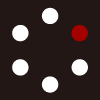
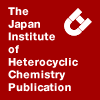
HETEROCYCLES
An International Journal for Reviews and Communications in Heterocyclic ChemistryWeb Edition ISSN: 1881-0942
Published online by The Japan Institute of Heterocyclic Chemistry
e-Journal
Full Text HTML
Received, 18th May, 2010, Accepted, 17th June, 2010, Published online, 18th June, 2010.
DOI: 10.3987/COM-10-S(E)35
■ Reductive Cyclization of 3-Cyanomethyloxindoles to Hexahydro-2-oxopyrrolo[2,3-b]indoles with Lithium Aluminum Hydride
Masashi Shinada, Yoshiyuki Aihara, Satoshi Takiguchi, Naoko Ban, Kazuhiro Higuchi, and Tomomi Kawasaki*
Meiji Pharmaceutical University, 2-522-1 Noshio, Kiyose, Tokyo 204-8588, Japan
Abstract
Reduction of 3-cyanomethyloxindoles with lithium aluminum hydride at low temperature proceeded smoothly with cyclization to afford hexahydro-2-oxopyrrolo[2,3-b]indoles.INTRODUCTION
The hexahydropyrrolo[2,3-b]indole alkaloids constitute a large family of The hexahydropyrrolo[2,3-b]indole alkaloids constitute a large family of natural products.1 The reductive cyclization of oxindoles possessing a C2N unit such as 2-aminoethyl,2,3 2-azidoethyl,4 2-hydrazonoethyl,5 carbamoylmethyl,6-7 and cyanomethyl groups8-10 at the C3 position are useful for constructing the pyrroloindole framework (Scheme 1). Among them, the reduction of 3-cyanomethyloxindoles 1 with LiAlH4 under heating conditions is a convenient and efficient route to hexahydropyrroloindoles 2.10 This reaction could conceivably proceed through any of the following route: a) an initial reduction of the cyano group to an amino group followed by a second reduction of the lactam moiety to aminal, and cyclization to pyrroloindole, or b) the contrary reductive way (Scheme 2). In our synthetic studies of pyrroloindole alkaloids,11 we have also utilized this methodology. To date, we have found that the LiAlH4 reduction of 3-cyanomethyloxindoles 1 at low temperature proceeds smoothly to provide hexahydro-2-oxopyrroloindoles 3 (Scheme 3) and that,
contrary to the above considerations, the reductive cyclization of 1 to 2 includes the reduction process of 3. In this paper, we describe a new LiAlH4-reductive cyclization of oxindoles 1 to 2-oxopyrroloindoles 3.
RESULTS AND DISCUSSION
3-Cyanomethyloxindoles 1a, 1b, and 1f were obtained by N-alkylation of 1e and 1g, which were prepared according to our synthetic methods, respectively (Scheme 4).11d Oxindole 1c was prepared from commercially available 3-cyanomethylindole by allylation, oxidation, and methylation,
and 1d was obtained according to the reported method.9
Next we attempted the reduction of compounds 1a-g with LiAlH4 in THF under several conditions (Scheme 5, Table 1). We initially performed the ordinary reduction of 1a with LiAlH4 in THF under reflux conditions for 10 min to obtain the corresponding pyrroloindole 2a in 60% yield (entry 1). When LiAlH4-reduction of 1a was carried out at room temperature, 2-oxopyrroloindole 3a was formed in 11% yield together with 2a (68%) (entry 2). On treatment at the lower temperature (–15 °C) for 10 min, the starting compound was remained, but the formation of 3a was increased (entry 3). Furthermore, a prolonged reaction (1.5 h) under the same temperature provided 3a (64%) in preference to 2a (5%) (entry 4). Reduction of 3a with LiAlH4 under reflux conditions gave 2a (89%).12 These results indicate that 3a is an intermediate in the LiAlH4-reductive cyclization of 1a to 2a.
To the best of our knowledge, this is the first example of the formation of 2-oxopyrroloindole 3 in LiAlH4-reduction, thus we attempted the reduction of 1b-d at –15 °C for 5 min to give the same results in the formation of 3b-d in good yields, respectively (entries 5-7). In the case of R1 = H, the desired reduction of 1e at –15 °C did not occurred at all, but the high-temperature reaction afforded 2e in 54% yield (entries 8, 9). The reduction of 1f and 1g both possessing a bulky substituent at the C3 site required prolonged heating to give the corresponding 2f and 2g13 (entries 10, 11). The low-temperature reductive cyclization of 1 to 3 is affected by the bulkiness of the C3-substituent and the presence of NH of the lactam moiety.
The formation of 3 is explained by the initial reduction of the lactam moiety of 1 to the aminal intermediate A. Once the aminal A cyclizes to form the furan ring B, the ring opening generates the iminium species C followed by cyclization of the amide moiety to 3 (Scheme 6).
In summary, we developed a method for the preparation of 2-oxopyrroloindole 3 by LiAlH4-reductive cyclization of oxindoles 1 at low temperature, and demonstrated that 3 is an intermediate in the high-temperature reduction to pyrroloindole 2.
EXPERIMENTAL
General. 1H-NMR spectra were obtained using a JEOL JNM-EX-300, or JNM-EX-400 spectrometer with tetramethylsilane as an internal standard. J-Values are given in Hz. Mass spectra were obtained using a JEOL JMS-DX302 or JMS-700 instrument with a direct inlet system operating at 70 eV. IR spectra were recorded with a Shimadzu FTIR-8100 spectrophotometer. All mp values are uncorrected, and were measured on a Yanagimoto micromelting point apparatus. Elemental analyses were obtained using a Yanaco CHN Corder MT-6 elemental analyzer. Column chromatography was carried out on silica gel (Kanto Chemical Co. Inc., Silica Gel 60N, 100-200 mesh and Merck, Silica Gel 60, 230-400 mesh).
General Alkylation Procedure
To a suspension of NaH (60%) (16.5 mmol) in dry DMF (30 mL) was added a solution of the corresponding oxindole 1e or 1g (15 mmol) in dry DMF (20 mL) at 0 °C, and then the stirring mixture was warmed up to room temperature to keep at the same temperature for 1 h. After cooling to 0 °C, alkyl halide (16.5 mmol) was added to the resulted mixture. After 15 min., the reaction mixture was quenched with water (300 mL), and the mixture was extracted with diethyl ether. The extract was washed with brain, dried over MgSO4, and concentrated. The residue was purified by silica gel column chromatography with EtOAc-hexane (1 : 1 – 5) to afford the alkylated product 1a (91%),14 1b (88%)11d or 1f (quant. yield), respectively.
1-Allyl-3-cyanomethyl-3-t-pentenyloxindole (1f)
Prepared from 1g as colorless crystals; mp 99-100 ℃ (AcOEt); IR (CHCl3) ν 2252, 1707, 1612 cm-1; 1H NMR (400 MHz, CDCl3) δ 1.05 (3H, s), 1.15 (3H, s), 2.86 (1H, d, J = 16.4 Hz), 3.01 (1H, d, J = 16.4 Hz), 4.29 (1H, tdd, J = 16.4, 5.4, 1.2 Hz), 4.47 (1H, tdd, J = 16.4, 5.4, 1.2 Hz), 5.09 (1H, d, J = 17.6 Hz), 5.20 (1H, d, J = 10.7 Hz), 5.23 (1H, qd, J = 10.3, 1.2 Hz), 5.29 (1H, qd, J = 17.1, 1.2 Hz), 5.82 (1H, tdd, J = 17.1, 10.3, 5.4 Hz), 6.06 (1H, dd, J = 17.6, 10.7 Hz), 6.88 (1H, d, J = 7.8 Hz), 7.09 (1H, td, J = 7.8, 1.0 Hz), 7.32 (1H, d, J = 7.8 Hz), 7.34 (1H, td, J = 7.8, 1.0 Hz); 13C NMR (100 MHz, CDCl3) δ 21.8, 22.1, 22.1, 41.8, 42.6, 54.8, 109.2, 115.0, 116.6, 117.8, 122.1, 125.3, 127.5, 129.0, 130.8, 141.9, 143.4, 175.7; MS (EI) m/z (%) 280 (M+, 6), 212 (100), 185 (45), 69 (33), 41 (15). Anal. Calcd for C18H20N2O: C, 77.11; H, 7.19; N, 9.99. Found: C, 77.04; H, 7.45; N, 10.02.
Preparation of 1-Allyl-3-cyanomethyl-3-methyloxindole (1c)
A mixture of 3-cyanomethylindole (3.0 g, 19.2 mmol), allyl bromide (2.49 mL, d = 1.398, 28.8 mmol), 10% NaOH (20 mL), and tetrabutylammoniun hydrosulfate (1.3 g, 3.84 mmol) in CH2Cl2 (60 mL) was stirred at room temperature. After consumption of the starting indole, the resulted mixture was extracted with CH2Cl2, and the extract was washed with water and brine, dried over MgSO4, and concentrated under reduced pressure. The obtained residue was chromatographed by silica gel column with EtOAc-hexane (1 : 4) to give 1-allyl-3-cyanomethylindole (3.43 g, 91%) as a pale yellow oil; IR (CHCl3) ν 2251, 1645, 1616 cm-1; 1H NMR (300 MHz, CDCl3) δ 3.81 (2H, s), 4.68 (2H, d, J = 5.3 Hz), 5.09 (1H, dd, J = 17.0, 1.1 Hz), 5.21 (1H, dd, J = 10.2, 1.1 Hz), 5.96 (1H, ddt, J = 17.0, 10.2, 5.3 Hz), 7.07 (1H, s), 7.16 (1H, t, J = 7.8 Hz), 7.25 (1H, t, J = 7.8 Hz), 7.31 (1H, d, J = 7.8 Hz), 7.56 (1H, d, J = 7.8 Hz); 13C NMR (75 MHz, CDCl3) δ 14.3, 48.8, 103.4, 110.0, 117.7, 118.18, 118.22, 119.8, 122.4, 126.3, 126.6, 133.0, 136.5. HRMS (EI) m/z 196.1004 (M+, C13H12N2 requires 196.1000).
To a solution of 1-allyl-3-cyanomethylindole (3.0 g, 15.3 mmol) in DMSO (5.25 mL) was gradually added concentrated HCl (35 mL) at 0 °C. After stirring at room temperature for 4 h, the reaction mixture was diluted with water (10 mL), neutralized with K2CO3 (powder), and extracted with AcOEt. The extract was washed with water and brine, dried over MgSO4, and concentrated under reduced pressure. The obtained residue was chromatographed by silica gel column with EtOAc-hexane (1 : 1) to give 1-allyl-3-cyanomethyloxindole (3.24 g, quant. yield) as a pale yellow oil; IR (CHCl3) ν 2253, 1714, 1647, 1614 cm-1; 1H NMR (300 MHz, CDCl3) δ 2.74 (1H, dd, J = 16.9, 8.8 Hz), 3.13 (1H, dd, J = 16.9, 4.7 Hz), 3.71 (1H, dd, J = 8.8, 4.7 Hz), 4.36 (2H, d, J = 5.3 Hz), 5.23 (1H, d, J = 10.5 Hz), 5.25 (1H, d, J = 15.5 Hz), 5.85 (1H, ddt, J = 15.5, 10.5, 5.3 Hz), 6.88 (1H, d, J = 7.8 Hz), 7.13 (1H, t, J = 7.8 Hz), 7.33 (1H, t, J = 7.8 Hz), 7.51 (1H, d, J = 7.8 Hz); 13C NMR (75 MHz, CDCl3) δ 19.0, 41.3, 42.5, 109.5, 117.0, 117.9, 123.0, 124.2, 125.5, 129.2, 130.7, 143.3, 173.9. HRMS (EI) m/z 212.0948 (M+, C13H12N2O2 requires 212.0950).
A mixture of 1-allyl-3-cyanomethyloxindole (3.0 g, 14.1 mmol), methyl iodide (1.30 mL, d = 2.317, 21.2 mmol), 10% NaOH (20 mL), and tetrabutylammoniun hydrosulfate (0.96 g, 2.83 mmol) in CH2Cl2 (60 mL) was stirred at room temperature. After consumption of the starting oxindole, the resulted mixture was extracted with CH2Cl2. The extract was washed with water and brine, dried over MgSO4, and concentrated under reduced pressure. The obtained residue was chromatographed by silica gel column with EtOAc-hexane (2 : 3) to give 1c (3.20 g, 87%) as a pale yellow oil; IR (CHCl3) ν 2251, 1715, 1647, 1614 cm-1; 1H NMR (300 MHz, CDCl3) δ 1.54 (3H, s), 2.60 (1H, d, J = 16.5 Hz), 2.86 (1H, d, J = 16.5 Hz), 4.32 (1H, ddt, J = 14.7, 5.2, 1.8 Hz), 4.39 (1H, ddt, J = 14.7, 5.2, 1.8 Hz), 5.22 (1H, dt, J = 17.4, 1.8 Hz), 5.23 (1H, dt, J = 10.8, 1.8 Hz), 5.86 (1H, ddt, J = 17.4, 10.8, 5.2 Hz), 6.90 (1H, d, J = 7.5 Hz), 7.13 (1H, t, J = 7.5 Hz), 7.34 (1H, t, J = 7.5 Hz), 7.47 (1H. d, J = 7.5 Hz); 13C NMR (75 MHz, CDCl3) δ 22.3, 26.3, 42.4, 44.8, 109.5, 116.5, 117.7, 123.06, 123.13, 129.0, 130.8, 130.9, 141.8, 177.2. MS (EI) m/z (%) 226 (M+, 64), 186 (100). 158 (10), 143 (8), 130 (14). HRMS (EI) m/z 226.1111 (M+, C14H14N2O requires 226.1106).
General Reductive Cyclization Procedure
To a solution of 3-cyanomethyloxindole 1 (1.86 mmol) in dry THF (1.6 mL) was added LiAlH4 (powder 95%, 18.6 mmol). The mixture was stirred at the corresponding reaction temperature for the desired period (Table 1), quenched with THF-H2O (1 : 1), and filtrated through Cerite. The filtrate was washed with sat. aq. Na2CO3, and the aqueous layer was extracted with EtOAc. The extract was washed with brine, dried over MgSO4, and concentrated under reduced pressure. The obtained residue was chromatographed by silica gel column with EtOAc-hexane (1 : 1) to give the corresponding 2 and/or 3. The products 2e, 2g, and 3d were confirmed by direct comparison to these spectral data with that of the authentic samples.11d,14b
3a,8-Diallyl-1,2,3,3a,8,8a-hexahydropyrrolo[2,3-b]indole (2a)
A colorless oil; IR (CHCl3) ν 1639, 1605 cm-1; 1H NMR (300 MHz, CDCl3) δ 1.91 (1H, ddd, J = 11.1, 10.8, 6.8 Hz), 1.99 (1H, ddd, J = 11.1, 5.7, 1.8 Hz), 2.22 (1H, br), 2.43 (1H, dd, J = 13.9, 8.2 Hz), 2.60 (1H, ddt, J = 13.9, 8.2, 1.3 Hz), 2.73 (1H, td, J = 10.8, 5.7 Hz), 3.00 (1H, ddd, J = 10.8, 6.8, 1.8 Hz), 3.78-3.91 (2H, m), 4.74 (1H, s), 5.00-5.09 (2H, m), 5.13 (1H, dq, J = 10.2, 1.6 Hz), 5.22 (1H, dq, J = 17.0, 1.6 Hz), 5.68 (1H, dddd, J = 16.5, 10.1, 8.1, 6.2 Hz), 5.85 (1H, ddt, J = 17.0, 10.2, 5.3 Hz), 6.30 (1H, d, J = 7.5 Hz), 6.61 (1H, t, J = 7.5 Hz), 6.99-7.05 (2H, m); 13C NMR (75 MHz, CDCl3) δ 41.3, 43.8, 45.4, 47.8, 56.0, 87.2, 105.1, 116.2, 116.6, 117.6, 123.2, 127.7, 133.6, 134.4, 134.9, 150.7. MS (EI) m/z (%) 240 (M+, 100), 199 (76), 182 (38), 170 (31), 158 (35), 130 (14). HRMS (EI) m/z 240.1629 (M+, C16H20N2 requires 240.1626).
3a,8-Diallyl-3,3a,8,8a-tetrahydropyrrolo[2,3-b]indol-2(1H)-one (3a)
White powder; mp 121-124 °C (AcOEt/hexane); IR (CHCl3) ν 3426, 3204, 1694, 1641, 1607 cm-1; 1H NMR (300 MHz, CDCl3) δ 2.47 (1H, dd, J = 14.1, 7.8 Hz), 2.54 (1H, dd, J = 14.1, 7.8 Hz), 2.65 (2H, s), 3.78-3.90 (2H, m), 5.00 (1H, s), 5.02-5.11 (2H, m), 5.23 (1H, dq, J = 10.2, 1.5 Hz), 5.34 (1H, dq, J = 17.4, 1.5 Hz), 5.66 (1H, m), 5.95 (1H, ddt, J = 17.4, 10.2, 5.7 Hz), 6.06 (1H, br), 6.47 (1H, d, J = 7.5 Hz), 6.75 (1H, t, J = 7.5 Hz), 7.06 (1H, d, J = 7.5 Hz), 7.13 (1H, t, J = 7.5 Hz); 13C NMR (75 MHz, CDCl3) δ 42.0, 42.8, 48.5, 51.4, 81.3, 107.3, 117.8, 118.4, 119.1, 123.4, 128.7, 132.9, 133.5, 133.7, 148.6, 176.9. MS (EI) m/z (%) 254 (M+, 99), 213 (100), 170 (29). Anal. Calcd for C16H18N2O: C, 75.56; H, 7.13; N, 11.01. Found: C, 75.57; H, 7.30; N, 10.97.
3a-Allyl-8-methyl-3,3a,8,8a-tetrahydropyrrolo[2,3-b]indol-2(1H)-one (3b)
Colorless crystals; mp 124-126 °C (AcOEt/hexane). IR (CHCl3) ν 3428, 3202, 1693, 1639, 1607 cm-1; 1H NMR (300 MHz, CDCl3) δ 2.49 (1H, dd, J = 14.1, 8.1 Hz), 2.53 (1H, dd, J = 14.1, 7.5 Hz), 2.65 (2H, s), 2.85 (3H, s), 4.95 (1H, s), 5.08-5.16 (2H, m), 5.69 (1H, dddd, J = 17.0, 10.0, 8.1, 7.5 Hz), 6.42 (1H, d, J = 7.5 Hz), 6.73 (1H, t, J = 7.5 Hz), 7.04 (1H, d, J = 7.5 Hz), 7.17 (1H, t, J = 7.5 Hz), 7.18 (1H, br); 13C NMR (75 MHz, CDCl3) δ 31.8, 42.0, 42.4, 51.6, 82.9, 107.1, 118.4, 119.2, 123.3, 128.9, 133.0, 133.5, 149.4, 176.9. MS (EI) m/z 228 (M+, 100), 187 (95), 160 (20), 144 (36). HRMS (EI) m/z 228.1260 (M+, C14H16N2O requires 228.1263). Anal. Calcd for C14H16N2O: C, 73.66; H, 7.06; N, 12.27. Found: C, 73.50; H, 7.06; N, 12.18.
8-Allyl-3a-methyl-3,3a,8,8a-tetrahydropyrrolo[2,3-b]indol-2(1H)-one (3c)
White powder; mp 147-148 °C (AcOEt/hexane); IR (CHCl3) ν 3428, 1695, 1643, 1607 cm-1; 1H NMR (300 MHz, CDCl3) δ 1.46 (3H, s), 2.52 (1H, d, J = 17.0 Hz), 2.73 (1H, d, J = 17.0 Hz), 3.81 (1H, ddt, J = 15.8, 6.2, 1.2 Hz), 3.89 (1H, ddt, J = 15.8, 6.2, 1.2 Hz), 4.90 (1H, s), 5.21 (1H, dq, J = 10.1, 1.2 Hz), 5.33 (1H, dq, J = 17.0, 1.2 Hz), 5.92 (1H, ddt, J = 17.0, 10.1, 6.2 Hz), 6.45 (1H, d, J = 7.5 Hz), 6.74 (1H, t, J = 7.5 Hz), 7.05 (1H, d, J = 7.5 Hz), 7.10 (1H, t, J = 7.5 Hz), 7.15 (1H, br); 13C NMR (75 MHz, CDCl3) δ 25.6, 44.0, 48.1, 48.7, 84.0, 107.3, 117.9, 118.6, 122.7, 128.6, 133.8, 135.1, 148.1, 177.0. MS (EI) m/z 228 (M+, 100), 201 (14), 184 (39), 170 (21), 144 (10). Anal. Calcd for C14H16N2O: C, 73.66; H, 7.06; N, 12.27. Found: C, 73.85; H, 7.23; N, 12.31.
8-Allyl-1,2,3,3a,8,8a-hexahydro-3a-(2-methylbut-3-en-2-yl)pyrrolo[2,3-b]indole (2f)
A colorless oil; IR (CHCl3) ν 1635, 1601 cm-1; 1H NMR (400 MHz, CDCl3) δ 0.98 (3H, s), 1.11 (3H, s), 1.87 (1H, ddd, J = 11.7, 5.1, 0.7 Hz,), 1.97 (1H, br), 2.08 (1H, ddd, J = 11.7, 11.4, 7.0 Hz), 2.55 (1H, ddd, J = 11.4, 11.0, 5.1 Hz), 2.97 (1H, ddd, J = 11.0, 7.0, 0.7 Hz), 3.82 (1H, tdd, J = 16.5, 4.9, 1.7 Hz), 3.90 (1H, tdd, J = 16.5, 5.6, 1.4 Hz), 4.77 (1H, s), 5.02 (1H, dd, J = 17.4, 1.2 Hz), 5.08 (1H, dd, J = 10.7, 1.2 Hz), 5.14 (1H, qd, J = 10.3, 1.4 Hz), 5.25 (1H, qd, J = 17.1, 1.7 Hz), 5,87 (1H, dddd, J = 17.1, 10.3, 5.6, 4.8 Hz), 6.01 (1H, dd, J = 17.4, 10.7 Hz), 6.28 (1H, d, J = 7.5 Hz), 6.57 (1H, td, J = 7.5, 1.0 Hz), 7.04 (1H, td, J = 7.5, 1.0 Hz), 7.11 (1H, 1d, J = 7.5, 1.0 Hz); 13C NMR (100 MHz, CDCl3) δ 23.1, 23.6, 37.6, 41.3, 46.2, 47.6, 63.5, 84.9, 104.5, 113.0, 115.9, 116.0, 124.8, 127.7, 131.8, 134.5, 144.9, 151.3. MS (EI) m/z 268 (M+, 22), 199 (100), 158 (26). HRMS (EI) m/z 268.1941 (M+, C18H24N2 requires 268.1939).
Reduction of 3a with LiAlH4 to 2a
A suspension of 2-oxopyrroloindole 3a (10.4 mg, 0.041 mmol) and LiAlH4 (powder 92%, 16.9 mg, 0.41 mmol) in dry THF (410 µmL) was heated under reflux for 1.5 h. The reaction mixture was allowed to treat in the same manner as the reductive protocol described above to give pyrroloindole 2a (8.7 mg, 89%).
ACKNOWLEDGEMENTS
This work was supported by a Grant-in-Aid for Scientific Research (C) (No. 21590028) and for the High-Tech Research Center Project (S0801043) from the Ministry of Education, Culture, Sports, Science and Technology (MEXT), Japan. We are grateful to N. Eguchi, T. Koseki, and S. Kubota at the Analytical Center of MPU for performing microanalysis and mass spectrometry.
References
1. Reviews: (a) D. Crich and A. Banerjee, Acc. Chem. Res., 2007, 40, 151; CrossRef (b) U. Anthoni, C. Christophersen, and P. H. Nielsen, Alkaloids: Chemical and Biological Perspectives, ed. by S. W. Pelletiesr, Pergamon Press: New York, 1999, Vol. 13, p. 163; (c) S. Takano and K. Ogasawara, The Alkaloids, ed. by A. Brossi, Academic, San Diego, CA, 1989, Vol. 36, p. 225.
2. Selected recent examples using LiAlH4: (a) Y. Yasui, H. Kamisaki, and Y. Takemoto, Org. Lett., 2008, 10, 3303; CrossRef (b) L. E. Overman and Y. Shin, Org. Lett., 2007, 9, 339. CrossRef
3. Selected recent examples using Na/HOEt: (a) J. J. Kodanko, S. Hiebert, E. A. Peterson, L. Sung, L. E. Overman, V. de M. Linck, G. C. Goerck, Y. A. Amador, M. B. Leal, and E. Elisabetsky, J. Org. Chem., 2007, 72, 7909; CrossRef (b) L. E. Overman and E. A. Peterson, Tetrahedron, 2003, 59, 6905. CrossRef
4. Using Red-Al: (a) C. Menozzi, P. I. Dalko, and J. Cossy, Chem. Commun., 2006, 4638; CrossRef (b) L. E. Overman and D. V. Paone, J. Am. Chem. Soc., 2001, 123, 9465. CrossRef
5. Using LiAlH4: M. K. G. Mekhael and H. Heimgartner, Helv. Chim. Acta, 2003, 86, 2805. CrossRef
6. Selected recent examples using LiAlH4: (a) Y. Kobayashi, H. Kamisaki, H. Takeda, Y. Yasui, R. Yanada, and Y. Takemoto, Tetrahedron, 2007, 63, 2978; CrossRef (b) M. Pallavicini, E. Valoti, L. Villa, and I. Resta, Tetrahedron Asymmetry, 2005, 16, 2493. CrossRef
7. Using BH3·THF: C.-L. Fang, S. Horne, N. Taylor, and R. Rodrigo, J. Am. Chem. Soc., 1994, 116, 9480. CrossRef
8. Using Red-Al: X.-F. Pei and S. Bi, Heterocycles, 1994, 39, 357. CrossRef
9. Using Na/HOEt: P. L. Julian, J. Pikl, and D. Boggess, J. Am. Chem. Soc., 1934, 56, 1797. CrossRef
10. Selected recent examples using LiAlH4: (a) Y. Nakao, S. Ebata, A. Yada, T. Hiyama, M. Ikawa, and S. Ogoshi, J. Am. Chem. Soc, 2008, 130, 12874; CrossRef (b) E. Rivera-Becerri, P. Josep-Nathan, V. M. Pérez-Álvarez, and M. S. Morales-Ríos, J. Med. Chem, 2008, 51, 5271; CrossRef (c) A. Pinto, Y. Jia, L. Neuville, and J. Zhu, Chem. Eur. J., 2007, 13, 961; CrossRef (d) M. S. Morales-Ríos, N. F. Santos-Sánchez, Y. Mora-Pérez, and P. Josep-Nathan, Heterocycles, 2004, 63, 1131. CrossRef
11. (a) S. Takiguchi, T. Iiduka, Y. Kumakura, K. Murasaki, N. Ban, K. Higuchi, and T. Kawasaki, J. Org. Chem., 2010, 75, 1126; CrossRef (b) T. Kawasaki, M. Shinada, M. Ohzono, A. Ogawa, R. Terashima, and M. Sakamoto, J. Org. Chem., 2008, 73, 5959; CrossRef (c) T. Kawasaki, M. Shinada, D. Kamimura, M. Ohzono, and A. Ogawa, Chem. Commun. 2006, 420; CrossRef (d) T. Kawasaki, A. Ogawa, R. Terashima, T. Saheki, N. Ban, H. Sekiguchi, K. Sakaguchi, and M. Sakamoto, J. Org. Chem., 2005, 70, 2957; CrossRef (e) T. Kawasaki, A. Ogawa, Y. Takashima, and M. Sakamoto, Tetrahedron Lett., 2003, 44, 1591. CrossRef
12. (a) P. Rosenmund and E. Sadri, Liebigs Ann. Chem., 1979, 927; CrossRef (b) P. Rosenmund and A. Sotiriou, Chem. Ber., 1975, 108, 208. CrossRef
13. Our recent report described the LiAlH4-reduction of 1g in refluxing 1,4-dioxane for 3 h to give a higher yield of 2g, see ref. 11a.
14. B. M. Trost and Y. Zang, J. Am. Chem. Soc., 2006, 128, 4590. CrossRef