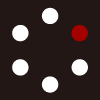
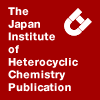
HETEROCYCLES
An International Journal for Reviews and Communications in Heterocyclic ChemistryWeb Edition ISSN: 1881-0942
Published online by The Japan Institute of Heterocyclic Chemistry
e-Journal
Full Text HTML
Received, 31st May, 2010, Accepted, 5th July, 2010, Published online, 6th July, 2010.
DOI: 10.3987/REV-10-SR(E)1
■ The Chemistry of Azaazulenes
Noritaka Abe* and Takahiro Gunji
Department of Industrial Chemistry, Faculty of Science and Technology, Tokyo University of Science, 2641 Yamazaki, Noda, Chiba 278-8510, Japan
Abstract
This review describes the synthetic methods and reactions of azaazulenes including some of their dihydro-, oxo-derivatives and hetero-fused derivatives published during 2000 to early in 2010. The biological and physical properties of azaazulenes are also described.INTRODUCTION
Azaazulenes are a class of the compounds that have been receiving continual interest of chemists for their relationship with the chemistry of azulenes and for remarkable characters about physical and chemical properties as well as biological activities.1 After being previously reviewed a decade ago,1a,b many significant researches were developed in this field. This review covers the recent progress in the chemistry of fully-conjugated azaazulenes including some of their dihydro-, oxo-derivatives and hetero-fused derivatives published during 2000 to early in 2010.
I. SYNTHESES OF AZAAZULENES
Syntheses of 1-azazulenes from reactive troponoids were fundamental methods.1 Recently, some novel synthetic methods of 1-azaazulenes being based on seven-menvered ring nuclei were developed. Konakahara reported the new approach to the synthesis of functionalized 1-azaazulenes by the reaction of the N-silylenamine or the enamine with 2-substituted tropones. (Scheme 1).2 The reaction of N-silylenamine (1a) with 2-chlorotropone (2a) in the presence of KF gave 2-substituted-azaazulene (3a) in 24% yield. The reaction of the enamines (1b-e) with 2-chlorotropone or tropolone tosylate (2b) in the presence of Et3N gave corresponding azaazulenes (3b-e) (Scheme 1). It is indicated that an aprotic polar solvent was effective for the reaction. Interestingly, the reaction of the enamines (1c-f) with 4-isopropyltropolone tosylate (4) gave 6-isopropylidene-8a-hydroxy-6,8a-dihydroazaazulene derivatives(5c-f), respectively (Scheme 2).
As related reaction, it is reported that the reaction of chlorotropones (2) with the anion, prepared from active methyl compounds, with arylcarbonitriles in the presence of base gave 1-azaazulene derivatives (5) (Scheme 3).3
Narasaka reported elegant synthetic methods which are adopted of amino-Heck reaction to cycloheptatrienylmethyl ketone oximes.4a-c Thus, the treatment of cycloheptatrienylmethyl ketone O-pentafluorobenzoyloximes (6) with a catalytic amount of Pd(dba)2-t-Bu3P and triethylamine in the presence of MS 4A followed by the oxidation with MnO2 gave various 1-azaazulenes (7) in moderate to good yields (Scheme 4).
This method was applied to the synthesis of fused azaazulene derivatives, and 6-azanaphth[1,2-b]azulene (8) and 11,12-dihydro-6-azanaphth[1,2-b]azulene (9) were obtained (Scheme 5).
Convenient preparation of heteroazulenes by the reaction of 2-aminotropone (10) with heterocumulenes was reported by Nitta.5 The reaction of 10 with N,N’-diphenylcarbodiimide (11a) in the presence of t-BuOK gave 1,3-diazaaulene derivative (12a). Similarly, the reaction of 10 with heterocumulenes, such as phenyl isothiocyanate (11b), phenyl isocyanate (11c), and carbon disulfide (11d), in the presence of t-BuOK gave heteroazulenes (12b-f) (Scheme 6).
Nitta early reviewed the utility of (vinylimino)phosphoranes as useful building blocks for the synthesis of azaheterocycles including azaazulenes.6 In the extension of the researches, Nitta reported the reactions of (tropon-2-ylimino)pnictoranes (13a-d) with heterocumulenes (11a-d), which are good methods for construction of azaazulene skeletons (14a-d) (Scheme 7).7a-c
They also reported the synthesis of 2-(1-triphenylphosphoranylideneethyl)tropones (15a) and 2-(triphenylarsoranylideneethyl)tropones (15b). Reaction of 15a with heterocumulenes (11a-c) gave 1-azaazulene derivatives (16), 1-oxaazulene derivatives (17), and 1-thiaazulene derivatives (18) (Scheme 8).8a,b
The reaction of 15b with heterocumulenes (11a-c) of dimethyl acetylenedicarboxylate (DMAD) resulted in the formation of heteroazulenes (18-20) and azulene derivatives (21) (Scheme 9).8c
The [8 + 2]-cycloaddition of 8-azaheptafulvenes (cycloheptatrienylideneamines; troponeimines) is a good method for construction of the 1-azaazulene skeleton.9 Reaction of 8-aryl-8-azaheptafulvenes (22) with trimethylsilylketene followed by the desilylation with TBAF and successive oxidation with MnO2 gave 1-aryl-1-azaazulen-2(1H)-ones (23) (Scheme10).10
The reaction of 8-aryl-8-azaheptafulvenes (22) with activated stylenes underwent an efficient [8 + 2]-cycloaddition to give 2-aryl-1,2,3,3a-tetrahydro-1-azaazulene derivatives (24) (Scheme 11).11
A facile and practical method for the synthesis of fully-conjugated substituted-1-azaazulenes from 8-azaheptafulvene N-sulfonates was reported. The treatment of 8-(substituted sulfonyloxy)-8-azaheptafulvenes (25) and the ketonic methylene compounds (26) with bases such as amines in the presence of MS 3A or MS 4A gave 1-azaazulene derivatives (27) (Scheme 12).3
For the preparation of heteroaromatics being fused to 1,3-diazaazulene, the reaction of 5-nitrosotropolone (28) with pyridines was carried out, and pyrido[1’,2’:1,2]imidazo[4,5-e]tropolone (29) and its acetate (30) were obtained via tropoquinone derivative (31). (Scheme 13) 12 The treatment of 31 with pyridine afforded 29 and this result exemplified that 31 is the precursor of 29. The reaction was applicable to azines, such as pyridazine, isoquinoline, phthalazine, or thiazole, and corresponding tricyclic heterocycles were obtained.12
Nitta synthesized the uracil-annulated azaazulenes, which showed an oxidizing ability.13a-f The treatment of 2-chlorotropone (2a) with 32 in the presence of Et3N and K2CO3 in refluxing 1,4-dioxane gave 33. The reaction was improved;13a the treatment of 2a with 32 in the presence of t-BuNH2 in ethanol at rt gave 33 in good yields (Scheme 14).13b Compounds (33) were also synthesized via 35 by the ring transformation of 34 in good yields by the treatment with amines followed by heating at 90 ºC in 1,4-dioxane.13b
By using similar ring transformation, Nitta synthesized 36 from 37 with the amines via 38 (Scheme 15).13c The treatment of 33 with MeI followed by HBF4 also gave 6-aminouracil fused 1-azaazulenium ions.13d They extended the procedure to synthesize areno-annulated 6-aminouracil-fused 1-azaazulenium ions (39-41)13e and the ions 42 and 43 (Figure 1).13f
Ring expansion of benzene ring to seven-membered ring being attended with pyrrole ring formation is a fascinating synthetic method for the construction of the 7-5 ring system at a stroke.
Ishikawa reported an interesting ring expansion by abnormal Bischler-Napieralski reaction (BNR).14a-d The treatment of 44 with POCl3 and successive evaporation and recrystallization of the product gave 45. When the reaction product of 44 with POCl3 was poured into water and successive extraction and chromatographic separation were taken, the compound (46) was formed. The reaction mechanism of the ring expansion on the BNR was discussed.
Aoyama reported a facile synthetic method of 2-azaazulenes by Buchner ring expansion reaction using trimethylsilyldiazomethane (TMSCHN2) and thiobenzoyl isocyanate which was generated from 2-phenylthiazol-4,5-dione (Scheme 17).15a,b The intermediate 48 could be produced by the treatment of 47 in refluxing toluene for 5 min. Addition of TMSCHN2 to the reaction mixture, and the successive treatment with i-Pr2NH produced 49. The treatment of 49 with Rh2(OAc)4 as catalyst gave 2-azaazulene derivative (51) via the intermediate 50.
Intramolecular cyclization was often used to construct a seven-memberd ring. When the thermolysis of 52 was carried out in a sealed tube, 5-styryl-1H-pyrrole (53) was obtained and the compound 54 was not produced. The formation of 54 was achieved by flash vacuum pyrolyses (FVP) of 52 and 53 (Scheme 18).16 The reaction mechanisms of the formation of 53 and 54 were shown in the Scheme 18.
Platinum catalyzed intramolecular cyclization of the alkynes (55) gave 56 as the major product (8% yield), and this method was an efficient synthetic way for 1,5-diazaazulene skeleton (Scheme 19).17
A pyrrole ring is susceptible to an intramolecular nucleophilic attack by organolithiums. Thus the treatment of N-allylpyrrolocarboxamide (57) with LDA yielded azaazulene derivatives (58) by an intramolecular cyclization (Scheme 20).18
Synthetic routes involving Pictet-Spengler condensation or Bischler-Napieralski reaction are known as useful methods for construction of heterocyclic nuclei, and these reactions have been frequently employed for construction of azepine ring.
Previously, Goto reported that the acid-catalyzed condensation of dehydrotryptophan ester (59) with aldehydes gave respective 1,5-diazabenz[cd]azulene derivatives (60) (Scheme 21).19
As application of the reaction, Khashashneh reported that the double Pictet-Spengler condensation of 3-(2,5-diaminophenyl)indole (61) with aldehydes gave 62 (Scheme 22).20
The Bischler-Napieralski reaction of the carboxamides (63) using POCl3 in MeCN under reflux gave 64 (Scheme 23),21a and the reaction of the carboxamides (65) using polyphosphoric acid (PPA) at 140 ºC gave 66 (Scheme 24).21b
II. REACTIONS OF AZAAZULENES
As previously reviewed,1 1-azaazulenes are susceptible to electrophilic attack at nitrogen and C-3 and to nucleophilic attack at C-2 and the seven-membered ring carbons (particularly at C-6 and C-8). It is also known that 1-azaazulen-2(1H)-one shows similar reactivity and is susceptible to an electrophilic attack at nitrogen and C-3.1
A. Reactions with electrophiles
A TFA-catalyzed electrophilic aromatic substitution on the 1-azaazulen-2(1H)-ones (67) with 4-hydroxybenzaldehyde (68) gave the corresponding methane derivatives (69).22 Oxidative hydrogen
abstraction of 69 with DDQ, followed by exchange of the counter anion by using aq. HBF4 or aq. HPF6 and neutralization furnished to the 1,4-benzoquinonemethides (70) (Scheme 25).22a Using similar method, 7,7-bis(heteroazulen-3-yl)-8,8-dicyano-1,4-quinodimethanes were synthesized.22b Similar treatment of 67 with HC(OMe)3 gave tris(heteroazulene)-substituted methane.22c The treatment of bis(1-azaazulen-3-yl)methyl cation with barbituric acid followed by oxidation with o-chloranil gave 5-[bis(heteroazulen-3-yl)methylidene]pirimidine-2,4,6(1H,3H,5H)-triones.22d
Quarternization products of the nitrogen in azaazulenes are important since the produced cations have interesting character,13d,77 and some of them are potential intermediates for azaazulene N-ylides. 2-Alkylamino-1-azaazulenes (71) were alkylated at the N-1 by the treatment with ethyl bromoacetate to give 72, and successive neutralization of 72 with K2CO3 gave 73 (Scheme 26).23
A facile N-trimethylsilylmethylation of 1-azaazulenes (74) with trimethylsilylmethyl triflate (TMSCH2OTf) was presented (Scheme 27). Where the cations (75) were obtained in good yields, and 75 were converted to 76 using CsF.24a,b
B. Reactions with nucleophile
As previously reviewed, a halogen group on azaazulenes can be replaced by a nucleophile.1 Commonly, a mercapto group is more active than an amino group on substitution reaction. Interestingly, when the reaction of 1-azaazulene (77) with 4-amino-3-mercapto-4H-1,2,4-triazoles (78) was undertaken in a refluxing BuOH, N-substitution occurred and yielded 79. When the reaction was performed under basic conditions, S-substitution occurred and yielded 80 (Scheme 28).25 These phenomena could be interpreted as follows. The compound 78 would be existed as thione form (78A), therefore the nucleophilicity of the S-atom decreases and is less reactive than amino group. In the basic conditions, the generated thiolate ion is more reactive than amino group.
The reaction of 77 with PhMgBr followed by dehydrogenation with o-chloranil gave 8-, 6-, and 4-phenyl-1-azaazulenes. The addition occurred in the order of 8 > 4 > 6 in 77.26 The reaction of 2-chloro-1-azaazulenes (81) with aryllithium followed by hydrolysis and dehydrogenation with o-chloranil gave 8-aryl-1-azaazulenes (82) in good yields, regioselectively (Scheme 29).27,28
The reaction of 83 with PyLi gave 84 (58%) and 85 (4%). On the other hand, the reaction of 86a with PyLi and subsequent quenching with MeOH followed by dehydrogenation with o-chloranil gave 87a (30%), 87b (7%), 88a (30%), and 88b (9%). The reaction of 86b with PyLi gave similar result and 87b (26%) and 88b (30%) were obtained (Scheme 30).29 These results show that the coordination of lithium to the ring nitrogen would be essential on regioselectivity.
The treatment of 8-pyridyl-1-azaazulene (83) with an equivalent molar of [Cu(MeCN)4]PF4 gave Cu(II)-83 complex (89), and its structure was identified by X-Ray structure analysis (Figure 2).27
The reaction of 2-chloro-1-azaazulene with phenylethynyl lithium followed by dehydrogenation with o-chloranil gave 8-phenylethynyl-1-azaazulene (90) (13%) and 91 (86%) (Scheme 31).30
C. Coupling reactions
Cross-couplings of nucleophile and electrophile using transition metal catalysts are essentially important and widely applicable methods for precise syntheses.31 In the azaazulene chemistry, the methods, such as Sonogashira-Hagihara reaction, Suzuki coupling, Negishi coupling, Hartwig-Buchwald reaction, etc., were extensively exploited recently.
For the synthesis of 2-(2-pyridyl)-1-azaazulene (92), which induced a specific emission (see section IV), Suzuki coupling, Negishi coupling, and Stille coupling of 2-halo-1-azaazulenes (81: X = Cl, 93a: X = Br, 94: X = I) were took out. Negishi coupling using 2-pyridyl bromide required a greater amount of the palladium catalyst, but when 2-iodo-1-azaazulene (94) was used in the reaction, the highest yield (92%) was provided (Scheme 32).32
Negishi coupling of 93a-c with ZnMe2 in the presence of PdCl2(dppf) gave 2-methyl-1-azaazulenes (95a-c) in good yields (Scheme 33).33
The reaction of 2,3-dibromo-1-azaazulene (95b) occurred at only 2-position. This shows that the bromine at C-2 is more reactive than the one at C-3. Negishi coupling of 3-iodo-1-azaazulen-2(1H)-one (96) with ZnMe2 gave 97 (75%) along with deiodide product (98: 9%) (Scheme 34). Treatment of 97 with POBr3 gave 93c.
Phenylation of halo-1-azaazulenes with PhB(OH)2 by Suzuki coupling proceeded well. In the reaction, it is thought that the bromine at C-2 is more reactive than the one at C-3, as in the case of Negishi coupling. Thus, the reaction of 93b with PhB(OH)2 were taken out and only 99b was obtained in 87% yield (Scheme 35).28
Interestingly, the treatment of 93e with PhB(OH)2 gave 99e (21%) and 100 (31%) together with a recovery of 93e (31%). It seems that phenyl group hastened the reactivity of C-2. But the reaction of 93f with PhB(OH)2 under prolonged reaction time gave only 100 in 83% yield. The result suggests that the bromine at C-3 in 93 hasten the reactivity of C-2. For the synthesis of (1-azaazulen-3-yl)(pinacolate)boran, 93f was treated with bis(pinacolate)diboran [(Bpin)2] in the presence of PdCl2(dppf), and 101 (14%) and bi(1-azaazulene) 102a (57%) were obtained (Scheme 36).28 Produced 101 would rapidly react with 93f under the conditions.
On the occasion of the Suzuki coupling of 93f with PyB(NPDEA), 103a (3%) was isolated together with 86a (50%), and in the reaction of 93g with PyB(NPDEA), the dimer (102b) (6%) was obtained together with 86b (84%) (Scheme 37).29
Introduction of acetylenic moiety on the 1-azaazulene nuclei was achieved successfully by Sonogashira-Hagihara reaction. As similar as the case of Suzuki coupling, 2-bromo-1-azaazulenes and 3-iodo-azaazulenes were easily reacted with acetylenes, and gave corresponding ethynyl-1-azaazulenes (Figure 3).30,34 The reaction of 2-chloro-1-azaazulene (81) with phenylacetylene in the presence of PdCl2(PPh3)2 and CuI did not proceed, but the reaction of 2-bromo-1-azazulene (93a) with phenylacetylene in similar conditions gave 104a in a good yield. The reactivities at C-2 and C-3 were similar to that on the case of Suzuki coupling. Thus the reaction of 93b with phenylacetylene gave 104c. The reaction of 93f with phenylacetylene gave 105b (76%) together with 106 (12%). The reaction of halo-1-azaazulenes with trimethylsilylacetylene afforded similar results, and 104d and 105c were obtained. Desilylation of 104d and 105c using KF gave 104e and 105d in good yields, respectively.34,35
Suzuki coupling of 104e with 93a,35 105d with 93a,35 and 105d with 93f34 gave 107, 108, 109, respectively (Scheme 38).
Glaser coupling of 104e was examined but the reaction did not proceed, and 110 was not obtained. But when 104d was treated with CuCl in DMF, 110 was obtained (Scheme 39).35
On the other hand, the reaction of both 105c and 105d gave 111 in good yields (Scheme 40).34
Sonogashira-Hagihara reaction at seven-membered ring also proceeded. Thus the reaction of 8-bromo-1-azaazulene (112) with phenylacetylene in the presence of PdCl2(PPh3)2 together with CuI gave 113 in 61% yield (Scheme 41).34
At present, 1,2-bis(1-azaazulen-6-yl)acetylene was not synthesized form the 1-azaazulene by similar methods, but it was synthesized via 1,2-bis(1-aminotropon-5-yl)acetylene. Sonogashira-Hagihara reaction of 114 with 115 gave 116, and successive reaction of 116 with diethyl malonate (DEM) in the presence of NaOEt gave 117 (Scheme 42).35
n common with other aromatics, a halogen group in the azaazulenes can be displaced by a nucleophile, and an amino group is easily introduced on the azaazulene nuclei.1 Nevertheless, an introduction of heteroarylamino group on the 1-azaazulene was unsuccessful.36 Hartwig-Buchwald reaction is well known as superior method for the amination of aromatics.37 Therefore, an application of Hartwig-Buchwald reaction was carried out to introduce heteroarylamino group on 1-azaazulene nuclei. The reaction of 77 with heteroarylamines in the presence of Pd2(dba)3, Xantphos, and CsCO3 in dioxane at 120 ºC was undertaken and the corresponding 2-(heteroarylamino)-1-azaaulenes (118) yielded in good yields (Scheme 43).36
This method was also suited for the synthesis of bis(1-azaazulenyl)amines (Scheme 44).38
D. Cycloaddition and Annulation
Cycloadditions of azaazulenes or annulations on azaazulene nuclei are extremely interest from their wide variety of reaction pattern and synthetically use for novel heterocycles.
The reaction of 2-chloro-1-azaazulene (81) with benzyne (123) in CH2Cl2, generated from 124 and TBAF (Kitamura’s method), gave 125 and 126 in low yields together with recovered 81. Similar treatment of 122a gave 125 and 127a, and the treatment of 122b gave only 128 as distinct product. The reaction of 2-chloro-1-azaazulene (81) with benzyne (123), generated from 130 in 1,2-dichloroethane under reflux, gave 126 and 131 (Figure 4).39 Similar reaction of 122a gave 131 and 132. These results suggested that the benzyne, having higher energy, can undergo Diels-Alder type reaction, but the benzyne, having lower energy, takes out Michael type addition.
Cycloaddition of 2-chloro-1-azaazulene (81) with diphenylcyclopropenone (133) showed interesting feature, and gave 134 (4%) and 135 (19%) along with recovered 81 (58%) (Scheme 45).40 The structure of 135 was confirmed by X-Ray structural analysis. At first, an extended dipolar cycloaddition of diphenylcyclopropenone occurred at N-1 and C-8 of 1-azaazulene nuclei, and an intermediate A would be produced. The nucleophilic attack of H2O to A, accompanied with elimination of HCl and dehydrogenation, gave 134. A hydrogen migration of A would give an intermediate B. The cine-substitution of B with A would produce an intermediate C. The cycloheptatriene-norcaradiene interconversion of C affords D. The ring contraction of D attended with elimination of HCl furnishes 135.
Cycloadditions of 1-aza- and 1,3-diaza-azulenium 1-methylides (azaazulene N-ylides) with acetylenic esters were reported (Scheme 46).24a,b The reaction of 2-chloro-1-azaazulenium 1-methylide (76a) with DMAD gave 136 (23%) and 137 (32%). Reaction of 2-methoxy-1-azaazulenium methylode (76b) with DMAD gave 136 (7%), 137 (1%), and 138 (14%). The dipolar cyclization at the carbanion moiety (CH2-) and C-8 of 76 gave 136 (path a) and the dipolar cyclization at the carbanion moiety (CH2-) and C-8a of 76 followed by the rearrangement and hydrogen migration gave 137 (path b), respectively. The reaction of 2-(N-piperidino)-1-azaazulenium 1-methylide (76c) with DMAD gave 138 (22%) as a major product. The selectivities of the reaction site on the cycloaddition of 1-azaazulenium methylides were affected by the nature of the substituent of 76. Although their yields were low, the regioselectivity of the reaction was established by the reaction of 76a with methyl propiolate (MP) as shown in the Scheme 46.24b
Although the compound 73 exists mainly in the imino-form, a potentiality of having a tautomerism with its ylide form 139 raises some investigation. As expected, the treatment of 73 with MP in MeCN for 8 h underwent cycloaddition to give 140 (23%) (Scheme 47).23 The treatment under prolonged reaction time or the reaction in the presence of K2CO3 gave 141 together with 140. The compound 140 was quantitatively converted to 141 by the treatment of 140 with K2CO3 in refluxing MeCN.
The treatment of 73 with acid anhydrides such as acetic anhydride or benzoic anhydride in the presence of K2CO3 gave mesoionic compounds (142) in 90-95% yields (Scheme 48).23
The structure of 142 was confirmed by X-Ray structure analysis. Similar treatment of 73 with succinic anhydride gave 143 (Scheme 49).23
It have been reported that 2-amino-1-azaazulenes reacted with halo- and phenylketenes to give various types of cycloadducts according to the nature of the substituents and the ketenes.41 For comparison with 2-amino-1-azaazulenes, the reaction of 8-amino-1-azaazulene was performed. The treatment of 8-amino-3-phenyl-1-azaazulene (144) with chloroketene gave 145 and 146 in low yields together with 147 (Scheme 50).42 Further treatment of 147 with chloroketene gave 145. It was shown that the compound (146) was produced at the stage of chromatography on the silica gel in the presence of an alcohol. The reaction of 144 with phenylketene gave 145 and 147, but the compound like 146 was not isolated (Scheme 50).
The reaction of 144 with diphenylketene gave 148 (Scheme 51).42
Saito reported interesting [π2 + σ2] and [π8 + σ2] type cycloaddition of 1-azaazulen-2(1H)-one derivatives.43 Naphtho[b]cyclopropene (149) reacted with 1-azaazulen-2(1H)-ones (150) in the presence of ytterbium complex as catalyst in CHCl3 to give [π2 + σ2] type cycloadducts (151) (Scheme 52).
The similar reaction of 149 with 1-thia-3-azaazulen-2(1H)-one (152) gave [π8 + σ2] type cycloadduct (153) (Scheme 53).
It is thought that these reactions proceeded via ionic processes. When the reaction 149 with 150 was performed in benzene, another type of [π8 + σ2] type cycloadducts (154) were obtained via a concerted process (Scheme 54).
Generation and reaction of 7,8-dehydro-1-azaazulene were reported.44 The treatment of 8-bromo-1-azaazulene (155) with t-BuOK in DMSO or in THF in the presence of 16-crown-8-ether generated 7,8-dehydro-1-azaazulene (156), which was trapped with diphenylisobenzofuran (DPIBF) or furan to give 157 or 158 and 159 (Scheme 55). Interestingly, when the reaction was performed in DMSO, the compound 160 was obxy-product.
Reductive cyclization of 8-(2-nitrophenyl)-1-azaazulenes (161) using P(OEt)3 or P(NMe2)3 produced diazanaphth[3,2,1-cd]azulenes (162) and 7H-1,7-diazaindeno[1,2-e]azulenes (163) via the nitrene (164) (Scheme 56).45
Tautomerisation between 163 and 165 was investigated; 163 was converted to 165 by the treatment of aq. KOH followed by aq. HCl, and 165 was converted to 163 at rt with a half-life period of 20 d in CDCl3 (Scheme 57).
E. Aza-Wittig reaction
The aza-Wittig reaction of iminophosphoranes is one of the most useful methodologies for the synthesis of nitrogen-containing heterocycles.6,46 The aza-Wittig reactions of phosphoimino-azaazulene derivatives were reported by Nitta.47-49 and Abe,50-54 independently. Synthesis of 2-phosphoimino-1-azaazulenes, 2-phosphoimino-1,3-diazaazulene, and 8-phosphoimino-1-azaazulene were achieved by four different methods using A) Staudinger reaction,46,47,55 B) Mitsunobu conditions,48,56 C) Kirsanov reaction,50,52,57 and D) Appel reaction.52,54,58
At first, Nitta et al. synthesized 2-phosphoimino-1-azaazulene (168) from 2-azido-1-azaazulene (167), prepared from 2-hydrazino-1-azaazulene (166), by Staudinger reaction (Scheme 58). By the reaction of 168 with 2-bromotropone, they synthesized 169.47
2-Phosphoimino-1,3-diazaazulene (171a) and 2-phosphoimino-1-azaazulenes (171b-d) were synthesized from corresponding 2-amino-1,3-diazaazulene (170a) and 2-amino-1-azaazulenes (170b-d) by three ways (Method B,48 Method C,52 and Method D52) as shown in the Scheme 59.
The reaction of 171 with aryl isocyanates gave 172, being produced as aza-Wittig reaction products, and 173, being produced as abnormal aza-Wittig reaction products, along with 174.48,49,52 At lower temperature (rt ~ 60 ºC), 172 and 174 were obtained. Whereas the reaction was carried out at elevated temperature (benzene reflux ~ xylene reflux), 173 was obtained together with 172 and 174. The reaction would proceed as shown in the Scheme 60.
Aryl isothiocyanates also reacted with 171b and to give cycloadducts (175) (Scheme 61).52,59
2-Phosphoimino-1-azaazulene (171c) reacted with aryl aldehydes and to give 176 (Scheme 62).59 In the reaction, the Schiff base (177) was not isolated. Addition of small amount of 2-amino-1-azaazulene (170c) accelerated the reaction. The results suggested that 170c, produced by hydrolysis of 171, easily reacted with 177.
3-Ethynyl-2-phosphoimino-1-azaazulene (178) was synthesized from 2-amino-3-ethynyl-1-azaazulene by Appel reaction.54 When 178 was treated with Cu(OTf)2, no cyclization product was isolated; instead, compounds (179, 180, and 181) were obtained (Scheme 63).54 The reaction of 178 with aryl isocyanate gave similar results in the case of 171 as shown in Scheme 60. The treatment of 178 with aryl isocyanate in the presence of benzoyl peroxide gave a cyclization product in low yield.54
8-Phosphoimino-1-azaazulene (182) was synthesized from 8-amino-1-azaazulene (183) by the Kirsanov reaction (Scheme 64).50 The structure of 182 was determined by X-Ray structure analysis (Figure 5). The bond distance between N(2) and P(1) is 2.762(6) Å, which is rather short from the value of van der Waals radii (3.4 Å). The bond distance between N(1) and P(1) is 1.597(2) Å and the bond distance between N(1) and C(1) is 1.350(2) Å; these can regard as intermediate value between single bonds and double bonds. Therefore, the existence of the resonance between the formulas 182 and 182A can be presumed.
Some reactions of 182 were examined. The aza-Wittig reaction of 182 with aryl aldehydes proceeded, and following cyclization and hydrogen migration gave 184 (Scheme 64).51
The reaction of 182 with aryl isocyanates gave the cyclization products (185) together with 186 (Scheme 65).53
F. Electrochemical reactions and photoreactions.
Electrochemical oxidation of 1-aryl-3-tosyl-1,2,3,3a-tetrahydro-1,3-diazaazulen-2-ones (187) gave 6-tosyl-1-aryl-1,2,3,6-tetrahaydro-1,3-diazaazulen-2-ones (188) via migrations of the tosyl group.60a Circumstantial examination of the electrochemical oxidation of 187 showed that 189 was produced at first, and successive electrolysis of 189 gave 188 (Scheme 66).60b Electrochemical oxidation of 188 resulted in the recoveries of the starting material (188). On the other hand, electrochemical reductions of 187 gave 1-aryl-1,2,3,3a-tetrahydro-1,3-diazaazulen-2-ones (190) through eliminations of the tosyl group (Scheme 66).60a
Electrochemical oxidation of 1,2,3,3a-tetrahydro-1-azaazulen-2-ones (191), possessing a spirocyclic moiety, afforded 1,3-diaryl-1,2,8,8a-tetrahydro-1-azaazulen-2-ones (192) and 1,3-diaryl-1,2,3,3a-tetrahydro-1-azaazulen-2-ones (193) together with 1,3-diaryl-1-azaazulen-2(1H)-ones (194) (Scheme 67).61
The photoreaction of 187 showed a solvent effect. The reaction in THF gave ion pairs (195) between p-toluenesulfonate anion and 1-aryl-2,3-dihydro-1,3-diazaazulen-2(1H)-one cations. On the other hand, the reaction in polar solvent afforded 196.62
In CV measurements, the reduction waves of quinonemethides (70) were reversible, suggesting a stabilizing effect of heteroazulenes toward the radical anions (197) and dianions (198). Quinonemethides (70) showed two oxidation waves owing the formation of radical cations (199) and dications (200), and the first oxidation potential of 70 (R1 = t-Bu) is reversible (Scheme 69).22a
In the CV of 202, two reversible reduction waves (E1red -1.07 ~ -1.13 V, E2red -1.68 ~ -1.70 V) are observed, indicating the stabilizing ability of 1-azaazulenes toward the corresponding radical anions (203) and dianions (204), respectively (Scheme 70).22d Furthermore, 202 exhibits one irreversible oxidation wave (E1ox +0.77 ~ +0.81 V) owing to the formation of radical cations (205) and the corresponding reduction wave appearing in far negative region (+0.08~0.10 V), which suggested a conformational change in the radical cations during the redox process.22d
The electrochemical reduction of the cations (206) exhibits two reversible waves and low reduction potentials at -0.58 (R = Ph) and -0.62 (R = Me) (E1red) (V vs. Ag/Ag+) owing to the formation of radicals (207) and -1.27 (R = Ph) and -1.33 (R = Me) (E2red) owing to the formation of anions (208) upon CV, whose values are lower than that of tris(azulen-1-yl)methyl cations (Scheme 71).22c
As mentioned as above, 6-aminouracil fused 1-azaazulenium ions (209) were easily synthesized form 33 (Scheme 72).13d The electrochemical reduction of 209 exhibited high reduction potentials at -0.84 (R = Ph) and -0.87 (R= Me) (V vs. Ag/Ag+) upon CV. A good linear correlation between the pKR+ values and reduction potentials (E1red) of 209 was obtained.13d The photo-induced oxidation reaction of 209 toward some alcohols under aerobic conditions afforded the corresponding carbonyl compounds in more than 100% yield, suggesting the oxidizing function of 209 toward alcohols in the autorecycling process.13d Similar photo-induced autorecycling oxidizing reaction of 209 prepared by methylation of 33, 39-43 and related compounds toward some amines were also reported.13c,13e,13f,48,49
III. BIOLOGICAL ACTIVITIES
In the research of biological activities, it has been reported that azaazulene derivatives have a wide variety of activities.1a,1c 1-Azaazulene derivatives (5: R1 = H, NH2, OH, etc.; R2, R3 = Ph, naphthyl, furyl, etc.) were useful for treating diseases caused by unregulated p38 mitogen-activated protein kinase (MAPK) or tumor necrosis factor-alpha (TNF-alpha) activity. E.g., the compound (5: R1 = H; R2 = 4-pyridinyl; R3 = 4-FC6H4) showed IC50 of 1340 nM and 230 nM against p38 MAPK and TNF-alpha, respectively.3 Detailed researches about the inhibitor activities against p38 MAPK and c-Jun N-terminal kinase 2-alpha (JNK2α) of 1-azaazulenes (5a,5b) were made (Figure 6).63 It is shown that the azaazulene class of inhibitors will be useful to decipher cellular actions of p38 MAPK (5a: IC50 = 0.6 µM, 5b: IC50 = 4.7 µM) and JNK2α (5a: IC50 = 3.5 µM).
It is known that azaazulene derivatives showed the utility as antitumor agents.14d For inquiry of new antitumor agents, cytotoxic activities of 1-azaazulene derivatives against HeLa S3 cells were investigated. 3-Benzothiazolyl-1-azaazulene (211: X = Cl, Y = S; IC50 = 5.3 µM), which was obtained by the condensation of 2-chloro-3-formyl-1-azaazulene (210) with o-aminothiophenol (Scheme 73), showed strong cytotoxic activity against HeLa S3 cells.64 Some resemble compounds (211) also showed cytotoxic activity against HeLa S3 cells.64
Some 2-(heteroarylamino)-1-azaazulenes showed cytotoxic activity against HeLa S3 cells (E.g., 118a: IC50 = 6.5 µM, 118b: IC50 = 23.3 µM) (Figure 7).36
Azaazulenotropones (214: R1 = H, OMe, CH2Ph, OAc, CO2Et, R2 = H, CO2Et) and azaazulenotropolone (214: R1 = OH, R2 = H) were synthesized by the condensation of 2,3-diformyl-1-azaazulene (213), prepared from 3-formyl-2-styryl-1-azaazulene (212), with acetone derivatives (Scheme 74).65 The IC50 value of azaazulenotropolone (214: R1 = OH, R2 = H) against HeLa S3 cells was 19 µM.65
Azulene and azaazulene have characteristic photo-activity. Therefore, introduction of these molecules on bioactive compounds of known structure and function is expected to direct integrated photo-active molecules which the original biological activity is retained. Thus, analogs of peptides containing 1-azaazulene were synthesized by the replacement of tyrosine by azaazulene moiety (Figure 8). The integrated photo-active peptides and psuedopeptides could be used in a medical phototherapy.66
Two novel antimitotic heterocyclic alkaloids, ceratamines A (215a) and B (215b), have been isolated from the marine sponge Pseudoceratanina sp., collected in Papua New Guinea (Figure 9). The IC50 values of 215a and 215 were 10 µM in the cell-based antimitotic assay.67
The 3a,10-diazabenz[f]azulene (pyrrolo[1,2-c][1,4]benzodiazepine: PBD) ring system, a group of potent naturally occurring antitumor antibiotics from Streptomyces species, are of considerable interest for their potentiality as antitumor agents, gene regulators, and DNA probs. Although many synthetically and pharmacological investigations related to PBD and its analogs were reported (especially, D. E. Thurston group and A. Kamal group have been investigating ardently),68,69 only some examples, about the compounds showing bioactivity, were described here. Three novel C2-aryl substituted PBDs (216a,b,c) have been synthesized and evaluated in a number of cell lines revealing selective cytotoxicity at the sub-nano-molar level towards melanoma and ovarian cancer cell lines (Figure 10).70 Cytotoxic compounds (217) were synthesized and evaluated in the human leukemic K562 cell line and were shown to have micromolar potency (Figure 10. 217a: IC50 = 18 µM, 217b: IC50 = 30 µM).71
Compounds (Figure 11. 64a-e) showed weak binding affinities to the bovine dopamine D1 receptor and the human dopamine D2long, D2short, D3 and D4 receptor expressed by the ability to displace the corresponding radioligands only incomplete at micrometer concentration.21a
IV. THEORETICAL AND STRUCTURAL STUDIES.
AM1 calculations with complete optimization have been carried out on azulene, 1-azaazulene, and 1,3-diazaazulene.72 The dipole moment values were estimated (1-azaazulene: 3.05 D, 1,3-diazaazulene: 4.40 D), the latter coincided with experimental data (1,3-diazaazulene: 4.39 D) by Kurita and Kubo.73
Determination of most stable geometrical conformations and some spectroscopic values were also reported.
Electronic properties of 1,3-diazaazulenes in ground and excited states were characterized by using high level quantum chemistry methods (CASSCF/cc-pvdz).74 The S0-S1 energy transition in 1,3-diazaazulene (2.77 eV) is noticeably larger than that in the parent azulene (1.97 eV). The difference in dipole moments in the ground and excited states for 1,3-diazaazulene is about twice larger than that for azulene, and presumably, its derivatives, influencing the second order non-linear optical responses of corresponding substances. The values of S0-S1 excitation energies of the 1,3-diazaazulene derivatives by direct calculations are in a reasonable agreement with experimental data.
2-(2-Pyridyl)-1-azaazulene (92) exhibits relatively strong basicity (pKa1 = 6.02, pKa2 = 2.42) and showed interesting behavior in the emission spectra. The free base (92) in MeCN showed an emission, assumed to be S2→S0 relaxation, at 434 nm upon excitation of 368 nm and no emission upon excitation of the longest wavelength maximum of 512 nm. On the other hand, the diprotonated species of 92 in 50% H2SO4 exhibited an emission of 484 nm upon excitation of the longest wavelength maximum of 429 nm, assumed to be S1→S0 relaxation. The absorption and emission spectra of 92 were also found dependent on a metal cation present in a solution. In the presence of Mg2+, an emission at 543 nm from the S1 state was observed upon excitation of 478 nm, but in the presence of Na+, no emissions was observed from the S1 state but an emission from the S2 state was observed.32
It is decided by X-Ray crystal structure analysis that the compound (218) exists in the crystal in its keto form as 3-phenylcyclohepta[b]pyrrol-8(1H)-one (218) rather than in the enol form as 8-hydroxy-3-phenyl-1-azaazulene (219) (Scheme 75).75
The tautomerization of 8-amino-1-azaazulenes was investigated by X-Ray crystal structure analysis and molecular orbital calculation (Gaussian 98 using RHF/6-31G*).53,59 The results showed that 8-aryl amino-1-azaazulene favored in imino-form (220a), whereas 8-methylamino-1-azaazulene favored in aminoform (221b). The results can be explained as follows: an aryl group causes stabilization of azaheptafulvene moiety by the conjugation with lone pair on the imino-nitrogen, while an electron-donating methyl group bring down the instability to azaheptafulvene moiety and increasing electron density of amino-nitrogen.
1-Methyl-6,7-dihydropyrrolo[2,3-c]azepine-4,8(1H,5H)-dione (222) was synthesized. The X-ray crystal structure analysis of 222 showed that intermolecular N-H···O hydrogen bonds generate a one-dimensional chain (Figure 12).76
2-Azaazulenium cation and monomethine cyanine dyes bearing 2-azaazulenium moieties (227) were synthesized as a series of novel monomethine cyanine dyes as shown in Scheme 77.77 The key products (223 and 224) were synthesized by known methods.78 Compounds (225 and 226) were prepared also known methods.79 The series of symmetrical and asymmetrical monomethine cyanine dyes (227) were synthesized by conventional cyanine chemistry synthetic approaches. Combined spectral and quantum chemical investigations of their molecular geometries and their electronic structures and the nature of their lowest electron transitions have been performed. Analysis of quantum chemical calculation and experimental spectroscopic data has shown that there is practically no difference in charge distribution over the π electron system in the ground state in 224 and in 227. This would be attributed to the charge distribution which is not dependent on the relative location of MOs. The spectral properties, and correspondingly the nature of electron transitions in the chromophores, of compounds (224 and 227) are drastically different because of the different mechanisms of generation of the HOMOs.
CONCLUSION
In recent studies of azaazulene chemistry, some new synthetic methods were evolved. In addition, a variety of methodologies for functionalization of azaaulenes, such as transition metal catalyzed coupling reaction or cycloaddition and so on, were applied, and a lot of novel compounds having various patterns of the skeletons. In the azaazulene chemistry, biological and pharmaceutical pursuits are prominent. We expect the further development about azaazulene chemistry.
References
1. For reviews see, a) N. Abe, ‘Recent Research Developments in Organic and Bioorganic Chemistry’, 2001, 4, 14, Transworld Research Network; b) N. Abe, ‘Trends in Heterocyclic Chemistry’, 2001, 7, 25, Research Trends; c) T. Nishiwaki and N. Abe, Heterocycles, 1981, 15, 547; CrossRef d) M. Kimura, Yuki Gosei Kagaku Kyokai Shi, 1981, 39, 690.
2. N. Sakai, N. Hattori, N. Tomizawa, N. Abe, and T. Konakahara, Heterocycles, 2005, 65, 2799. CrossRef
3. R. J. Sciotti and R. Wagner, PCT Int. Appl., 2001, WO2001057018.
4. a) M. Kitamura, S. Chiba, O. Saku, and K. Narasaka, Chem. Lett., 2002, 606; CrossRef b) M. Kitamura and K. Narasaka, Chem. Rec., 2002, 2, 268; CrossRef c) S. Chiba, M. Kitamura, O. Saku, and K. Narasaka, Bull. Chem. Soc. Jpn., 2004, 77, 785. CrossRef
5. Y. Matsumoto and M. Nitta, Heterocycles, 2001, 55, 2131. CrossRef
6. M. Nitta, Rev. Heteroatom Chem., 1993, 9, 1197.
7. a) H. Yamamoto, M. Ohnuma, and M. Nitta, J. Chem. Res. (S), 1999, 173; H. Yamamoto, M. Ohnuma, and M. Nitta, J. Chem. Res. (M), 1999, 901; b) M. Nitta and Y. Mitsumoto, Heterocycles, 2001, 55, 629; CrossRef c) M. Nitta, Y. Mitsumoto, and H. Yamamoto, J. Chem. Soc., Perkin Trans. 1, 2001, 1901. CrossRef
8. a) M. Nitta and S. Naya, J. Chem. Res. (S), 1998, 522; M. Nitta and S. Naya, J. Chem. Res. (M), 1998, 2363; b) S. Naya and M. Nitta, J. Chem. Soc., Perkin Trans. 2, 2002, 1017; CrossRef c) Y. Mistsumoto and M. Nitta, J. Chem. Soc., Perkin Trans. 1, 2002, 2268. CrossRef
9. K. Ito and K. Saito, ‘Recent Research Development in Pure & Applied Chemistry’, 1999, 3, 91, Transworld Research Network.
10. K. Takaoka, T. Aoyama, and T. Shioiri, Heterocycles, 2001, 54, 209. CrossRef
11. V. Nair and K. G. Abhilash, Tetrahedron Lett., 2006, 47, 8707. CrossRef
12. O. Sato, K. Tsurumaki, S. Tamaru, and J. Tsunetsugu, Heterocycles, 2004, 62, 535. CrossRef
13. a) M. Nitta and Y. Tajima, Synthesis, 2000, 651; CrossRef b) S. Naya, Y. Iida, and M. Nitta, Tetrahedron, 2004, 60, 459; CrossRef c) S. Naya and M. Nitta, Tetrahedron, 2004, 60, 9139; CrossRef d) S. Naya and M. Nitta, Tetrahedron, 2003, 59, 7291; CrossRef e) S. Naya, H. Ohtoshi, and M. Nitta, J. Org. Chem., 2006, 71, 176; CrossRef f) S. Naya, J. Nishimura, and M. Nitta, J. Org. Chem., 2005, 70, 9780. CrossRef
14. a) T. Ishikawa, T. Saito, S. Noguchi, H. Ishii, S. Ito, and T. Hata, Tetrahedron Lett., 1995, 36, 2795; CrossRef b) T. Ishikawa, K. Shimooka, T. Narioka, S. Noguchi, T. Saito, A. Ishikawa, E. Yamazaki, T. Harayama, H. Seki, and N. Yamaguchi, J. Org. Chem., 2000, 65, 9143; CrossRef c) T. Narioka and T. Ishikawa, Heterocycles, 2002, 56, 413; CrossRef d) T. Ishikawa and A. Zeimoto, Jpn. Kokai Tokkyo Koho, 1999, JP 11,255,746.
15. a) M. Morita, Y. Hari, T. Iguchi, and T. Aoyama, Tetrahedron, 2008, 64, 1753; CrossRef b) Y. Hari, T. Shioiri, and T. Aoyama, Yuki Gosei Kagaku Kyokai Shi, 2009, 67, 357.
16. T. M. V. D. Pinho e Melo, M. I. L. Soares, A. M. d’A. Rocha Gonsalves, and H. McNab, Tetrahedron Lett., 2004, 45, 3889. CrossRef
17. M. Gruit, D. Michalik, A. Tillack, and M. Beller, Angew. Chem. Int. Ed., 2009, 48, 7212. CrossRef
18. J. Clayden, R. Turnbull, and I. Pinto, Org. Lett., 2004, 6, 609. CrossRef
19. S. Nakatsuka, H. Miyazaki, and T. Goto, Tetrahedron Lett., 1986, 27, 4757. CrossRef
20. A. M. Al-Khashashneh, M. M. El-Abadelah, and R. Boese, Heterocycles, 2003, 60, 73. CrossRef
21. a) P. Gmeiner, H. Hübner, K. A. Abu Safieh, I. I. Fasfous, M. M. El-Abadelah, S. S. Sabri, and W. Voelter, Heterocycles, 2003, 60, 1339; CrossRef b) K. A. Abu Safieh, F. H. Darras, M. T. Ayoub, M. M. El-Abadelah, S. S. Sabri, and W. Voelter, Heterocycles, 2007, 71, 2681. CrossRef
22. a) S. Naya, T. Watano, and M. Nitta, Bull. Chem. Soc. Jpn., 2003, 76, 2035; CrossRef b) S. Naya, K. Yoda, and M. Nitta, Tetrahedron, 2004, 60, 4953; CrossRef c) S. Naya and M. Nitta, J. Chem. Soc., Perkin Trans. 1, 2000, 2777; CrossRef d) S. Naya, K. Yoda, and M. Nitta, Tetrahedron, 2005, 61, 8616. CrossRef
23. N. Abe, Y. Fukumoto, H. Fujii, and A. Kakehi, Heterocycles, 2002, 56, 171. CrossRef
24. a) H. Fujii, K. Iwafuji, Y. Sawae, N. Abe, and A. Kakehi, Heterocycles, 2003, 59, 63; CrossRef b) H. Fujii, I. Kawano, K. Iwafuji, Y. Sawae, K. Nagamatsu, and N. Abe, Heterocycles, 2006, 70, 207. CrossRef
25. E. Yoshioka, K. Koizumi, S. Yamazaki, H. Fujii, and N. Abe, Heterocycles, 2009, 78, 3065. CrossRef
26. T. Morita, N. Abe, and K. Takase, J. Chem. Soc., Perkin Trans. 1, 2000, 3063. CrossRef
27. N. Abe, E. Hashimoto, H. Fujii, Y. Murakami, S. Tagashira, and A. Kakehi, Heterocycles, 2004, 63, 2341. CrossRef
28. N. Abe, M. Tanaka, T. Maeda, H. Fujii, and A. Kakehi, Heterocycles, 2005, 66, 229. CrossRef
29. T. Ariyoshi, T. Noda, S. Watarai, S. Tagashira, Y. Murakami, H. Fujii, and N. Abe, Heterocycles, 2009, 77, 565. CrossRef
30. H. Fujii, N. Abe, N. Umeda, and A. Kakehi, Heterocycles, 2002, 58, 283. CrossRef
31. a) ‘Topics in Current Chemistry’ Vol. 219, ed. by N. Miyaura, Springer, 2002; b) ‘Metal-Catalyzed Cross-Coupling Reactions’, ed. by F. Diederich and P. J. Stang, Willey-VCH, 1998; c) J. Hassan, M. Sévignon, C. Gozzi, E. Schulz., and M. Lemaire, Chem. Rev., 2002. 102, 1359. CrossRef
32. M. Oda, K. Ogura, N. C. Thanh, S. Kishi, S. Kuroda, K. Fujimori, T. Noda, and N. Abe, Tetrahedron Lett., 2007, 48, 4471. CrossRef
33. K. Koizumi, C. Miyake, T. Ariyoshi, K. Umeda, N. Yamauchi, S. Tagashira, Y. Murakami, H. Fujii, and N. Abe, Heterocycles, 2007, 73, 325. CrossRef
34. N. Abe, Y. Harada, Y. Imachi, H. Fujii, A. Kakehi, and M. Shiro, Heterocycles, 2007, 72, 459. CrossRef
35. Y. Harada, H. Fujii, and N. Abe, unpublished results.
36. K. Koizumi, K. Shimabara, A. Takemoto, S. Yamazaki, N. Yamauchi, H. Fujii, M. Kurosawa, T. Konakahara, and N. Abe, Heterocycles, 2009, 79, 319. CrossRef
37. a) J. F. Hartwig, Angew. Chem. Int. Ed., 1998, 37, 2046; CrossRef b) J. P. Wolfe, S. Wagaw, J.-F. Marcoux, and S. L. Buchwald, Acc. Chem. Res., 1998, 31, 805; CrossRef c) B. H. Yan and S. L. Buchward, J. Organomet. Chem., 1999, 576, 125; CrossRef d) D. Prim, J.-M. Campagne, D. Joseph, and B. Andrioletti, Tetrahedron, 2002, 58, 2041. CrossRef
38. E. Yoshioka, K. Koizumi, S. Yamazaki, H. Fujii, and N. Abe, Heterocycles, 2009, 78, 3065. CrossRef
39. N. Abe, M. Mori, and H. Fujii. J. Chem. Res. (S), 2002, 8; N. Abe, M. Mori, and H. Fujii. J. Chem. Res. (M), 2002, 0162-0172.
40. N. Abe, H. Fujii, and A. Kakehi, Heterocycles, 2001, 55, 1189. CrossRef
41. N. Abe, I. Osaki, S. Kojima, H. Matsuda, Y. Sugihara, and A. Kakehi, J. Chem. Soc., Perkin Trans. 1, 1996, 2351. CrossRef
42. N. Abe, A. Otani, T. Nagai, and H. Fujii, Heterocycles, 2003, 59, 199. CrossRef
43. K. Saito, N. Ito, and S. Ando, Heterocycles, 2002, 56, 59. CrossRef
44. N. Abe, M. Yoshitsugu, H. Fujii, and A. Kakehi, Heterocycles, 2003, 61, 237. CrossRef
45. N. Abe, M. Nabeshima, H. Fujii, A. Kakehi, Y. Kageura, and T. Konakahara, Heterocycles, 2001, 54, 329. CrossRef
46. For reviews: a) Y. G. Gololobov and L. F. Kasukhin, Tetrahedron, 1992, 48, 1353; CrossRef b) S. Eguchi, Y. Maatsushita, and K. Yamashita, Org. Prep. Proced. Int., 1992, 24, 209; CrossRef c) P. Molina and M. J. Viapana, Synthesis, 1994, 1197; CrossRef d) H. Wamhoff, G. Richardt, and S. Stolben, Adv. Heterocycl. Chem., 1995, 64, 159. CrossRef
47. M. Nitta, Y. Iino, T. Sugiyama, and A. Akaogi, Tetrahedron Lett., 1993, 34, 831. CrossRef
48. M. Nitta, D. Ohtsuki, Y. Mitsumoto, and S. Naya, Tetrahedron, 2005, 61, 6073. CrossRef
49. M. Nitta, T. Morito, Y. Mitsumoto, and S. Naya, Heterocycles, 2005, 65, 1629. CrossRef
50. N. Abe, H. Fujii, K. Tahara, and M. Shiro, Heterocycles, 2001, 55, 1659. CrossRef
51. N. Abe, K. Nagamatsu, K. Tahara, and H. Fujii, Heterocycles, 2004, 63, 809. CrossRef
52. K. Nagamatsu, A. Serita, J.-H. Zeng, H. Fujii, N. Abe, and A. Kakehi, Heterocycles, 2006, 67, 337. CrossRef
53. K. Nagamatsu, H. Fujii, N. Abe, and A. Kakehi, Heterocycles, 2004, 64, 291. CrossRef
54. N. Abe, K. Nagamatsu, T. Ariyoshi, H. Fujii, Y. Murakami, S. Tagashira, and A. Kakehi, Heterocycles, 2007, 74, 309. CrossRef
55. Y. G. Golovov, I. N. Zhmurova, and L. F. Kasukin, Tetrahedron, 1981, 37, 437. CrossRef
56. C. E. Hoest, A. Nefzi, and R. A. Houghten, Tetrahedron Lett., 2003, 3705. CrossRef
57. a) A. V. Kirsanov, Izv. Acad. Nauk. SSSR, Otd., Kim. Nauk, 1950, 426; b) G. I. Derkah, E. S. Gubitskaya, V. A. Shokol, and A. V. Kirsanov, Zh. Obsh. Kim., 1962, 32, 1201.
58. a) R. Appel and M. Halstenberg, ‘Organophosphorus Reagents in Organic Synthesis’, ed. by J. I. G. Cadogan, Academic Press, London, 1979; b) T. Okawa, M. Toda, S. Eguchi, and A. Kakehi, Synthesis, 1998, 1467; CrossRef c) T. Okawa, M. Kawase, and S. Eguchi, Synthesis, 1998, 1185. CrossRef
59. K. Nagamatsu, Doctor Thesis, Yamaguchi University, 2007.
60. a) K. Saito, T. Ido, Y. Awada, T. Kanie, and S. Kondo, Heterocycles, 2000, 53, 519; CrossRef b) K. Saito, Y. Ueda, A. Kawamura, A. Kajita, H. Ishiguro, T. Ido, K. Ono, and Y. Awadu, Heterocycles, 2002, 58, 63. CrossRef
61. K. Saito and R. Ueno, Heterocycles, 2002, 57, 219. CrossRef
62. K. Saito, T. Kunisada, H. Ishiguro, M. Sato, and T. Doi, Heterocycles, 2001, 55, 2315. CrossRef
63. R. Somwar, S. Koterski, G. Sweeney, R. Sciotti, S. Djuric, C. Berg, J. Trevillyan, P. E. Scherer, C. M. Rondinone, and A. Klip, J. Biol. Chem., 2002, 277, 50386. CrossRef
64. N. Yamauchi, H. Fujii, A. Kakehi, M. Shiro, M. Kurosawa, T. Konakahara, and N. Abe, Heterocycles, 2008, 76, 617. CrossRef
65. T. Ariyoshi, K. Yoshinaga, K. Koizumi, H. Fujii, R. Ikeda, T. Konakahara, and N. Abe, Heterocycles, 2010, 80, 427. CrossRef
66. R. Rajagopalan, W. L. Neumann, and R. B. Dorshow, PCT Int. Appl., 2001, WO2008108941.
67. E. Manzo, R. van Soest, L. Matainaho, M. Roberge, and R. J. Andersen, Org. Lett., 2003, 5, 4591. CrossRef
68. a) D. E. Thurston, Advanced in the study of Pyrrolo[2,1-c][1,4]benzodiazepine (PBD) Antitumour Antibiotics, in ‘Molecular Aspects of Anticancer Drug-DNA Interactions’, ed. by S. Neidle and M. Waring, Macmillan Press Ltd., London, 1993, pp. 54-58; b) A. Kamal, M. V. Rao, N. Laxman, G. Ramesh, and G. S. K. Reddy, Curr. Med. Chem.: Anti-Cancer Agents, 2002, 2, 215; CrossRef c) S. J. Gregson, P. W. Howard, J. A. Hartley, N. A. Brooks, L. J. Adams, T. C. Jenkins, L. R. Kelland, and D. E. Thurston, J. Med. Chem., 2001, 44. 737; CrossRef d) D. E. Thurston and D. S. Bose, Chem. Rev., 1994, 94, 433; CrossRef e) D. E. Thurston, ‘Chemistry and Pharmacology of Anticancer Drugs’ CRC Press, 2006; f) A. Kamal, R. Rajender, D. R. Reddy, M. K. Reddy, G. Balalishan, T. B. Shaik, M. Chourasia, and G. N. Sastry, Bioorg. Med. Chem., 2009, 17, 1557; CrossRef g) A. Kamal, K. L. Reddy, G. S. K. Reddy, and B. S. N. Reddy, Tetrahedlon Lett., 2004, 45, 3499; CrossRef h) M. Kotecha, J. Kluza, G. Wells, C. C. O’Hare, C. Forni, R. Mantovani, P. W. Howard, P. Mollis, D. E. Thurston, J. A. Hartley, and D. Hochhauser, Mol. Cancer Ther. 2008, 7, 1319; CrossRef i) P. W. Haward, Z. Chen, S. J. Gregson, L. A. Masterson, A. C. Tiberghien, N. Cooper, M. Fang, M. J. Coffils, S. Klee, J. A. Hartley, and D. E. Thurston, Bioorg. Med. Chem. Lett., 2009, 19, 6463. CrossRef
69. D. Antonow, M. Kaliszczak, G.-D. Kang, M. Coffils, A. C. Tiberghien, N. Cooper, T. Barata, S. Heidelberger, C. H. James, M. Zloh, T. C. Jenkins, A. P. Reszka, S. Neidle, S. M. Guichard, D. I. Jodrell, J. A. Hartley, P. W. Howard, and D. E. Thurston, J. Med. Chem., 2010, 53, 2927, and references cited therein. CrossRef
70. N. Cooper, D. R. Hagan, A. Tiberghien, T. Ademefun, C. S. Matthews, P. W. Howard, and D. E. Thurston, Chem. Commun., 2002, 1764. CrossRef
71. A. Tsotinis, M. Vlachou, K. Kiakos, J. A. Hartley, and D. E. Thurston, Chem. Lett., 2003, 32, 512. CrossRef
72. K. Elblidi, A. Boucetta, B. Benali, A. Kadiri, C. Cazeau-Dubroca, G. Nouchi, and M. Pesquer, Theo. Chem., 1995, 343, 57.
73. Y. Kurita and M. Kubo, J. Am. Chem. Soc., 1957, 79, 5460. CrossRef
74. K. B. Bravaya, B. L. Grigorenco, A. V. Nemukhin, Y.-J. Zhu, and J.-P. Zhang, Theo. Chem., 2008, 855, 40.
75. K. Kubo, T. Tsujimoto, and A. Mori, Acta Cryst., 2001, E57, 0225.
76. X.-C. Zeng, S.-H. Xu, J. Gu, and D.-S. Deng, Acta Cryst., 2005, E61, 0795.
77. J. Bricks, A. Ryabitskii, and A. Kachkovskii, Eur. J. Org. Chem., 2009, 3439. CrossRef
78. J. L. Bricks and N. N. Romanov, Khim. Hetero. Soed. (Russ.), 1994, 193.
79. G. Seitz, R. A. Olsen, T. Kӓmpchen, and R. Matusch, Chem. Ber., 1979, 112, 2087. CrossRef