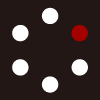
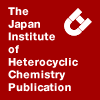
HETEROCYCLES
An International Journal for Reviews and Communications in Heterocyclic ChemistryWeb Edition ISSN: 1881-0942
Published online by The Japan Institute of Heterocyclic Chemistry
e-Journal
Full Text HTML
Received, 25th June, 2010, Accepted, 27th July, 2010, Published online, 29th July, 2010.
DOI: 10.3987/COM-10-S(E)71
■ A Facile Synthesis of Fluorine-Containing 1,7-Phenanthrolines by the Cyclization of N-Propargyl-6,8-bis(trifluoroacetyl)quinolin-5-amine with Various Active Methylene Compounds
Dai Shibata, Ayaka Sakai, Mizuki Hatakenaka, Shohei Saikawa, Yasuhiro Kamitori, Maurice Médebielle, and Etsuji Okada*
Department of Chemical Science and Engineering, Graduate School of Engineering, Kobe University, 1-1 Rokkodai-cho, Nada-ku, Kobe 657-8501, Japan
Abstract
The reaction of N-propargyl-6,8-bis(trifluoroacetyl)quinolin-5-amine (1) with various active methylene compounds gave the novel fluorine-containing 1,7-phenanthroline derivatives (3). Furthermore, changing the electron-withdrawing groups on active methylene compounds induced interesting alternation of the reaction site wherein the enolate anions attack first and led to construction of the different nitrogen-containing heterocyclic systems, 1,7-phenanthrolinone (4) and 1,4-dihydro-1,7-phenanthroline (5).INTRODUCTION
1,7-Phenanthroline and the related derivatives have been extensively studied because of their promising biological activities, such as topoisomerase I inhibitors with cytotoxic properties towards L1210 murine leukemia cells,1 antimalarials,2 and telomerase inhibitors.3 Besides, their application in host-guest chemistry has also been found.4 Synthetic methodology for 1,7-phenanthroline is usually similar to that of quinoline synthesis, for instance Skraup and Friedländer syntheses. General methods for the construction of 1,7-phenanthroline system are classified mainly into three types. One of the routes uses 1,3-phenylenediamine as the starting material and enables to construct two pyridine-rings at the same time.5 The other routes contain a pyridine-ring formation from 5-aminoquinoline1,2a,6 and 7-aminoquinoline.7
In recent years, the development of new methods for the synthesis of many kinds of fluorine-containing heterocycles has been an attractive area of research, since these compounds are now widely recognized as important organic materials showing interesting biological activities for their potential use in medicinal and agricultural scientific fields.8 So far, the report of fluorinated 1,7-phenanthroline derivatives is scarce, and therefore it is of synthetic value to develop convenient and mild approaches for the syntheses of fluorine-containing 1,7-phenanthrolines, which would be expected to exhibit new activities or functionalities.
Very recently, we have reported that N-propargyl-6,8-bis(trifluoroacetyl)quinolin-5-amine (1), prepared by our SNAr methodology,9 undergoes novel pyridine-ring formation reaction with various N-, S- and O-nucleophiles, which attack selectively the terminal acetylenic carbon of 1, to give the corresponding 4-trifluoromethyl-1,7-phenanthrolines (2), which are not easily obtained by other methods, in good yields (Scheme 1).10
In continuation of our work, we wish to report our successive studies on this type of ring formation reaction using the remarkable reactivity of 1 toward various C-nucleophiles, dialkyl malonates, β-diketones, β-ketoesters, methyl cyanoacetate, and malononitrile. This reaction showed an interesting chemoselectivity with changing the electron-withdrawing groups on active methylene compounds and gave an easy and efficient way to introduce additional substitution diversity.
RESULTS AND DISCUSSION
The requisite starting material 1 was readily prepared in two steps by bis(trifluoroacetylation) of N,N-dimethylquinolin-5-amine,11,12 and subsequent dimethylamino-propargylamino exchange reaction of
thus obtained N,N-dimethyl-6,8-bis(trifluoroacetyl)quinolin-5-amine with propargylamine.
The reaction of 1 with dialkyl malonates proceeded smoothly to give the corresponding fluorine-containing 1,7-phenanthrolines as shown in Scheme 2 and the results are summarized in Table 1 (Entries 1-4). In the presence of sodium methoxide (1 equiv), the reaction of 1 with dimethyl malonate (1 equiv) at 30 °C completed within 96 h to afford the corresponding 1,7-phenanthroline (3a) having a di(methoxycarbonyl)ethyl group at the 3-position in 45% yield (Entry 1). Interestingly, the reaction of 1 with bulky di-t-butyl malonate proceeded more easily (at 30 °C for 24 h) to give 3d in 50% yield (Entry 4). In contrast to this, in the cases of diethyl malonate and diisopropyl malonate, the short time (15 min) reaction under reflux conditions gave better results than the long time reaction at 30 °C and the corresponding 1,7-phenanthrolines (3b and 3c) were obtained in 48% and 39% yields, respectively, with less formation of by-products (Entries 2 and 3).
We also tried to conduct the present cyclization using β-diketones (Scheme 2), and the results are shown in Table 1 (Entries 5-7). In the presence of sodium ethoxide (3 equiv), the pyridine-ring formation reaction of 1 with acetylacetone (3 equiv) accompanied by deacetylation took place promptly in refluxing ethanol to afford selectively the novel fluorine-containing 3-acetylethyl-1,7-phenanthroline (3e) in 67% yield (Entry 5). Interestingly, the 1,3-diones which are expected to form initially were not detected in the products. Dibenzoylmethane reacted with 1 quite similarly to give the corresponding benzoylethyl-1,7-phenanthroline (3f) (Entry 6). In the case of the reaction with unsymmetrical benzoylacetone, both deacylated products (3e and 3f) were obtained (Entry 7).
We attempted to apply the present reactions to other active methylene compounds such as β-ketoesters, methyl cyanoacetate, and malononitrile. The cyclization of 1 with methyl acetoacetate proceeded easily in the presence of sodium methoxide to provide the corresponding
1,7-phenanthroline (3g) and deacetylated product (3h) in 22% and 42% yields, respectively, together with small amounts of 3-methoxymethyl-1,7-phenanthroline (2)10 derived from 1 by the reaction with methoxide anion (Scheme 3). In this case, the product (3g) in which acetyl group remained could be obtained in addition to deacylated product (3h) different from the case of the reaction of 1 with β-diketones.
Meanwhile, the reaction of 1 with methyl cyanoacetate and malononitrile gave quite different products. Thus, the reaction of 1 with methyl cyanoacetate in the presence of sodium methoxide occurred at room temperature to give 3-cyano-1-propargyl-1,7-phenanthrolinone (4) in good yield (Scheme 4). With the use of malononitrile as a nucleophile, the reaction of 1 afforded 2-amino-3-cyano-1-propargyl-1,4-dihydro-1,7-phenanthroline (5) as shown in Scheme 4.
Similar to the cases of 1,7-phenanthrolines (2),10 the 6-trifluoroacetyl group of the present products, except for 5, was found to be hydrated easily during the reaction and the work-up process.
Possible mechanistic pathways for the formation of 1,7-phenanthrolines (3) and 1-propargyl-1,7-phenanthrolinone (4) are illustrated in Scheme 5. In path A (the cases of dialkyl malonates, β-diketones, and β-ketoesters), the nucleophilic attack of the enolates at the terminal acetylenic carbon of 1 mediated cyclization similarly to the cases of N-, S-, and O-nucleophiles to give the corresponding exoolefine intermediate, which is converted to 1,7-phenanthrolines (3a-d,g) via 1,3-H shift followed by departure of hydroxide ion. In the cases of the reaction of 1 with β-diketones and β-ketoesters, subsequent deacylation also takes place in situ to afford 3e-f,h. On the other hand, in path B (the cases of methyl cyanoacetate and malononitrile), the addition of enolates occurs exclusively onto the carbonyl carbon of 6-trifluoroacetyl group on 1. In the case of cyanoacetate, the subsequent intramolecular ester-amide exchange reaction (lactam formation) followed by dehydration to give 4. The reaction of 1 with malononitrile affording 5 would also proceed similarly along path B. It is not still clear why the interesting alternation of reaction site occurs with changing the type of active methylene compounds. Further studies are now underway to make clear the faithful mechanism.
In conclusion, we succeeded in extending applicable scope of the pyridine-ring formation reactions of 1 with N-, S-, and O-nucleophiles to those with C-nucleophiles such as a variety of active methylene compounds, dialkyl malonates, β-diketones, β-ketoesters, methyl cyanoacetate, and malononitrile, and presenting a facile and convenient synthetic method accessing CF3-containing 1,7-phenanthrolines and 1,7-phenanthrolinones, which are hardly obtained by other methods. Furthermore, we also found that the crucial chemoselectivity emerged by changing the electron-withdrawing groups on active methylene compounds allowing construction of the variant nitrogen containing heterocyclic systems, pyridine, pyridone, and dihydropyridine.
EXPERIMENTAL
Mps were determined on an electrothermal digital melting point apparatus and are uncorrected. 1H NMR spectra were obtained on a Bruker AVANCE500 spectrometer (1H at 500 MHz, 13C at 126 MHz) and a JEOL PMX 60SI spectrometer (1H at 60 MHz) using TMS as an internal standard. IR spectra were taken with PerkinElmer Spectrum ONE spectrophotometer. Microanalyses were taken with a YANACO CHN-Coder MT-5 analyzer.
Reaction of 1 with Active Methylene Compounds; General Procedure
Sodium (1-3 mmol) and active methylene compounds (1-3 mmol) were added to alcohols (10-40 mL) and the mixture was stirred at room temperature for 15 min. To the solution was added 110 (374 mg, 1 mmol) and then the whole mixture was stirred for 15 min - 96 h at rt - reflux temperature. The reaction was quenched with 1N HCl and the solvent was removed under reduced pressure. The mixture was extracted with EtOAc (100 mL), washed with water (100 mL), and dried over Na2SO4. Evaporation of the solvent gave the crude mixture which was submitted to column chromatography on silica gel eluting with n-C6H14/EtOAc (4:1 - 0:1) to give 2 (Nu = OMe),10 3-5. In the case of malononitrile, the reaction was quenched with sat. aq. NH4Cl instead of 1N HCl.
Dimethyl 2-(6-(2,2,2-trifluoro-1,1-dihydroxyethyl)-4-trifluoromethyl-1,7-phenanthrolin-3-ylmethyl)malonate (3a): mp 137-139 °C (n-C6H14/EtOAc); 1H NMR (60 MHz, CD3CN/CDCl3): δ 9.72 (dd, J = 2.0, 8.0 Hz, 1H, H-10), 9.17 (s, 1H, H-2), 9.03 (dd, J = 2.0, 4.0 Hz, 1H, H-8), 8.88 (q, JHF = 2.0 Hz, 1H, H-5), 8.07-6.88 (br, 2H, OH), 7.82 (dd, J = 4.0, 8.0 Hz, 1H, H-9), 3.95-3.68 (m, 9H, CH2CH, CH3); IR (KBr): 3355, 1755, 1732 cm-1. Anal. Calcd for C21H16F6N2O6: C, 49.81; H, 3.18; N, 5.53. Found: C, 49.57; H, 3.23; N, 5.29.
Diethyl 2-(6-(2,2,2-trifluoro-1,1-dihydroxyethyl)-4-trifluoromethyl-1,7-phenanthrolin-3-ylmethyl)malonate (3b): mp 118-120 °C (n-C6H14/EtOAc); 1H NMR (500 MHz, CDCl3): δ 9.62 (d, J = 8.5 Hz, 1H, H-10), 9.07 (s, 1H, H-2), 8.85 (d, J = 4.0 Hz, 1H, H-8), 8.83 (br s, 1H, H-5), 8.22-7.81 (br, 2H, OH), 7.67 (dd, J = 4.0, 8.5 Hz, 1H, H-9), 4.27-4.14 (m, 4H, CH2CH3), 3.78-3.73 (m, 3H, CH2CH), 1.23 (t, J = 7.0 Hz, 6H, CH2CH3); 13C NMR (126 MHz, CDCl3) δ 168.1, 154.9, 148.8, 146.0, 145.8, 135.0, 133.1 (q, JCF = 30.2 Hz), 131.5, 131.0, 127.8, 126.8, 124.3 (q, JCF = 278.0 Hz), 123.2 (q, JCF = 290.5 Hz), 122.4, 121.1, 95.5 (q, JCF = 33.3 Hz), 62.0, 53.4, 31.2, 14.0; IR (KBr): 3304, 1754, 1725 cm-1. Anal. Calcd for C23H20F6N2O6: C, 51.69; H, 3.77; N, 5.24. Found: C, 51.48; H, 3.79; N, 5.31.
Diisopropyl 2-(6-(2,2,2-trifluoro-1,1-dihydroxyethyl)-4-trifluoromethyl-1,7-phenanthrolin-3-ylmethyl)malonate (3c): mp 176-177 °C (EtOAc); 1H NMR (60 MHz, CDCl3): δ (nonhydrate form) 9.93 (dd, J = 2.0, 9.0 Hz, 1H, H-10), 9.63 (q, JHF = 2.0 Hz, 1H, H-5), 9.32 (s, 1H, H-2), 9.20 (dd, J = 2.0, 4.0 Hz, 1H, H-8), 8.00 (dd, J = 4.0, 9.0 Hz, 1H, H-9), 5.47-4.85 (m, 2H, OCH), 3.78 (br s, 3H, CH2CH), 1.35-1.13 (m, 12H, CH3); IR (KBr): 3451, 1728 cm-1. Anal. Calcd for C25H24F6N2O6: C, 53.38; H, 4.30; N, 4.98. Found: C, 53.75; H, 4.14; N, 4.78.
Di-tert-butyl 2-(6-(2,2,2-trifluoro-1,1-dihydroxyethyl)-4-trifluoromethyl-1,7-phenanthrolin-3-ylmethyl)malonate (3d): mp 133-135 °C (n-C6H14/EtOAc); 1H NMR (500 MHz, CDCl3): δ 9.70 (d, J = 8.5 Hz, 1H, H-10), 9.08 (s, 1H, H-2), 8.95 (d, J = 4.0 Hz, 1H, H-8), 8.87 (br s, 1H, H-5), 7.75 (dd, J = 4.0, 8.5 Hz, 1H, H-9), 7.63 (br s, 2H, OH), 3.62-3.55 (m, 3H, CH2CH), 1.42 (s, 18H, CH3); IR (KBr): 3343, 1749, 1723 cm-1. Anal. Calcd for C27H28F6N2O6: C, 54.92; H, 4.78; N, 4.74. Found: C, 55.12; H, 4.63; N, 4.70.
4-(6-(2,2,2-Trifluoro-1,1-dihydroxyethyl)-4-trifluoromethyl-1,7-phenanthrolin-3-yl)butan-2-one (3e): mp 154-156 °C (n-C6H14/EtOAc); 1H NMR (500 MHz, CDCl3): δ 9.71 (d, J = 8.0 Hz, 1H, H-10), 9.10 (s, 1H, H-2), 8.96 (d, J = 4.0 Hz, 1H, H-8), 8.87 (br s, 1H, H-5), 7.76 (dd, J = 4.0, 8.0 Hz, 1H, H-9), 7.68 (br s, 2H, OH), 3.39 (br s, 2H, CH2), 2.89 (t, J = 7.5 Hz, 2H, CH2CO), 2.19 (s, 3H, CH3); IR (KBr): 3361, 1722 cm-1. Anal. Calcd for C19H14F6N2O3: C, 52.79; H, 3.26; N, 6.48. Found: C, 52.55; H, 3.54; N, 6.31.
1-Phenyl-3-(6-(2,2,2-trifluoro-1,1-dihydroxyethyl)-4-trifluoromethyl-1,7-phenanthrolin-3-yl)propan-1-one (3f): mp 112-113 °C (n-C6H14/EtOAc); 1H NMR (500 MHz, CDCl3): δ 9.63 (d, J = 8.0 Hz, 1H, H-10), 9.16 (s, 1H, H-2), 8.86 (br s, 2H, H-5, H-8), 8.72-8.09 (br, 2H, OH), 7.96 (d, J = 7.5 Hz, 2H, Harom), 7.67 (dd, J = 4.0, 8.0 Hz, 1H, H-9), 7.57 (t, J = 7.5 Hz, 1H, Harom), 7.46 (t, J = 7.5 Hz, 2H, Harom), 3.58 (br s, 2H, CH2), 3.44 (t, J = 7.0 Hz, 2H, CH2CO); IR (KBr): 3410, 3064, 1683 cm-1. Anal. Calcd for C24H16F6N2O3: C, 58.31; H, 3.26; N, 5.67. Found: C, 58.25; H, 3.32; N, 5.64.
Methyl 3-oxo-2-(6-(2,2,2-trifluoro-1,1-dihydroxyethyl)-4-trifluoromethyl-1,7-phenanthrolin-3-ylmethyl)butanoate (3g): mp 107-108 °C (n-C6H14/EtOAc); 1H NMR (500 MHz, CDCl3): δ 9.67 (d, J = 8.3 Hz, 1H, H-10), 9.09 (s, 1H, H-2), 8.92 (d, J = 4.5 Hz, 1H, H-8), 8.84 (s, 1H, H-5), 7.94-7.64 (br s, 2H, OH), 7.74 (dd, J = 4.5, 8.3 Hz, 1H, H-9), 3.90 (t, J = 7.0 Hz, 1H, CH), 3.73, 3.77-3.67, 3.63-3.56 (s, m, 5H, CO2CH3, CH2), 2.30 (s, 3H, COCH3); IR (KBr): 3332, 1736 cm-1. Anal. Calcd for C21H16F6N2O5: C, 51.44; H, 3.29; N, 5.71. Found: C, 51.45; H, 3.46; N, 5.86.
Methyl 3-(6-(2,2,2-trifluoro-1,1-dihydroxyethyl)-4-trifluoromethyl-1,7-phenanthrolin-3-yl)propanoate (3h): mp 114-116 °C (n-C6H14/EtOAc); 1H NMR (500 MHz, CD3CN): δ 9.47 (d, J = 8.3 Hz, 1H, H-10), 9.06 (s, 1H, H-2), 8.92 (d, J = 4.4 Hz, 1H, H-8), 8.71 (br s, 1H, H-5), 8.60 (br s, 2H, OH), 7.71 (dd, J = 4.4, 8.3 Hz, 1H, H-9), 3.67 (s, 3H, CH3), 3.42-3.34 (m, 2H, CH2), 2.76 (t, J = 7.7 Hz, 2H, CH2CO); 13C NMR (126 MHz, CD3CN): δ 173.6, 156.4, 150.6, 147.0, 146.5, 135.9, 135.8, 133.2, 133.0 (q, JCF = 30.9 Hz), 128.6, 128.0, 126.0 (q, JCF = 277.5 Hz), 125.1 (q, JCF = 289.1 Hz), 124.3, 121.9, 96.7 (q, JCF = 32.7 Hz), 52.7, 36.4, 28.6; IR (KBr): 3329, 1733 cm-1. Anal. Calcd for C19H14F6N2O4: C, 50.90; H, 3.15; N, 6.25. Found: C, 51.08; H, 3.19; N, 6.03.
2-Oxo-1-(prop-2-ynyl)-6-trifluoroacetyl-4-trifluoromethyl-1,2-dihydro-1,7-phenanthroline-3-carbonitrile (4): mp 218-219 °C (dec.) (n-C6H14/EtOAc); 1H NMR (500 MHz, CD3CN): δ (hydrate form) 9.45 (d, J = 8.7 Hz, 1H, H-10), 9.07 (d, J = 4.1 Hz, 1H, H-8), 8.47 (br s, 1H, H-5), 8.37-8.18 (br, 2H, OH), 7.83 (dd, J = 4.1, 8.7 Hz, 1H, H-9), 4.93 (br s, 2H, CH2), 3.01 (br s, 1H, C≡CH); 13C NMR (126 MHz, CD3CN): δ (hydrate form) 160.5, 152.0, 148.7, 144.3, 143.4 (q, JCF = 31.3 Hz), 137.3, 129.0, 127.9, 124.6 (q, JCF = 288.9 Hz), 122.5, 122.4 (q, JCF = 277.9 Hz), 120.2, 113.1, 112.9, 108.0, 95.8 (q, JCF = 32.8 Hz), 78.8, 77.1, 44.4; IR (KBr): 3300, 2235, 2134, 1671, 1667 cm-1. Anal. Calcd for C19H7F6N3O2: C, 53.91; H, 1.67; N, 9.93. Found: C, 53.99; H, 1.96; N, 9.57.
2-Amino-4-hydroxy-1-(prop-2-ynyl)-6-trifluoroacetyl-4-trifluoromethyl-1,4-dihydro-1,7-phenanthroline-3-carbonitrile (5): mp 217-218 °C (dec.) (n-C6H14/EtOAc); 1H NMR (500 MHz, CD3CN): δ 8.94 (d, J = 4.1 Hz, 1H, H-8), 8.76 (d, J = 8.6 Hz, 1H, H-10), 8.37 (br s, 1H, H-5), 7.70 (dd, J = 4.1, 8.6 Hz, 1H, H-9), 6.60-6.05 (br, 2H, NH2), 5.74 (br s, 1H, OH), 4.60 (d, Jgem = 18.3 Hz, 1H, CH2), 4.52 (d, Jgem = 18.3 Hz, 1H, CH2), 2.87 (br s, 1H, C≡CH); IR (KBr): 3353, 3313, 2198, 2126, 1635 cm-1. Anal. Calcd for C19H12F6N4O3 (hydrated form): C, 49.80; H, 2.64; N, 12.23. Found: C, 49.70; H, 2.91; N, 12.05.
References
1. S. Prado, S. Michel, F. Tillequin, M. Koch, B. Pfeiffer, A. Pierré, S. Léonce, P. Colson, B. Baldeyrou, A. Lansiaux, and C. Bailly, Bioorg. Med. Chem., 2004, 12, 3943. CrossRef
2. (a) A. D. Yapi, M. Mustofa, A. Valentin, O. Chavignon, J.-C. Teulade, M. Mallie, J.-P. Chapat, and Y. Blache, Chem. Pharm. Bull., 2000, 48, 1886; (b) L. W. Scheibel and A. Adler, Mol. Pharmacol., 1982, 22, 140.
3. (a) J. Cuesta, M. A. Read, and S. Neidle, Mini. Rev. Med. Chem., 2003, 3, 11; (b) J.-L. Mergny, L. Lacroix, M.-P. Teulade-Fichou, C. Hounsou, L. Guittat, M. Hoarau, P. B. Arimondo, J.-P. Vigneron, J.-M. Lehn, J.-F. Riou, T. Garestier, and C. Hélène, Proc. Natl. Acad. Sci. U.S.A., 2001, 98, 3062. CrossRef
4. V. R. Pedireddi, Cryst. Growth Des., 2001, 1, 383. CrossRef
5. (a) Q. Zhang, Y. Chen, Y. Q. Zheng, P. Xia, Y. Xia, Z. Y. Yang, K. F. Bastow, S. L. Morris-Natschke, and K.-H. Lee, Bioorg. Med. Chem., 2003, 11, 1031; CrossRef (b) P. K. Mahata, C. Venkatesh, U. K. S. Kumar, H. Ila, and H. Junjappa, J. Org. Chem., 2003, 68, 3966; CrossRef (c) G. I. Graf, D. Hastreiter, L. Everson da Silva, R. A. Rebelo, A. G. Montalban, and A. McKillop, Tetrahedron, 2002, 58, 9095; CrossRef (d) J. Bethke and P. Margaretha, Helv. Chim. Acta, 2002, 85, 544; CrossRef (e) F. F. Molock and D. W. Boykin, J. Heterocycl. Chem., 1983, 20, 681. CrossRef
6. (a) V. V. Kouznetsov, L. Y. V. Mendez, B. Tibaduiza, C. Ochoa, D. M. Pereira, J. J. N. Ruiz, C. F. Portillo, S. M. Serrano, A. G. Barrio, A. Bahsas, and J. Amaro-Luis, Arch. Pharm. (Weinheim), 2004, 337, 127; CrossRef (b) U. Jordis, F. Sauter, M. Rudolf, and G. Cai, Monatsh. Chem., 1988, 119, 761. CrossRef
7. (a) A. Tatibouet, R. Hancock, M. Demeunynck, and J. Lhomme, Angew. Chem., Int. Ed. Engl., 1997, 36, 1190; CrossRef (b) C. F. Nutaitis and K. Smith, Org. Prep. Proced. Int., 2007, 39, 611; CrossRef (c) M. Jastrzebska-Glapa and J. Mlochowski, Rocz. Chem., 1976, 50, 987.
8. (a) A. S. Dey and M. M. Joullié, J. Heterocycl. Chem., 1965, 2, 120; CrossRef (b) E. B. Nyquist and M. M. Joullié, J. Heterocycl. Chem., 1967, 4, 539; CrossRef (c) M. Loy and M. M. Joullié, J. Med. Chem., 1973, 16, 549; CrossRef (d) R. Filler, 'Organofluorine Chemicals and Their Industrial Applications,' Ellis Horwood, London, 1979; (e) R. Filler and Y. Kobayashi, 'Biomedicinal Aspects of Fluorine Chemistry,' Kodansha & Elsevier Biomedical, Tokyo, 1982; (f) J. T. Welch, Tetrahedron, 1987, 43, 3123; CrossRef (g) R. Filler, Y. Kobayashi, and L. M. Yagupolskii, 'Organofluorine Compounds in Medicinal Chemistry and Biomedical Applications,' Elsevier, Amsterdam, 1993; (h) K. Burger, U. Wucherpfennig, and E. Brunner, Adv. Heterocycl. Chem., 1994, 60, 1. CrossRef
9. (a) M. Hojo, R. Masuda, and E. Okada, Tetrahedron Lett., 1987, 28, 6199; CrossRef (b) M. Hojo, R. Masuda, E. Okada, and H. Miya, Synthesis, 1989, 870; CrossRef (c) E. Okada and N. Tsukushi, Synlett, 1999, 210; CrossRef (d) E. Okada, N. Tsukushi, and N. Shimomura, Synthesis, 2000, 237. CrossRef
10. D. Shibata, E. Okada, M. Hinoshita, and M. Médebielle, Synthesis, 2009, 3039. CrossRef
11. J. O. Mellor and G. D. Merriman, Steroids, 1995, 60, 693. CrossRef
12. C. Feller and J. Renault, Bull. Soc. Chim. France, 1973, 3, 1112.