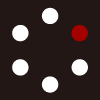
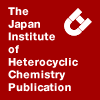
HETEROCYCLES
An International Journal for Reviews and Communications in Heterocyclic ChemistryWeb Edition ISSN: 1881-0942
Published online by The Japan Institute of Heterocyclic Chemistry
e-Journal
Full Text HTML
Received, 29th May, 2010, Accepted, 1st July, 2010, Published online, 1st July, 2010.
DOI: 10.3987/COM-10-S(E)40
■ Studies on the Synthesis and Metabolism of 14-epi-2α-(3-Hydroxypropyl)-19-norvitamin D3 and Its 2β-Isomer
Atsushi Kittaka,* Hideki Hara, Kaori Yasuda, Masashi Takano, Midori A. Arai, Daisuke Sawada, Hiroshi Saito, Kazuya Takenouchi, Tai C. Chen, and Toshiyuki Sakaki*
Department of Pharmaceutical Chemistry, Faculty of Pharmaceutical Sciences, Teikyo University, 1091-1 Suarashi, Midori-ku, Sagamihara-shi, Kanagawa 252-5195, Japan
Abstract
Two derivatives of 14-epi-1α,25-Dihydroxy-19-norvitamin D3, 14-epi-2α-(3-hydroxypropyl)-1α,25-dihydroxy-19-norvitamin D3 (14-epi-MART-10) and its 2-epimeric analog (14-epi-MART-11), were synthesized using Julia coupling reaction to connect between the C5 position (steroidal numbering) of an A-ring precursor ketone derived from (–)-quinic acid and the C6 position of a protected 14-epi-CD-ring benzothiazole sulfone. The coupling and deprotection reactions generated a mixture of 14-epi-MART-10 and 14-epi-MART-11 in a moderate yield. The C2-isomers were then separated as their pivalate forms. The C2-stereochemistry of 2α- and 2β-isomers was determined by 1H NMR studies including NOE experiments. The pivaloyl group was removed under basic conditions to obtain the target molecules of 14-epi-MART-10 and 14-epi-MART-11. The metabolism of these two new analogs was further studied in a reconstituted cell-free human CYP24A1 system to elucidate the potential mechanism of their super agonistic action on vitamin D receptor. Our results indicate that epimerization at C14 makes the analogs less susceptible to CYP24A1 degradation and therefore more bio-available, leading to enhanced biological activities.INTRODUCTION
The biological active form of vitamin D3, 1α,25-dihydroxyvitamin D3 (1α,25(OH)2D3, 1), regulates calcium and phosphorus homeostasis and promotes cell differentiation and apoptosis, and also suppresses autoimmune diseases.1 Most of the biological actions of 1α,25(OH)2D3 are mediated through binding to vitamin D receptor (VDR), which belongs to the nuclear receptor superfamily.2,3 The potent calcemic activity of 1α,25(OH)2D3 prevents its application in pharmacological doses for cancer chemotherapy, even though 1α,25(OH)2D3 is able to inhibit cancer cell growth.4,5 We synthesized a series of active vitamin D3 analogs with C2-modifications and found some of them showing greater VDR agonistic activity than 1α,25(OH)2D3.6-10 For example, 2α-(3-hydroxypropyl)-1α,25(OH)2D3 (2, Figure 1) showed three-fold higher binding activity for VDR, and the mechanism of the effects of the 2α-(3-hydroxypropyl) group on enhanced VDR binding has been explained by X-ray crystallographic analysis of the VDR-2 complex, i.e., the terminal hydroxy group of 2 forms a hydrogen bond with Arg274 and replaces one of the water molecules in the ligand binding domain (LBD) of the VDR to stabilize the complex.11 On the other hand, we synthesized several highly potent VDR-antagonists (the highest IC50 = 7.4 pM) with C2α-functionalization as well as 24-alkyl modification on the lactone ring of Teijin analog, TEI-9647.12-14 1α,25-Dihydroxy-19-norvitamin D3 (3) is known to have a selective activity profile, combining high potency in inducing differentiation of malignant cells with very low or no calcemic activity.15-18 The C2-modified active 19-norvitamin D3 analog, MART-10 (2α-(3-hydroxypropyl)-1α,25-dihydroxy-19-norvitamin D3), is 36 times more potent than the natural hormone 1α,25(OH)2D3 in inducing HL-60 human promyelocytic leukemia cell differentiation.19,20 MART-10 is also about 500- to 1000-fold more active than 1α,25(OH)2D3 in inhibiting the proliferation of immortalized PZ-HPV-7 normal prostate cells and LNCaP prostate cancer cells, respectively.21,22 Moreover, MART-10 was 10-fold more active than 1α,25(OH)2D3 in inhibiting the invasion of PC-3 prostate cancer cells21 and trans-activation of VDRE in LNCaP cells.22 It has also been reported that the removal of 10(19)-methylene group from 1α,25(OH)2D3 eliminates the hydrophobic interactions with the ligand binding domain of VDR, and therefore reduces the VDR binding affinity relative to 1α,25(OH)2D3, as demonstrated by a 70% and 83% drop of VDR binding affinity for the porcine VDR23 and the calf thymus VDR,19 respectively. However, the addition of the 2α-(3-hydroxypropyl) group to 19-nor-1α,25(OH)2D3 molecule, that results in the formation of MART-10 analog, restores the VDR binding affinity to the level of 1α,25(OH)2D3.19
Work from Bouillon’s group reported that 14-epi-19-norvitamin D analogs, TX522 and TX527, possess a much enhanced antiproliferative action on breast cancer cells in vitro and in vivo, with much lower calcemic activity compared to 1α,25(OH)2D3.24 The X-ray crystallographic analysis of the VDR-TX522 complex revealed that TX522 binds to the hVDR with modified contacts of C12–Val300 and C22–Ile268 compared with 1α,25(OH)2D3, which may increase interactions between the VDR and coactivators.25 Therefore, we synthesized two C2-substituted 14-epi-19-norvitamin D3 analogs, 14-epi-MART-10 and 14-epi-MART-11, and studied their metabolism by human CYP24A1 in an attempt to explain their potent biological activities in vitro and in vivo.26
RESULTS AND DISCUSSION
The known 8-oxo-14-epi-CD-ring 427 with the 14S configuration was converted to sulfone 826 as shown in Scheme 1. The tertiary alcohol 4 was treated with MOM-Cl to give 5 in 90% yield, and the subsequent two-carbon unit elongation at the C8 position was accomplished through Horner-Wadsworth-Emmons reaction, yielding α,β-unsaturated ester 6 in 92%. The E/Z ratio of the olefinination was ca. 50/1 and the stereochemistry of the major isomer 6 was determined by NOE experiments, in which NOEs were observed between vinylic H7 and H14 and also between H14 and H18. The ester 6 was reduced by DIBAL-H in toluene to yield allylic alcohol 7 in 99% yield. The subsequent sulfonylation with 2-mercaptobenzothiazole under Mitsunobu conditions followed by molybdenum(VI)-catalyzed oxidation afforded sulfone 8 in 82% yield, which was the substrate for the Julia coupling reaction.28-30
The A-ring part ketone 919 and sulfone 8 were coupled using LiHMDS in THF, and then the protecting groups of the coupling products were removed under acidic conditions to obtain 14-epi-2-(3-hydroxypropyl)-19-norvitamin D3 (10) as a diastereoisomeric mixture due to the C2 stereochemistry in 38% yield in two steps (Scheme 2). The products were converted to pivalates 2α-11 and 2β-11, which could be separated from each other (2α:2β = 2.4:1) by HPLC. The stereochemistries of the C2 position of 2α-11 and 2β-11 were determined by 1H NMR experiments including NOE observation as shown in Figure 2.19 Finally, the pivaloyl group was removed from each isomer under basic conditions to obtain the target molecules, 14-epi-MART-10 or 14-epi-MART-11.
In the previous report, we described biological activities of 14-epi-MART-10 and 14-epi-MART-11 such as VDR binding affinity (27% and 3% of 1, respectively), inducing activity of HL-60 cell differentiation (794% and 87% of 1), potent antiproliferative activity in PZ-HPV-7 cells (14-epi-MART-10 showed nearly 10-50 times more active than 1), transactivation activity of the osteocalcin promoter in HOS (human osteoblast cell line) cells (388% and 54% of 1), and effects on bone mineral density (BMD) as well as serum and urine calcium concentrations of ovariectomized (OVX) rats.26,31 14-epi-MART-10 at 0.1 µg/kg/day induced a marked increase in BMD in the OVX rats without significant calcemic and calciurinic side-effects, although at the higher dose, such as 1.0 µg/kg/day, a hypercalcemia became evident.
CYP24A1-dependent 24-hydroxylation is the first step in the degradation and the termination of various biological activities of 1α,25(OH)2D3 and its analogs. Thus, we examined the metabolism of 14-epi-MART-10 and 14-epi-MART-11 by human CYP24A1 in a reconstituted cell free system. A single metabolite, a putative 24-hydroxy product based on LC/MS analysis, was observed in the metabolism of 14-epi-MART-10, 14-epi-MART-11, and MART-10 (data not shown). Our previous study revealed that MART-10 is a poor substrate of CYP24A1 based on the fact that the kcat/Km value of MART-10 is only 0.2% of that of 1α,25(OH)2D3.22 The 3-hydroxypropyl group at C2 and the deletion of the methylene group at C19 in the MART-10 molecule may hinder the binding of MART-10 to the catalytic pocket of CYP24A1. Table 1 shows kinetic parameters of CYP24A1-dependent hydroxylation toward MART-10, 14-epi-MART-10 and 14-epi-MART-11. Although kcat values of three substrates are similar, Km values of 14-epi-MART-10 and 14-epi-MART-11 are significantly higher than that of MART-10, suggesting that both 14-epi compounds have lower affinity for CYP24A1 than MART-10. Conformational difference of 14-epi-MART-10 from MART-10 might explain the higher Km value of 14-epi-MART-10. It is noted that kcat/Km value of 14-epi-MART-10 is less than 0.1% of 1α,25(OH)2D3. These results indicate much less degradation of 14-epi-MART-10 and 14-epi-MART-11 by human CYP24A1. This could explain their greater biological activity than 1α,25(OH)2D3 in vitro and in vivo in spite of its lower VDR binding affinity.
CONCLUSION
Two new C2 substituted 14-epi-19-norvitamin D3 analogs, 14-epi-MART-10 and 14-epi-MART-11, were synthesized using Julia coupling reaction between C5 of A-ring and C6 of 14-epi-CD-ring (steroid numbering). The metabolic studies using human CYP24A1 in a reconstituted cell-free system indicate that both analogs have lower binding affinity for human CYP24A1 than MART-10. The results suggest that the epimerization at C-14 position further increases their resistance to the metabolic degradation of the side chain by CYP24A1,22 and may provide a mechanism for their super agonistic actions in vitro and in vivo.
EXPERIMENTAL
(1S,6R,7R)-7-[(R)-6-Methoxymethoxy-6-methylheptan-2-yl]-6-methylbicyclo[4.3.0]nonan-2-one (5).
The tertiary alcohol 4 (380 mg, 1.34 mmol) was dissolved in CH2Cl2 (8 mL), and i-Pr2NEt (1.2 mL, 6.8 mmol) and MOMCl (0.41 mL, 5.4 mmol) were added at 0 ºC. After stirring at rt for 18 h, the mixture was partitioned between H2O and CH2Cl2, and the organic layer was washed with 1 M aqueous HCl, H2O, and brine, successively, dried over MgSO4, filtered, and evaporated. The residue was purified by silica gel column chromatography (hexane/EtOAc = 6:1) to give 390 mg of 5 as a colorless oil in 90% yield. [α]D21 +45.6 (c 1.19, CHCl3); 1H NMR (400 MHz, CDCl3): δ 0.91 (3H, d, J = 6.4 Hz), 1.08 (3H, s), 1.24 (6H, s), 0.91-1.59 (8H, m), 1.73-1.93 (6H, m), 2.15-2.17 (1H, m), 2.27-2.34 (3H, m), 3.36 (3H, s), 4.70 (2H, s); 13C NMR (100 MHz, CDCl3): δ 19.2, 20.2, 21.4, 23.3, 26.5, 27.9, 29.9, 34.6, 36.3, 40.4, 42.5, 49.0, 50.8, 55.4, 55.4, 61.7, 61.8, 76.7, 91.4, 214.6; IR (neat): 2948, 2876, 1709, 1146, 1094, 1044, 918, 756, 723, 710 cm-1; EIMS: 324 (M+); HREIMS: calcd for C20H36O3 324.2664, found 324.2658.
Ethyl {(1S,2E,6R,7R)-7-[(R)-6-Methoxymethoxy-6-methylheptan-2-yl]-6-methylbicyclo[4.3.0]nonan-2-ylidene}acetate (6).
To a suspension of NaH (60% in oil, 370 mg, ca. 9.2 mmol) in THF (10 mL) was added (EtO)2P(O)CH2CO2Et (2.4 g, 11 mmol) at 0 ºC, and the mixture was stirred at rt overnight. The solution of ketone 5 (500 mg, 1.54 mmol) in THF (5 mL) was added at 0 ºC, and the mixture was stirred at rt for 4 days. The reaction mixture was poured into saturated aqueous NH4Cl, and extracted with EtOAc. The combined extracts were washed with saturated aqueous NH4Cl and brine, dried over MgSO4, filtered, and evaporated. The residue was purified by silica gel column chromatography (hexane/EtOAc = 9:1) to give 558 mg of 6 as a colorless oil in 92% yield. [α]D21 +34.8 (c 1.32, CHCl3); 1H NMR (400 MHz, CDCl3): δ 0.89 (3H, d, J = 6.4 Hz), 0.95 (3H, s), 0.83-1.65 (24H, m), 1.66-1.73 (3H, m), 1.74-1.91 (1H, m), 2.18 (1H, t, J = 8.8 Hz), 2.35-2.47 (1H, m), 3.28 (1H, ddd, J = 4.8, 4.8, 16.8 Hz), 3.36 (3H, s), 4.09-4.38 (2H, m), 4.76 (2H, s), 5.65 (1H, s); 13C NMR (100 MHz, CDCl3): δ 14.4, 19.7, 21.5, 22.0, 22.2, 26.0, 26.4, 27.5, 30.0, 34.0, 34.9, 37.4, 42.3, 46.3, 54.4, 55.1, 58.0, 59.4, 76.3, 90.9, 114.5, 164.9, 166.6; IR (neat): 2953, 1715, 1640, 1466, 1171, 1146, 1095, 1040, 918, 864, 729, 637 cm-1; EIMS: 394 (M+); HREIMS: calcd for C24H42O4 394.3083, found 394.3085.
2-{(1S,2E,6R,7R)-7-[(R)-6-Methoxymethoxy-6-methylheptan-2-yl]-6-methylbicyclo[4.3.0]nonan-2-ylidene}ethanol (7).
To the toluene solution (7 mL) of ester 6 (552 mg, 1.40 mmol) was added DIBAL-H (0.99 M solution in toluene, 3.5 mL, 3.5 mmol) at –78 ºC. After 1 h stirring at the same temperature, the reaction was quenched by adding saturated aqueous Na2SO4. The mixture was extracted with EtOAc, and the combined extracts were washed with brine, dried over MgSO4, filtered, and concentrated in vacuo. The residue was purified by silica gel column chromatography (hexane/EtOAc = 4:1) to yield 488 mg of 8 as a colorless oil in 99% yield. [α]D21 +26.5 (c 1.08, CHCl3); 1H NMR(400 MHz, CDCl3): δ 0.77-0.82 (6H, m), 0.87 (3H, s), 1.14 (6H, s), 1.17-1.23 (6H, m), 1.26-1.62 (12H, m), 1.74-1.82 (1H, m), 1.97-2.03 (1H, m), 2.18-2.25 (1H, m), 3.30 (3H, s), 4.02-4.14 (2H, m), 4.64 (2H, s), 5.33 (1H, t, J = 6.4 Hz); 13C NMR (100 MHz, CDCl3): δ 14.2, 19.8, 21.1, 21.6, 22.5, 24.7, 26.3, 26.4, 27.0, 29.2, 34.1, 34.7, 42.2, 45.0, 54.2, 55.1, 57.0, 58.8, 60.4, 76.3, 90.9, 122.3, 143.8; IR (neat): 2948, 2876, 1709, 1146, 1094, 1044, 918, 756, 723, 710 cm-1; EIMS: 352 (M+); HREIMS: calcd for C22H40O3 352.2977, found 352.2978.
(1S,2E,6R,7R)-2-[2-(Benzothiazole-2-sulfonyl)ethyliden]-7-[(R)-6-methoxymethoxy-6-methylheptan-2-yl]-6-methylbicyclo[4.3.0]nonane (8).
To a solution of 2-mercaptobenzothiazole (209 mg, 1.25 mmol) and PPh3 (328 mg, 1.25 mmol) in CH2Cl2 (2.7 mL) were added a solution of 7 (293 mg, 0.832 mmol) in CH2Cl2 (2.7 mL) and DIAD (0.36 mL, 1.25 mmol) at 0 ºC. After 2 h stirring, the mixture was concentrated, and the residue was dissolved in EtOH (5 mL), and to the solution were added 30% H2O2 (2.7 mL) and (NH4)6Mo7O24・4H2O (265 mg, 0.215 mmol) at 0 ºC with stirring. After stirring at rt for 2 h, the mixture was poured into saturated aqueous Na2SO4 and was extracted with EtOAc. The extracts were combined and washed with brine, dried over MgSO4, filtered, and concentrated in vacuo. The residue was purified by silica gel column chromatography (hexane/EtOAc = from 20:1 to 3:1) to give 362 mg of 8 as a colorless oil in 82% yield. [α]D21 +24.2 (c 1.02, CHCl3); 1H NMR (400 MHz, CDCl3): δ 0.73 (3H, s), 0.78 (3H, d, J = 6.6 Hz), 0.85-1.60 (15H, m), 1.21 (6H, s), 1.70-1.78 (1H, m), 1.86 (1H, ddd, J = 4.9, 9.8, 14.6 Hz), 2.07 (1H, dd, J = 8.5, 9.0 Hz), 2.15 (1H, ddd, J = 4.9, 4.9, 14.6 Hz), 3.37 (3H, s), 4.23 (1H, dd, J = 7.8, 14.4 Hz), 4.31 (1H, dd, J = 8.3, 14.4 Hz), 4.71 (2H, s), 5.23 (1H, dd, J = 7.8, 8.3 Hz), 7.59 (1H, ddd, J = 1.2, 7.8, 8.1 Hz), 7.64 (1H, ddd, J = 1.2, 8.1, 8.3 Hz), 8.00 (1H, ddd, J = 0.7, 1.2, 7.8 Hz), 8.22 (1H, ddd, J = 0.7, 1.2, 8.3 Hz); 13C NMR (100 MHz, CDCl3): δ 19.6, 21.3, 21.6, 21.6, 21.9, 25.0, 26.3, 26.4, 26.4, 26.8, 29.1, 34.1, 34.6, 37.2, 42.3, 45.5, 54.1, 54.3, 55.2, 57.3, 60.6, 76.5, 91.2, 107.5, 122.7, 125.7, 128.0, 128.3, 137.4, 153.0, 153.3, 166.4; IR (neat): 3065, 2936, 2361, 2330, 1740, 1470, 1425, 1331, 1150, 1084, 1035, 916, 853, 758, 729, 710, 689, 654, 635 cm-1; EIMS: 533 (M+); HREIMS: calcd for C29H43O4NS2 533.2634, found 533.2636.
14-Epi-2-(3-hydroxypropyl)-1α,25-dihydroxy-19-norvitamin D3 (a mixture of 14-epi-MART-10 and 14-epi-MART-11).
To a solution of 8 (49.3 mg, 0.092 mmol) in dry THF (800 μL) was added LiHMDS (1 M solution in THF, 90 μL, 90 μmol) at –78 ºC under argon. After stirring at the same temperature for 60 min, a solution of 9 (35.0 mg, 0.066 mmol) in dry THF (800 μL) was added dropwise to the mixture. After stirring for 2 h, the reaction mixture was quenched by addition of saturated aqueous NH4Cl, and the mixture was extracted with ether. The organic layer was washed with brine, dried over MgSO4, and filtered. After evaporation of the solvent, the crude mixture was applied on silica gel column chromatography (hexane/EtOAc = from 50:1 to 10:1) to give the crude protected vitamin D mixture. To the solution of the mixture in dry MeOH (0.5 mL) was added (+)-CSA (31 mg, 0.13 mmol) at 0 ºC under argon. After stirring at rt for 17 h, the reaction mixture was diluted with EtOAc. The resulting mixture was washed with saturated aqueous NaHCO3, brine, dried over MgSO4, filtered, and evaporated. Purification by silica gel column chromatography (EtOAc/EtOH = 10:1) gave 11.5 mg of diastereomixture 10 as white powder in 38% yield in two steps.
14-Epi-2α-(3-pivaloyloxypropyl)-1α,25-dihydroxy-19-norvitamin D3 (2α-11) and 14-epi-2β-(3- pivaloyloxypropyl)-1α,25-dihydroxy-19-norvitamin D3 (2β-11).
To the solution of 10 (49.2 mg, 0.106 mmol) in pyridine (0.6 mL) were added DMAP (1.3 mg, 0.01 mmol) and pivaloyl chloride (20 μL, 0.15 mmol), and the mixture was stirred at rt for 14 h. The mixture was diluted with EtOAc, and washed with H2O, 0.5 M aqueous CuSO4, H2O, and brine, successively, and dried over MgSO4, filtered, and concentrated in vacuo. The residue was purified by silica gel column chromatography (hexane/EtOAc = 1:1) to give 35.3 mg of the mixture of 2α-11 and 2β-11 in 61% yield. The mixture was separated by preparative HPLC (Shim-pack PREP-SIL (H)•Kit, 20 x 250 mm, 9.9 mL/min, hexane/EtOH = 9:1) to afford 23.0 mg of 2α-11 and 9.6 mg of 2β-11. 2α-11: [α]D23 +24.1 (c 0.26, CHCl3); 1H NMR (600 MHz, CDCl3): δ 0.88 (3H, d, J = 6.6 Hz), 0.92 (3H, s), 1.00-1.07 (1H, m), 1.20 (9H, s), 1.23 (6H, s), 1.22-1.93 (23H, m), 2.08-2.18 (4H, m), 2.47 (1H, ddd, J = 4.9, 4.9, 14.6 Hz), 2.60 (1H, dd, J = 4.7, 12.4 Hz), 2.90 (1H, dd, J = 3.6, 14.0 Hz), 3.62 (1H, ddd, J = 4.7, 9.9, 9.9 Hz), 4.09-4.12 (1H, m), 4.11 (2H, t, J = 6.6 Hz), 6.00 (1H, d, J = 11.3 Hz), 6.33 (1H, d, J = 11.3 Hz); 13C NMR (150 MHz, CDCl3): δ 19.9, 21.7, 21.9, 22.6, 23.9, 25.0, 26.4, 27.1, 27.4, 29.3, 29.6, 29.9, 29.9, 34.2, 34.8, 34.9, 39.0, 44.3, 44.7, 49.1, 54.9, 58.3, 64.9, 68.5, 71.4, 71.5, 119.3, 124.5, 132.0, 144.3, 179.9; IR (neat): 3431, 2957, 2918, 2847, 2361, 1730, 1718, 1458, 1366, 1289, 1165, 1049, 849 cm-1; EIMS: 546 (M+); HREIMS: calcd for C34H58O5 546.4284, found 546.4270. 2β-11: [α]D23 +13.9 (c 0.22, CHCl3); 1H NMR (600 MHz, CDCl3): δ 0.89 (3H, d, J = 6.6 Hz), 0.93 (3H, s), 1.03-1.10 (1H, m), 1.20 (9H, s), 1.22 (3H, s), 1.23 (3H, s), 1.22-1.93 (24H, m), 2.06-2.12 (1H, m), 2.14 (1H, dd, J = 8.0, 10.5 Hz), 2.34 (1H, ddd, J = 1.4, 3.9, 13.5 Hz), 2.41 (1H, d, J = 13.5 Hz), 2.47 (1H, ddd, J = 4.7, 4.9, 14.6 Hz), 3.11 (1H, dd, J = 3.6, 12.9 Hz), 3.54 (1H, ddd, J = 4.7, 10.5, 10.5 Hz), 4.05 (1H, ddd, J = 2.8, 2.8, 3.0 Hz), 4.10 (2H, t, J = 6.6 Hz), 6.05 (1H, d, J = 11.3 Hz), 6.19 (1H, d, J = 11.3 Hz); 13C NMR (150 MHz, CDCl3): δ 19.8, 21.8, 22.1, 22.4, 25.0, 26.5, 27.1, 27.4, 29.4, 29.5, 29.9, 30.1, 34.3, 34.7, 35.9, 38.1, 39.0, 44.7, 45.6, 45.9, 49.1, 54.9, 58.2, 64.9, 68.7, 71.5, 71.9, 119.2, 125.1, 131.9, 144.5, 197.9; IR (neat): 3447, 2957, 2920, 2361, 2341, 1717, 1701, 1163, 910 cm-1; EIMS: 546 (M+); HREIMS: calcd for C34H58O5 546.4284, found 546.4270.
14-Epi-2α-(3-hydroxypropyl)-1α,25-dihydroxy-19-norvitamin D3 (14-epi-MART-10).
Pivalate 2α-11 (3.0 mg, 0.005 mmol) was dissolved in the 10% NaOMe solution in MeOH at 0 ºC. After 1.5 h stirring at rt, Amberlyst® (H+ form) was added at 0 ºC for neutralization, followed by filtration, and the filtrate was concentrated in vacuo. The residue was purified by preparative thin layer chromatography on silica gel (EtOAc/EtOH = 1:1) to obtain 2.1 mg of 14-epi-MART-10 as a white powder in 91% yield. [α]D23 +5.9 (c 0.17, CHCl3); UV(EtOH): λmax 244.5, 252.5, 262.0 nm; λmin 247.5, 259.0 nm. 1H NMR (600 MHz, CDCl3): δ 0.88 (3H, d, J = 6.6 Hz), 0.92 (3H, s), 1.00-1.08 (1H, m), 1.23 (6H, s), 1.22-1.88 (24H, m), 2.06-2.12 (3H, m), 2.16 (1H, d, J = 11.5 Hz), 2.47 (1H, ddd, J = 4.4, 4.4, 14.9 Hz), 2.60 (1H, dd, J = 4.7, 12.4 Hz), 2.90 (1H, dd, J = 4.1, 14.0 Hz), 3.65 (1H, ddd, J = 4.7, 9.9, 9.9 Hz), 3.71 (2H, t, J = 5.8 Hz), 4.11 (1H, ddd, J = 2.8, 2.8, 4.1 Hz), 6.01 (1H, d, J = 11.3 Hz), 6.32 (1H, d, J = 11.3 Hz); 13C NMR (150 MHz, CDCl3): δ 19.8, 21.7, 21.9, 22.3, 23.5, 24.8, 26.9, 29.2, 29.4, 30.0, 30.1, 34.1, 34.6, 35.6, 37.9, 44.4, 45.3, 45.5, 48.8, 54.6, 57.9, 63.0, 68.6, 71.1, 71.7, 118.5, 124.3, 131.3, 143.6; IR (neat): 3340, 2920, 1203, 1147, 1051, 801 cm-1; EIMS: 462 (M+); HREIMS: calcd for C29H50O4 462.3709, found 462.3712.
14-Epi-2β-(3-hydroxypropyl)-1α,25-dihydroxy-19-norvitamin D3 (14-epi-MART-11).
This compound was obtained by the same procedure as described above from pivalate 2β-11 (3.0 mg, 0.005 mmol). 14-epi-MART-11 (2.2 mg) was obtained as a white powder in 95% yield. [α]D23 +4.3 (c 0.12, CHCl3); UV (EtOH): λmax 244.5, 252.5, 262.0 nm; λmin 247.5, 259.0 nm. 1H NMR (600 MHz, CDCl3): δ 0.89 (3H, d, J = 6.4 Hz), 0.93 (3H, s), 1.03-1.10 (1H, m), 1.22 (6H, s), 1.22-1.93 (25H, m), 2.06-2.12 (1H, m), 2.14 (1H, dd, J = 8.0, 10.0 Hz), 2.35 (1H, dd, J = 3.5, 13.7 Hz), 2.41 (1H, d, J = 13.7 Hz), 2.46 (1H, ddd, J = 4.7, 4.9, 14.6 Hz), 3.11 (1H, dd, J = 4.2, 12.7 Hz), 3.57 (1H, ddd, J = 4.7, 10.0, 10.0 Hz), 3.70 (2H, t, J = 5.6 Hz), 4.05 (1H, ddd, J = 2.9, 2.9, 3.2 Hz), 6.05 (1H, d, J = 11.3 Hz), 6.19 (1H, d, J = 11.3 Hz); 13C NMR (150 MHz, CDCl3): δ 19.8, 21.7, 21.8, 22.5, 23.8, 24.9, 27.0, 29.2, 29.5, 29.8, 30.0, 34.1, 34.6, 37.9(2C), 44.0, 44.5, 45.4, 49.0, 54.6, 58.0, 63.0, 68.5, 71.1(2C), 118.5, 123.6, 131.2, 143.2; IR (neat): 3335, 2926, 1456, 1375, 1201, 1147, 1049, 800 cm-1; EIMS: 462 (M+); HREIMS: calcd for C29H50O4 462.3709, found 462.3715.
Metabolism of 14-epi-MART-10 and 14-epi-MART-11 by human CYP24A1 (24R-OHase) in a reconstituted cell-free system
CYP24A1-dependent metabolism of 14-epi-MART-10 and 14-epi-MART-11 were measured in a reconstituted system containing the membrane fraction.32-34 The metabolism of MART-10 was also measured to compare it with those of the 14-epi compounds. The activities were measured under the conditions as follows: 0.05 µM human CYP24A1, 2.0 µM ADX, 0.2 µM ADR, 0 to 20 µM 14-epi-MART-10 or 14-epi-MART-11 or MART-10 in 100 mM Tris-HCl (pH 7.4) and 1 mM EDTA at 37 °C. The reaction was initiated by the addition of NADPH at a final concentration of 1 mM. Aliquots of the reaction mixture were collected at various time-intervals and extracted with 4 volumes of chloroform-methanol (3:1). The organic phase was recovered and dried under reduced pressure. The resultant residue was dissolved in MeCN and applied to HPLC under the following conditions: column, YMC-Pack ODS-AM (5 µm) (4.6 mm x 300 mm) (YMC Co., Kyoto, Japan); UV detection, 254 nm; flow-rate, 1.0 mL min-1; column temperature, 40 °C; mobile phase, a linear gradient of 20-100% MeCN aqueous solution for the first 25 min followed by 100% MeCN for 10 min for the isolation and identification of 14-epi-MART-10 and 14-epi-MART-11, MART-10, and their metabolites.
ACKNOWLEDGEMENTS
We thank Ms. Junko Shimode, Ms. Akiko Tonoki, and Ms. Ayako Kawaji (Teikyo University) for the spectroscopic measurements. This study was supported in part by Grants-in-Aid for Scientific Research (B) and (C) from the Japan Society for the Promotion of Science (No. 21380067 to TS and No. 21590022 to AK, respectively).
References
1. A. J. Brown and E. Slatopolsky, Mol. Asp. Med., 2008, 29, 433. CrossRef
2. R. M. Evans, Science, 1988, 240, 889. CrossRef
3. For selective binding of coactivators to VDR-(synthetic ligand) complexes, see: M. A. Arai, K. Takeyama, S. Ito, S. Kato, T. C. Chen, and A. Kittaka, Bioconjugate Chem., 2007, 18, 614. CrossRef
4. K. K. Deeb, D. L. Trump, and C. S. Johnson, Nature Rev. Cancer, 2007, 7, 684. CrossRef
5. G. H. Posner and M. Kahraman, 'Vitamin D,' 2nd ed., ed. by D. Feldman, J. W. Pike, and F. H. Glorieux, Elsevier Academic Press, New York, 2005, pp. 1405-1422. CrossRef
6. N. Saito, S. Honzawa, and A. Kittaka, Curr. Top. Med. Chem., 2006, 6, 1273. CrossRef
7. N. Saito, Y. Suhara, M. Kurihara, T. Fujishima, S. Honzawa, H. Takayanagi, T. Kozono, M. Matsumoto, M. Ohmori, N. Miyata, H. Takayama, and A. Kittaka, J. Org. Chem., 2004, 69, 7463. CrossRef
8. E. Takahashi, K. Nakagawa, Y. Suhara, A. Kittaka, K. Nihei, K. Konno, H. Takayama, K. Ozono, and T. Okano, Biol. Pharm. Bull., 2006, 29, 2246. CrossRef
9. A. Kittaka, Yakugaku Zasshi, 2008, 128, 1235. CrossRef
10. S. Honzawa, N. Takahashi, A. Yamashita, T. Sugiura, M. Kurihara, M. A. Arai, S. Kato, and A. Kittaka, Tetrahedron, 2009, 65, 7135. CrossRef
11. S. Hourai, T. Fujishima, A. Kittaka, Y. Suhara, H. Takayama, N. Rochel, and D. Moras, J. Med. Chem., 2006, 49, 5199. CrossRef
12. N. Saito and A. Kittaka, ChemBioChem, 2006, 7, 1478. CrossRef
13. N. Saito, T. Matsunaga, H. Saito, M. Anzai, K. Takenouchi, D. Miura, J. Namekawa, S. Ishizuka, and A. Kittaka, J. Med. Chem. 2006, 49, 7063. CrossRef
14. N. Saito and A. Kittaka, J. Synth. Org. Chem. Japan, 2007, 65, 947.
15. R. R. Sicinski, P. Rotkiewics, A. Kolinski, W. Sicinska, J. M. Prahl, C. M. Smith, and H. F. DeLuca, J. Med. Chem., 2002, 45, 3366. CrossRef
16. The original report of 19-norvitamin D3, see: K. L. Perlman, R. R. Sicinski, H. K. Schnoes, and H. F. DeLuca, Tetrahedron Lett., 1990, 31, 1823. CrossRef
17. R. Barycki, R. R. Sicinski, L. A. Plum, P. Grzywacz, M. Clagett-Dame, and H. F. DeLuca, Bioorg. Med. Chem., 2009, 17, 7658. CrossRef
18. M. Shimizu, Y. Miyamoto, H. Takaku, M. Matsuo, M. Nakabayashi, H. Masuno, N. Udagawa, H. F. DeLuca, T. Ikura, and N. Ito, Bioorg. Med. Chem., 2008, 16, 6949. CrossRef
19. K. Ono, A. Yoshida, N. Saito, T. Fujishima, S. Honzawa, Y. Suhara, S. Kishimoto, T. Sugiura, K. Waku, H. Takayama, and A. Kittaka, J. Org. Chem., 2003, 83, 7407. CrossRef
20. M. A. Arai and A. Kittaka, Anticancer Res. 2006, 26, 2621.
21. T. C. Chen, K. S. Persons, S. Zheng, J. Mathieu, M. F. Holick, Y. F. Lee, B. Bao, M. A. Arai, and A. Kittaka, J. Steroid Biochem. Mol. Biol., 2007, 103, 717. CrossRef
22. J. N. Flanagan, S. Zheng, K.-C. Chiang, A. Kittaka, T. Sakaki, S. Nakabayashi, R. A. Spanjaard, K. S. Persons, J. S. Mathieu, M. F. Holick, and T. C. Chen, Anticancer Res. 2009, 29, 3547.
23. X. Zhou, G.-D. Zhu, D. Van Haver, M. Vandewalle, P. J. De Clercq, A. Verstuyf, and R. Bouillon, J. Med. Chem. 1999, 42, 3539. CrossRef
24. L. Verlinden, A. Verstuyf, M. Van Camp, S. Marcelis, K. Sabbe, X.-Y. Zhao, P. De Clercq, M. Vandewalle, and R. Bouillon, Cancer Res., 2000, 60, 2673.
25. G. Eelen, L. Verlinden, N. Rochel, F. Claessens, P. De Clercq, M. Vandewalle, G. Tocchini- Valentini, D. Moras, R. Bouillon, and A. Verstuyf, Mol. Pharmacol. 2005, 67, 1566. CrossRef
26. Synthetic route to 14-epi-MART-10 & -11 and their biological activities, i.e., VDR binding affinity, HL-60 cell differentiation activity, antiproliferative activity in PZ-HPV-7 cells, transactivation activity of the osteocalcin promoter in HOS cells, and effects on bone mineral density as well as the blood and urine calcium concentrations of ovariectomized rats, were reported in the proceedings of the symposium “Vitamin D Analogs in Cancer Prevention and Therapy”, May 2008, Krefeld, Germany; A. Kittaka, H. Hara, M. Takano, D. Sawada, M. A. Arai, K. Takagi, T. Chida, Y. Harada, H. Saito, K. Takenouchi, S. Ishizuka, K. Hayashi, S. Ikushiro, T. Sakaki, T. Sugiura, and T. C. Chen, Anticancer Res., 2009, 29, 3563.
27. D. F. Maynard, W. G. Trankle, A. W. Norman, and W. H. Okamura, J. Med. Chem. 1994, 37, 2387. CrossRef
28. A. Yoshida, K. Ono, Y. Suhara, N. Saito, H. Takayama, and A. Kittaka, Synlett, 2003, 1175. CrossRef
29. M. A. Arai, R. Tsutsumi, H. Hara, T. C. Chen, T. Sakaki, N. Urushino, K. Inouye, and A. Kittaka, Heterocycles, 2005, 66, 469. CrossRef
30. Julia Olefination synthesizing retiferol (Ro 65-2299), see: H. Hilpert and B. Wirz, Tetrahedron, 2001, 57, 681. CrossRef
31. 14-epi-19-Nor-1α,25(OH)2D3 without 2-substituent showed weak biological activity as compared with 1α,25(OH)2D3, see: ref. 26.
32. N. Saito, Y. Suhara, D. Abe, T. Kusudo, M. Ohta, K. Yasuda, T. Sakaki, S. Honzawa, T. Fujishima, and A. Kittaka, Bioorg. Med. Chem., 2009, 17, 4296. CrossRef
33. D. Abe, T. Sakaki, T. Kusudo, A. Kittaka, N. Saito, Y. Suhara, T. Fujishima, H. Takayama, H. Hamamoto, M. Kamakura, M. Ohta, and K. Inouye, Drug Metab. Dispos., 2005, 33, 778. CrossRef
34. T. Kusudo, T. Sakaki, D. Abe, T. Fujishima, A. Kittaka, H. Takayama, S. Hatakeyama, M. Ohta, and K. Inouye, Biochem. Biophys. Res. Commun., 2004, 321, 774. CrossRef