1988, 97, 785. CrossRef
21. Gaussian 03, Revision C.02, M. J. Frisch, G. W. Trucks, H. B. Schlegel, G. E. Scuseria, M. A. Robb, J. R. Cheeseman, J. A. Montgomery, Jr., T. Vreven, K. N. Kudin, J. C. Burant, J. M. Millam, S. S. Iyengar, J. Tomasi, V. Barone, B. Mennucci, M. Cossi, G. Scalmani, N. Rega, G. A. Petersson, H. Nakatsuji, M. Hada, M. Ehara, K. Toyota, R. Fukuda, J. Hasegawa, M. Ishida, T. Nakajima, Y. Honda, O. Kitao, H. Nakai, M. Klene, X. Li, J. E. Knox, H. P. Hratchian, J. B. Cross, C. Adamo, J. Jaramillo, R. Gomperts, R. E. Stratmann, O. Yazyev, A. J. Austin, R. Cammi, C. Pomelli, J. W. Ochterski, P. Y. Ayala, K. Morokuma, G. A. Voth, P. Salvador, J. J. Dannenberg, V. G. Zakrzewski, S. Dapprich, A. D. Daniels, M. C. Strain, O. Farkas, D. K. Malick, A. D. Rabuck, K. Raghavachari, J. B. Foresman, J. V. Ortiz, Q. Cui, A. G. Baboul, S. Clifford, J. Cioslowski, B. B. Stefanov, G. Liu, A. Liashenko, P. Piskorz, I. Komaromi, R. L. Martin, D. J. Fox, T. Keith, M. A. Al-Laham, C. Y. Peng, A. Nanayakkara, M. Challacombe, P. M. W. Gill, B. Johnson, W. Chen, M. W. Wong, C. Gonzalez, and J. A. Pople, Gaussian, Inc., Wallingford CT, 2004.
22. a) S. Fery-Forgues and D. Lavabre, J. Chem. Educ., 1999, 76, 1260; CrossRef b) R. Rusakowicz and A. C. Testa, J. Phys. Chem., 1968, 72, 2680; CrossRef c) A. Pardo, D. Reyman, J. M. L. Poyato, and F. Medina, J. Lumin., 1992, 51, 269; CrossRef d) Q. Umberger and V. K. LaMer, J. Am. Chem. Soc., 1945, 67, 1099. CrossRef