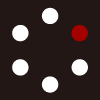
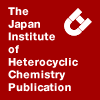
HETEROCYCLES
An International Journal for Reviews and Communications in Heterocyclic ChemistryWeb Edition ISSN: 1881-0942
Published online by The Japan Institute of Heterocyclic Chemistry
e-Journal
Full Text HTML
Received, 17th May, 2010, Accepted, 20th July, 2010, Published online, 21st July, 2010.
DOI: 10.3987/COM-10-S(E)33
■ Diastereoselective Cyclization Reactions of Chiral Proline Auxiliary-Substituted N-Benzoyl-α-dehydro(1-naphthyl)alaninamide Derivatives via Photoinduced Electron Transfer
Yuhki Sato, Yuhta Haruyama, Tetsutaro Igarashi, and Tadamitsu Sakurai*
Material and Life Chemistry, Faculty of Engineering, Kanagawa University, 3-27-1 Rokkakubashi, Kanagawa-ku, Yokohama 221-8686, Japan
Abstract
Irradiation of the title 1-naphthylalaninamide derivatives [(Z)-1] bearing N’-substituted (S)-prolinamide auxiliaries in 1,2-dichloroethane and methanol containing triethylamine mainly afforded the corresponding (4S,5S)-4,5-dihydrooxazoles [(4S,5S)-2, diastereomeric excess (de) = 21–84%] and (4R,5R)-2 (de = 18–43%), respectively. Analysis of substituent and solvent effects on the diastereoselective photocyclization of (Z)-1 substantiated that steric bulkiness of the chiral auxiliary, solvent polarity, and intramolecular hydrogen bond are major factors controlling de, while intermolecular hydrogen-bonding and charge-transfer interactions invert the configuration of the dihydrooxazole diastereomer preferentially formed.INTRODUCTION
Recent research in photochemistry has focused on diastereoselective and enantioselective photocyclization reactions in order to develop a new methodology for control of the chirality of pharmaceutically useful heterocyclic rings.1 In the course of a systematic study on the photoinduced electron transfer (PET)-initiated cyclization of chiral auxiliary-substituted N-acyl-α-dehydro(1-naphthyl)alaninamides, we found that these 1-naphthylalaninamide derivatives undergo an asymmetric photocyclization to afford the corresponding 3,4-dihydrobenzo[f]quinolinones in high diastereomeric excess (de).2 It was shown in this diastereoselective photocyclization that the magnitude of de strongly depends on the structure of chiral auxiliary as well as on temperature. We also observed the occurrence of a similar PET-initiated diastereoselective cyclization (selectively giving cis- and trans-4,5-dihydrooxazole derivatives with high efficiencies) in some chiral auxiliary-substituted N-benzoyl-α-dehydro(1-naphthyl)alanine alkyl esters.3a Because naphthylalanine alkyl ester-derived cis-4,5-dihydrooxazoles have already been found to undergo triethylamine (TEA)-catalyzed isomerizations readily to the thermodynamically more stable trans-isomers,4 there were some restrictions on the use of tertiary amine, solvent, and the starting 1-naphthylalanine alkyl ester for the asymmetric photocyclization.
In a previous paper we demonstrated that replacement of the alkoxycarbonyl group in this 1-naphthylalanine alkyl ester by the N’,N’-disubstituted aminocarbonyl completely suppresses the above-described TEA-catalyzed isomerization although the amidation of the alkoxycarbonyl moiety induces a great decrease in photoreactivity.5 In addition, PET-initiated cyclization of N-benzoyl-α-dehydronaphthylalanine N’,N’-dimethylamide preferentially gave the corresponding cis-4,5-dihydrooxazole isomer. Thus, on the basis of the previous finding that an increase in steric bulkiness of the alkoxycarbonyl group enhances selectivity for the cis-isomer,4 we may predict that introducing a sterically congested chiral auxiliary group into the alaninamide nitrogen enables selective formation of the corresponding (4S,5S)- and (4R,5R)-diastereomers and, hence, a more accurate and detailed analysis of the PET-initiated diastereoselective cyclization reactions. To fulfill this prediction we chose some N’-unsubstituted and N’-substituted (S)-prolinamides as bulky chiral auxiliaries and synthesized (Z)-N-benzoyl-α-dehydro(1-naphthyl)alanylprolinamide derivatives [(Z)-1a–g] (Chart 1). In the present communication we examined the effects of substituent (chiral auxiliary) and solvent on the extent to which either diastereomer is formed in excess with the aim of elucidating a detailed mechanism for the observed asymmetric photoinduction.
RESULTS AND DISCUSSION
The starting α-dehydronaphthylalanylprolinamides of the (Z)-configuration (1a–g) were prepared in good yields by the Knoevenagel-type condensation between 1-naphthaldehyde and N-benzoylglycine in acetic anhydride containing sodium acetate, followed by the ring-opening reactions of the resulting (Z)-4-(1-naphthylmethylene)-2-phenyl-5(4H)-oxazolone with equimolar amounts of the corresponding N’-unsubstituted, N’-substituted, and N’,N’-disubstituted prolinamides in DMF at room temperature. After a nitrogen-saturated methanol solution of (Z)-1a (4.0x10–3 mol dm–3, 500 mL) containing TEA (0.10 mol dm–3) was irradiated at wavelengths longer than 280 nm (Pyrex glass filter) from a 400 W high-pressure Hg lamp for 7 h at room temperature (conversion, 45%), the reaction mixture obtained was subjected to column chromatography or preparative thin layer chromatography over silica gel. We were able to isolate (4S,5S)- and (4R,5R)-4-[2-aminocarbonyl-(S)-pyrrolidin-1-ylcarbonyl]-5-(1-naphthyl)-2-phenyl-4,5-dihydrooxazoles [(4R,5R)-2a and (4S,5S)-2a] (having the vicinal coupling constants (J4,5) of 10.3 Hz in DMSO-d6) along with the (E)-isomer of 1a by this usual workup (Scheme 1). 1H NMR analysis of the reaction mixture revealed negligible formation of the corresponding (4S,5R)- and (4R,5S)-diastereomers which are predicted to give the J4,5 values of 6.8 Hz.3
This also demonstrates that no TEA-catalyzed isomerization of cis-2a to trans-2a takes place. On the basis of our previous finding that (4S,5S)- and (4R,5R)-4-(tert-butoxycarbonyl)-5-(1-naphthyl)-2-phenyl-4,5-dihydrooxazoles exhibit circular dichroism (CD) bands of positive and negative signs at about 220 nm, respectively,3 cis-2a giving a CD band of positive sign near 225 nm was assigned to the (4S,5S)-diastereomer and the corresponding CD band of negative sign was assigned to that of the (4R,5R)-diastereomer. Because each NMR signal for protons at 4- and 5-positions on the dihydrooxazole ring of these two diastereomers was detected at different positions (5.70 and 6.64 ppm for the former diastereomer and 5.61 and 6.73 ppm for the latter), diastereomeric excess (de) for 2a and its derivatives (2b–g) was estimated based on the area ratio of these ring proton signals.
To analyze the effects of the substituent R (introduced into the proline carboxyl moiety) on the de of cis-2 and the photoreactivity of 1, nitrogen-saturated methanol or 1,2-dichloroethane solutions of (Z)-1a–g (4.0x10–3 mol dm–3, 10 mL) containing TEA (0.10 mol dm–3) were irradiated (at wavelengths longer than 280 nm from a 400 W high-pressure Hg lamp) in parallel using a merry-go-round-type irradiation equipment for 5 h at room temperature. The irradiated solution was evaporated to dryness in vacuo affording the residual solid which was subjected to 1H NMR spectral analysis. In Table 1 are summarized substituent effects on the de value of the diastereomer 2 formed in excess as well as on the photoreactivity (conversion) of 1, estimated in 1,2-dichloroethane and methanol. As seen from comparison of the results for 2a, 2b, and 2c, de is increased with an increase in steric bulkiness of the substituent R (H→Me→t-Bu) in both of these solvents, being consistent with the previous result obtained for chiral menthyl auxiliary-substituted α-dehydronaphthylalanine alkyl esters.3a A maximum de was obtained for (4S,5S)-2e having R = NHC6H4(t-Bu)-2 in 1,2-dichloroethane (de = 84%). In addition, amidation of the proline carboxyl group with piperidine (1f) or morpholine (1g) resulted in an increase in the reactivity of the starting alaninamide 1 with a decrease in de for the corresponding (4S,5S)-diastereomers, estimated in 1,2-dichloroethane, when compared
with 1b having the less bulky methyl substituent. The latter observation suggests that the number of substituent attached to the prolinamide nitrogen is a factor controlling the magnitude of de in this aprotic solvent. Interestingly, on changing solvent from 1,2-dichloroethane to methanol, diastereomer possessing the opposite configuration, namely, (4R,5R)-2 was formed in excess in any PET-initiated cyclizations of (Z)-1. As proposed in a previous study,3a hydrogen-bonding interaction with a key intermediate involved is considered to exert a decisive effect on the configuration of this intermediate. This interaction also shows a clear tendency to diminish de for 2a–e having the proline N’-unsubstituted and N’-monosubstituted amide bonds and, in contrast, to enhance de for 2f and 2g having the N’,N’-disubstituted amide bond. As in 1,2-dichloroethane, disubstitution of the prolinamide nitrogen produced a large increase in the photoreactivity of 1 in the protic polar solvent, methanol.
We previously showed that PET-initiated reactions of chiral auxiliary-substituted α-dehydronaphthylalanine alkyl esters (that eventually give the corresponding 4,5-dihydrooxazole derivatives) proceed through a mechanism in which asymmetry at 4- and 5-positions on the dihydrooxazole ring is induced in either the cyclization of radical anions or the hydrogen shift of biradicals.3a Because no trans-dihydrooxazole isomer was formed in the photocyclization of (Z)-1a–g, we discuss a detailed mechanism of this stereoselective and diastereoselective photocyclization, in other words, major factors controlling the magnitude of de on the basis of Scheme 2. As described above, no formation of trans-2 proves that hydrogen shift in the biradicals IIIA and IIIB takes place stereoselectively, probably owing to steric hindrance of the 1-naphthyl group and the proline auxiliary and, hence, de for cis-2 is determined at the stage of cyclization of the radical ion pair intermediate (E)-I into the intermediates IIA and IIB. In this cyclization process, it is very likely that π-face selective intramolecular attack of the N-benzoyl carbonyl oxygen upon the olefinic carbon in the intermediate (E)-I is made so as to minimize steric hindrance caused by the bulky prolinamide moiety. Thus, the finding that the (4S,5S)-diastereomer is formed in excess in 1,2-dichloroethane confirms that the chiral auxiliary exists in the si face of the radical anion and the carbonyl oxygen attacks the olefinic carbon of (E)-I preferentially from the re face. Because hydrogen-bonding solvation in methanol results in a generation of (4R,5R)-2 as a major diastereomer, the above-mentioned intramolecular attack in this protic solvent should be made preferentially from the reverse face, namely, the si face. It is likely that methanol molecules (existing in the vicinity of (E)-I through hydrogen-bonding interaction) play a decisive role in controlling stereochemistry of the π-face selective attack of the carbonyl oxygen.
As already described, an increase in steric bulkiness for the chiral auxiliary group of (Z)-1 is clearly reflected in the enhanced de value in any solvents tested. Enhanced steric congestion around this chiral group existing in the si or re face is considered to suppress intramolecular cyclization from the same face to a more extent. In addition, it is of significance to explain the reason why the number of substituent attached to the prolinamide nitrogen exerts a great effect on de estimated in the aprotic solvent, 1,2-dichloroethane. Molecular modeling strongly suggests the formation of an intramolecular hydrogen bonding between the proline N-carbonyl oxygen and the prolinamide hydrogen in N’-unsubstituted and N’-monosubstituted (E)-I intermediates (Figure 1). Taking into account a decrease in conformational mobility of the bulky proline moiety with this hydrogen bonding, we propose that the hydrogen bond formation magnifies steric hindrance in the cyclization process from the si face to more accelerate this process from the re face. A protic solvent such as methanol is predicted to weaken an intramolecular hydrogen bonding and, hence, to diminish de for cis-2, as seen from comparison of de for (4S,5S)-2 (bearing the proline N’-monosubstituted and N’,N’-disubstituted amide bonds) in the aprotic solvent (Table 1). Results obtained are consistent with our prediction, making our interpretation of the effect of hydrogen-bonding interaction on de reasonable. Equally importantly, de for cis-2f and cis-2g having no ability to form the above intramolecular hydrogen bond was found to increase on changing solvent from 1,2-dichloroethane to methanol. This finding makes it highly probable that forming intermolecular hydrogen bond(s) with methanol also contributes to the achievement of high diastereoselectivity for cis-2, though the configuration of the dihydrooxazole diastereomer formed in excess is reversed.
To elucidate the role of solvent in the π-face selective intramolecular cycloaddition within (E)-I in more detail, we chose 1c and 1g as typical N-benzoyl-α-dehydro(1-naphthyl)alanylprolinamides [(Z)-1] containing the proline N’-monosubstituted and N’,N’-disubstituted amide bonds, respectively and explored the effects of aprotic and protic solvents on de for the cis-4,5-dihydrooxazole diastereomer formed in excess in a systematic way. Each nitrogen-saturated solution containing (Z)-1c or (Z)-1g (4.0×10–3 mol dm–3, 10 mL) and TEA (0.10 mol dm–3) was irradiated at λ> 280 nm for 5 h at room temperature in parallel using a merry-go-round-type irradiation equipment. Interestingly, the results collected in Table 2 show that while de for (4S,5S)-2c (in aprotic solvents) and (4R,5R)-2c (in protic solvents) is not much dependent on polarity in any solvent systems, properties of these two solvent systems exert dramatic effects on the magnitude of de for cis-2g as well as on the configuration of the major diastereomer produced. As described in the preceding section, intramolecular and intermolecular hydrogen bond formation in the radical ion pair intermediate (E)-I results in an enhancement of diastereoselectivity in the cyclization process from this intermediate. Furthermore, an increase in solvent polarity is expected to promote dissociation of the (E)-1-derived radical anion/TEA radical cation pair into a relatively free radical ion pair to result in a lowering of de. Thus, the finding that de for cis-2c is affected by changes in solvent properties to only a minor extent suggests that the above hydrogen bond formation strongly assists electrostatic interaction between the radical anion and the radical cation within (E)-I to inhibit dissociation of this radical ion pair even in acetonitrile and methanol. On the other hand, on increasing the polarity of protic solvents (t-BuOH< i-PrOH< MeOH),6 de for (4R,5R)-2g bearing the proline N’,N’-disubstituted
amide bond was reduced from 88% to 43%. Additionally, a large decrease in de for (4S,5S)-2 in acetonitrile was observed when the substituent R was changed from the tert-butylamino group to the morpholino group. These findings confirm that the (E)-1g-derived radical ion pair has a much stronger tendency to dissociate into a loose radical ion pair as compared to the 1c-derived one. The presence of the N’,N’-disubstituted amide bond having no amide hydrogen might be responsible for the appearance of this tendency. Suppresion of a decrease in de in methanol having almost the same polarity as acetonitrile is compatible with our expectation that hydrogen bonds with (E)-I assist the above-mentioned electrostatic interaction and render the dissociation of this radical ion pair intermediate more difficult.
The results in Table 2 also demonstrate that there is a marked tendency for de for cis-2g to increase as the polarity of chloro-substituted aprotic solvents is decreased (ClCH2CH2Cl> CH2Cl2> CHCl3)6 but the configuration of the diastereomer formed in excess is changed from (4S,5S)-2g in 1,2-dichloroethane to (4R,5R)-2g in dichloromethane and chloroform. These observations imply that in addition to the above electrostatic interaction, there should operate another interaction between the 1g-derived radical anion and the latter two solvents within the radical ion pair intermediate (E)-I. We propose a charge-transfer interaction within this radical ion pair on the basis of the facts that (4R,5R)-2g is formed as a major diastereomer also in protic solvents and carbon atoms in dichloromethane and chloroform bearing two and three electron-withdrawing chloro groups, respectively, are both electron deficient. These two aprotic halogen solvents are considered to play almost the same roles as protic solvents in controlling the π-face selectivity for intramolecular cycloaddition of the 1g-derived radical anion as well as the dissociation of this radical anion/TEA radical cation pair into their loose pair. Additional stabilization of such a radical ion pair through the charge-transfer interaction described above is reflected in a large increase in the photoreactivity of 1g in dichloromethane and chloroform.
EXPERIMENTAL
General methods
1H and 13C NMR spectra were taken with a JEOL JNM-A600 spectrometer. Chemical shifts were determined using tetramethylsilane as an internal standard. ESI-TOF mass spectra were measured with a JEOL JMS-T100LC AccuTOF mass spectrometer. Circular dichroism spectra were recorded on a Nihonbunko J-820 spectropolarimeter. TEA was fractionally distilled from sodium hydroxide. Methanol and acetonitrile were purified according to the standard procedure and freshly distilled prior to use.6 All other solvents were spectrophotometric grade and were used without further purification.
General procedure for the synthesis of (Z)-4-(1-naphthylmethylene)-2-phenyl-5(4H)-oxazolone
This oxazolone derivative was prepared from the Knoevenagel-type condensation reaction between 1-naphthaldehyde and N-benzoylglycine in acetic anhydride containing sodium acetate, described in a previous paper.5 The spectroscopic data of this derivative were consistent with those of the previously prepared sample.
General procedure for the synthesis of (S)-N-Benzyloxycarbonylprolinamide derivatives
After (S)-N-benzyloxycarbonylproline (12 mmol) and N-hydroxysuccinimide (13 mmol) were dissolved in 1,4-dioxane (50 mL), dicyclohexylcarbodiimide (13 mmol) was added to this dioxane solution and the resulting reaction mixture was stirred for 0.5 h at 0 °C and then for 5 h at rt. After removal of the precipitated N,N’-dicyclohexylurea, the filtrate was concentrated to dryness in vacuo. The resulting residual solid was dissolved in CHCl3 (50 mL) and washed twice with water (50 mLx2). The proline succinimido ester obtained by removing chloroform under reduced pressure was dissolved in 1,4-dioxane (50 mL) and allowed to react with the corresponding primary amines (16 mmol) for 1–3 h at rt or 100 °C. The reaction mixtures were concentrated to dryness under reduced pressure and dissolved in CHCl3 (50 mL). After washing with water (50 mLx2), the CHCl3 solutions were dried over anhydrous sodium sulfate and then concentrated to dryness in vacuo affording crystalline solids in more than 70% yields. 1H NMR spectral data of (S)-N-benzyloxycarbonylprolinamides purified by reprecipitation from EtOAc or EtOAc-hexane are as follows.
(S)-N-Benzyloxycarbonylprolinamide: 1H NMR (600 MHz, CDCl3) δ = 1.83–1.93 (2H, m), 2.20–2.30 (1H, m), 3.48–3.62 (2H, m), 4.30–4.40 (1H, m), 5.10–5.15 (2H, m), 6.26–6.30 (1H, m), 6.74 (2H, s), 7.25–7.38 (5H, m).
(S)-N-Benzyloxycarbonyl-N’-methylprolinamide: 1H NMR (600 MHz, CDCl3) δ = 1.84–2.00 (3H, m), 2.12–2.39 (1H, m), 2.75 (3H, d, J = 5.4 Hz), 3.44–4.52 (2H, m), 4.31–4.33 (1H, m), 5.06–5.18 (2H, m), 6.06–6.08 (1H, m), 7.28–7.40 (5H, m).
(S)-N-Benzyloxycarbonyl-N’-(tert-butyl)prolinamide: 1H NMR (600 MHz, CDCl3) δ = 1.15–1.39 (9H, s), 1.83–2.40 (3H, m), 3.35–3.69 (3H, m), 4.10–4.28 (1H, m), 4.98–5.28 (2H, m), 6.56 (1H, s), 7.28–7.42 (5H, m).
(S)-N-Benzyloxycarbonyl-N’-phenylprolinamide: 1H NMR (600 MHz, CDCl3) δ = 1.74–2.52 (4H, m), 3.35–3.62 (2H, m), 4.29–4.57 (1H, m), 5.06–5.28 (2H, m), 7.06–7.48 (10H, m), 9.18 (1H, s).
(S)-N-Benzyloxycarbonyl-N’-(o-tert-butylphenyl)prolinamide: It was very difficult to assign 1H NMR signals of this prolinamide owing to the coexistence of the cis- and trans-conformational isomers. For this reason, the benzyloxycarbonyl-protected prolinamide derivative was characterized after removal of the benzyloxycarbonyl group by catalytic hydrogenation: 1H NMR (600 MHz, CDCl3) δ = 1.32 (9H, s), 1.66–1.72 (2H, m), 1.90–2.18 (2H, m), 2.89–3.03 (2H, m), 3.80 (1H, dd, J = 5.1, 9.2 Hz), 7.01 (1H, dd, J = 7.9, 7.9 Hz), 7.13 (1H, dd, J = 7.9, 7.9 Hz), 7.29 (1H, d, J = 7.9 Hz), 7.76 (1H, d, J = 7.9 Hz), 9.97 (1H, s).
(S)-N-Benzyloxycarbonyl-N’,N’-(pentan-1,5-diyl)prolinamide: 1H NMR (600 MHz, CDCl3) δ = 1.27–2.18 (10 H, m), 3.20–3.35 (2H, m), 3.40–3.70 (4H, m), 4.70 (1H, dd, J = 3.4, 5.5 Hz), 5.00–5.24 (2H, m), 7.23–7.37 (5H, m).
(S)-N-Benzyloxycarbonyl-N’,N’-(3-oxapentan-1,5-diyl)prolinamide: 1H NMR (600 MHz, CDCl3) δ = 1.30–1.70 (2H, m), 1.83–1.92 (2H, m), 1.91–2.21 (2H, m), 3.23–3.44 (1H, m), 3.45–3.71 (7H, m), 4.73 (1H, dd, J = 3.4, 8.2 Hz), 5.04–5.11 (2H, m), 7.26–7.38 (5H, m).
General procedure for the synthesis of (Z)-N-benzoyl-α-dehydro(1-naphthyl)alaninamide derivatives [(Z)-1a–g]
(S)-N-Benzyloxycarbonylprolinamide derivative (2.0 mmol) and catalytic amounts of 10 wt% palladium charcoal were added to MeOH (20 mL) and the resulting solution was stirred under hydrogen atmosphere for 5 h at rt. The catalyst was filtered out and the filtrate obtained was concentrated to dryness in vacuo giving the deprotected prolinamide derivative. This derivative was dissolved in DMF (20 mL) and allowed to react with (Z)-4-(1-naphthylmethylene)-2-phenyl-5(4H)-oxazolone (2.0 mmol) for 5–7 h at rt. The reaction mixture was poured into a separatory funnel together with water (50 mL) and α-dehydro(1-naphthyl)alaninamide derivative contained in this mixture was extracted three times with CHCl3 (25 mLx3). The CHCl3 solution was washed with 2.0 mol dm–3 hydrochloric acid (25 mLx2), saturated aqueous solution of sodium hydrogencarbonate (25 mLx2), and then with saturated aqueous solution of sodium chloride (25 mLx2). After the solution was dried over anhydrous sodium sulfate, it was concentrated to dryness under reduced pressure and the resulting residue was subjected to column chromatography over silica gel (230 mesh, Merck) eluting with CHCl3 containing 4.8 vol% MeOH. The isolated 1-naphthylalaninamide derivative (Z)-1 was recrystallized or reprecipitated from MeOH-hexane affording colorless crystals in a 64–86% yield.
(Z)-2-Benzoylamino-N,N-[(S)-1-(aminocarbonyl)butan-1,4-diyl]-3-(1-naphthyl)-2-propenamide [(Z)-1a]: 1H NMR (600 MHz, CDCl3) δ = 2.02–2.10 (2H, m), 2.23–2.30 (1H, m), 2.35–2.40 (1H, m), 3.72–3.89 (2H, m), 4.69 (1H, dd, J = 2.9, 5.7 Hz), 5.41 (1H, s), 6.84 (1H, s), 7.35 (2H, dd, J = 7.4, 7.4 Hz), 7.49 (1H, dd, J = 7.4, 7.4 Hz), 7.53 (1H, d, J = 8.0 Hz), 7.55–7.59 (5H, m), 7.80 (1H, s), 7.89 (1H, d, J = 8.0 Hz), 7.93 (1H, dd, J = 6.8, 6.8 Hz), 7.98 (1H, dd, J = 6.8, 6.8 Hz), 8.12 (1H, s); 13C NMR (150 MHz, CDCl3) δ = 24.5, 29.7, 49.5, 60.7, 117.5, 124.4, 125.5, 126.5, 126.6, 126.8, 127.4 (2C), 128.7 (2C), 128.9, 129.3, 130.2, 131.2, 132.0, 132.3, 132.5, 133.9, 165.5, 165.8, 174.2. ESI-TOF-MS m/z calcd for C25H23N3O3: 414.1818 [M + H]+. Found: 414.1854.
(Z)-2-Benzoylamino-N,N-[(S)-1-(methylaminocarbonyl)butan-1,4-diyl]-3-(1-naphthyl)-2-propenamide [(Z)-1b]: 1H NMR (600 MHz, CDCl3) δ = 1.92–1.98 (2H, m), 2.08–2.16 (1H, m), 2.31–2.36 (1H, m), 2.84 (3H, d, J =5.1 Hz), 3.71–3.77 (1H, m), 3.84–3.89 (1H, m), 4.40–4.42 (1H, m), 6.82 (1H, s), 7.23–7.27 (2H, m), 7.41 (1H, dd, J = 7.4, 7.4 Hz), 7.49–7.57 (5H, m), 7.59 (1H, d, J = 6.8 Hz), 7.75–7.76 (1H, m), 7.89 (1H, d, J = 8.0 Hz), 7.92–7.98 (2H, m), 8.81 (1H, s); 13C NMR (150 MHz, CDCl3) δ = 24.2, 26.4, 29.6, 49.4, 61.0, 118.0, 124.3, 125.5, 126.4, 126.6, 126.7, 127.3 (2C), 128.5 (2C), 128.9, 129.2, 130.2, 131.1, 131.8, 132.1, 132.3, 133.8, 165.5, 165.7, 171.6. ESI-TOF-MS m/z calcd for C26H25N3O3: 428.1974 [M + H]+. Found: 428.1976.
(Z)-2-Benzoylamino-N,N-[(S)-1-(tert-butylaminocarbonyl)butan-1,4-diyl]-3-(1-naphthyl)-2-propenamide [(Z)-1c]: 1H NMR (600 MHz, CDCl3) δ = 1.23–1.33 (2H, m), 1.42 (9H, s), 1.90–2.00 (2H, m), 2.07–2.12 (1H, m), 2.23–2.28 (1H, m), 4.33 (1H, dd, J = 2.9, 5.7 Hz), 6.81 (1H, s), 7.15 (1H, s), 7.29 (2H, dd, J = 7.4, 7.4 Hz), 7.42 (1H, dd, J = 7.4, 7.4 Hz), 7.49 (1H, dd, J = 7.4, 7.4 Hz), 7.51–7.55(2H, m), 7.58 (2H, d, J = 7.4 Hz), 7.59 (1H, dd, J = 6.9, 6.9 Hz), 7.86 (1H, d, J = 8.0 Hz), 7.90 (1H, d, J = 6.9 Hz), 7.98 (1H, d, J = 6.9 Hz), 8.54 (1H, s); 13C NMR (150 MHz, CDCl3) δ = 24.6, 28.8 (3C), 31.6, 49.5, 51.3, 61.6, 117.8, 124.4, 125.5, 126.4, 126.6, 126.7, 127.3 (2C), 128.7 (2C), 128.9, 129.1, 130.5, 131.2, 132.0, 132.3, 132.7, 133.9, 165.0, 166.1, 170.5. ESI-TOF-MS m/z calcd for C29H31N3O3: 470.2444 [M + H]+. Found: 470.2488.
(Z)-2-Benzoylamino-N,N-[(S)-1-(anilinocarbonyl)butan-1,4-diyl]-3-(1-naphthyl)-2-propenamide [(Z)-1d]: 1H NMR (600 MHz, CDCl3) δ = 1.96–2.03 (2H, m), 2.14–2.22 (1H, m), 2.39–2.42 (1H, m), 3.75–3.81 (1H, m), 3.89–3.94 (1H, m), 4.40–4.42 (1H, m), 6.85 (1H, s), 7.05 (2H, dd, J = 7.4, 7.4 Hz), 7.09 (1H, dd, J = 7.4, 7.4 Hz), 7.26 (1H, dd, J = 7.4, 7.4 Hz), 7.30 (2H, dd, J= 7.4, 7.4 Hz), 7.51 (2H, d, J= 7.4 Hz), 7.52–7.55 (2H, m), 7.56 (1H, dd, J = 6.9, 6.9 Hz), 7.63 (1H, d, J = 6.9 Hz), 7.86 (2H, d, J = 7.4 Hz), 7.90 (1H, d, J = 8.0), 7.94 (1H, d, J = 6.9 Hz), 8.00 (1H, d, J = 6.9 Hz), 9.06 (1H, s), 9.29 (1H, s); 13C NMR (150 MHz, CDCl3) δ = 24.4, 29.9, 49.6, 61.6, 118.6, 120.5 (2C), 123.9, 124.3, 125.6, 126.3, 126.6, 126.8, 127.3 (2C), 128.5 (2C), 128.6 (2C), 129.0, 129.3, 130.3, 131.2, 131.5, 132.1, 132.3, 133.9, 138.6, 165.7, 166.3, 169.7. ESI-TOF-MS m/z calcd for C31H27N3O3: 490.2131 [M + H]+. Found: 490.2176.
(Z)-2-Benzoylamino-N,N-[(S)-1-(o-tert-butylphenylaminocarbonyl)butan-1,4-diyl]-3-(1-naphthyl)-2-propenamide [(Z)-1e]: 1H NMR (600 MHz, CDCl3) δ = 1.44 (9H, s), 2.21–2.06 (2H, m), 2.32–2.25 (1H, m), 2.52–2.46 (1H, m), 3.95–3.85 (2H, m), 4.87 (1H, dd, J = 4.1, 8.4 Hz), 6.86 (1H, s), 7.23–7.19 (2H, m), 7.26 (2H, dd, J = 7.6, 7.6 Hz), 7.34–7.31 (1H, m), 7.44–7.41 (2H, m), 7.46 (2H, d, J = 7.6 Hz), 7.51 (1H, dd, J = 7.6, 7.6 Hz), 7.57–7.54 (2H, m), 7.59 (1H, d, J = 7.6 Hz), 7.89 (1H, d, J = 7.6 Hz), 7.92 (1H, d, J = 6.3 Hz), 7.99 (1H, d, J = 6.3 Hz), 8.11 (1H, s), 9.04 (1H, s); 13C NMR (150 MHz, CDCl3) δ = 25.0, 28.6, 30.7 (3C), 34.9, 49.8, 61.5, 116.9, 124.4, 125.5, 126.4, 126.5, 126.6, 126.8, 126.9, 127.0, 127.2 (2C), 128.7 (2C), 128.8, 129.2, 130.2, 130.8, 131.2, 132.0, 132.4, 132.7, 133.9, 135.7, 145.8, 165.0, 166.8, 170.9. ESI-TOF-MS m/z calcd for C35H35N3O3: 546.2757 [M + H]+. Found: 546.2784.
(Z)-2-Benzoylamino-3-(1-naphthyl)-N,N-[(S)-1-(piperidinocarbonyl)butan-1,4-diyl]-2-propenamide [(Z)-1f]: 1H NMR (600 MHz, CDCl3) δ = 1.22–1.35 (1H, m), 1.60–1.75 (3H, m), 1.75–1.85 (1H, m), 2.07–2.17 (3H, m), 2.17–2.24 (1H, m), 3.20–3.33 (1H, m), 3.48–3.55 (2H, m), 3.66–3.72 (2H, m), 3.89–3.94 (1H, m), 4.19–4.24 (1H, m), 5.18–5.21 (1H, m), 7.01 (1H, s), 7.32 (2H, dd, J = 7.4, 7.4 Hz), 7.44–7.54 (4H, m), 7.57 (1H, d, J = 7.4 Hz), 7.61 (2H, d, J = 7.4 Hz), 7.71 (1H, s), 7.84 (1H d, J = 8.0 Hz), 7.89 (1H, d, J = 6.9 Hz), 8.06 (1H, d, J = 8.0 Hz); 13C NMR (150 MHz, CDCl3) δ = 24.7, 25.4, 25.7, 26.6, 29.6, 43.5, 46.9, 50.4, 56.2, 117.9, 124.9, 125.4, 126.3, 126.5, 126.8, 127.3 (2C), 127.4, 128.6 (2C), 128.7, 128.8, 130.9, 131.2, 132.2, 132.8, 133.8, 165.3, 165.6, 170.2. ESI-TOF-MS m/z calcd for C30H31N3O3: 482.2444 [M + H]+. Found: 482.2488.
(Z)-2-Benzoylamino-N,N-[(S)-1-(morpholinocarbonyl)butan-1,4-diyl]-3-(1-naphthyl)-2-propenamide [(Z)-1g]: 1H NMR (600 MHz, CDCl3) δ = 1.18–1.33 (1H, m), 1.54–1.58 (2H, m), 1.92–2.17 (3H, m), 2.30–2.45 (1H, m), 3.23–3.39 (1H, m), 3.49–3.73 (2H, m), 3.62–3.73 (2H, m), 3.87–3.92 (1H, m), 4.19–4.23 (1H, m), 5.16 (1H, dd, J = 7.4, 7.4 Hz), 7.00 (1H, s), 7.33 (2H, dd, J = 7.4, 7.4 Hz), 7.44 (1H, dd, J = 7.4, 7.4 Hz), 7.47–7.53 (2H, m), 7.57 (1H, dd, J = 6.9, 6.9 Hz), 7.61 (2H, d, J = 7.4 Hz), 7.83 (2H, d, J = 8.6 Hz), 7.88 (1H, dd, J = 3.4, 6.3 Hz), 8.03 (1H, dd, J = 3.4, 6.3 Hz), 8.13 (1H, s). ESI-TOF-MS m/z calcd for C29H29N3O4: 484.2236 [M + H]+. Found: 484.2239.
General procedure for the irradiation of (Z)-1a–g To isolate and characterize the (Z)-1-derived photoproducts, a nitrogen-saturated MeOH solution of (Z)-1 (4.0×10–3 mol dm–3, 500 mL) containing TEA (0.10 mol dm–3), placed in a Pyrex vessel, was irradiated for 7 h at wavelengths longer than 280 nm from a 400 W high-pressure Hg lamp at rt (internal irradiation, Pyrex glass filter). After the irradiation, the solution was concentrated to dryness in vacuo and the resulting residue was subjected to column chromatography over silica gel (230 mesh, Merck) eluting with acetone-hexane (2:1 v/v) or CHCl3-MeOH (15:1 v/v) containing 0.6 vol% TEA. For the purpose of isolating the photoproducts, preparative TLC plates (silica gel) were also used. Spectroscopic data of the isolated photoproducts: (4S,5S)-2a,d,g, (4R,5R)-2a,d,e,g, and (E)-1a,d are as follows. Any attempts to isolate analytical-grade (4S,5S)-2b,c,e,f and (4R,5R)-2b,c,f diastereomers were not fruitful and, additionally, we did not attempt to isolate the (E)-isomers other than (E)-1a and (E)-1d.
On the other hand, to evaluate the composition of the (Z)-1-derived 4,5-dihydrooxazole diastereomers, nitrogen-saturated MeOH and 1,2-dichloroethane solutions of (Z)-1 (4.0×10–3 mol dm–3, 10 mL) containing TEA (0.10 mol dm–3), placed in Pyrex test tubes, were irradiated in parallel for 5 h at wavelengths longer than 280 nm from a 400 W high-pressure Hg lamp set in a Pyrex cooling jacket (external irradiation). Parallel irradiation of the solutions was carried out at rt on a merry-go-round-type irradiation equipment immersed into a water bath (RIKO model RH400-10W). After the irradiation, the solutions were concentrated to dryness in vacuo and the resulting residues were dissolved in CDCl3 and subjected to 1H NMR spectral analyses.
(4S,5S)-4-(2-Aminocarbonylpyrrolidin-1-ylcarbonyl)-5-(1-naphthyl)-2-phenyl-4,5-dihydrooxazole [(4S,5S)-2a]: 1H NMR (600 MHz, CDCl3) δ = 1.49–1.58 (2H, m), 1.78–1.84 (1H, m), 2.11–2.17 (1H, m), 3.33–3.37 (1H, m), 3.59–3.64 (1H, m), 4.00 (1H, s), 4.28–4.31 (1H, m), 4.99 (1H, s), 5.70 (1H, d, J = 10.3 Hz), 6.64 (1H, d, J = 10.3 Hz), 7.48–7.61 (6H, m), 7.83 (1H, d, J = 7.4 Hz), 7.84 (1H, d, J = 8.0 Hz), 7.87 (1H ,d, J = 7.4 Hz), 7.92 (1H, d, J = 8.0 Hz), 8.15 (2H, d, J = 7.4 Hz); 13C NMR (150 MHz, CDCl3) δ = 24.7, 26.3, 47.8, 59.4, 70.9, 81.3, 121.7, 124.8, 125.6, 126.1, 126.5, 127.0, 128.5 (2C), 128.6, 128.7 (3C), 129.4, 131.3, 132.2, 133.4, 167.0, 168.1, 171.8. ESI-TOF-MS m/z calcd for C25H23N3O3: 414.1818 [M + H]+. Found: 414.1797.
(4R,5R)-4-(2-Aminocarbonylpyrrolidin-1-ylcarbonyl)-5-(1-naphthyl)-2-phenyl-4,5-dihydrooxazole [(4R,5R)-2a]: 1H NMR (600 MHz, CDCl3) δ = 0.46–0.56 (1H, m), 0.83–0.93 (1H, m), 1.08–1.16 (1H, m), 1.86–1.97 (1H, m), 3.16–3.22 (1H, m), 3.30–3.38 (1H, m), 4.82 (1H, s), 5.61 (1H, d, J = 10.3 Hz), 6.61 (1H, s), 6.73 (1H, d, J = 10.3 Hz), 7.48–7.61 (6H, m), 7.77 (1H, d, J = 7.4 Hz), 7.85 (1H, d, J = 8.0 Hz), 7.91 (1H ,d, J = 7.4 Hz), 7.97 (1H, d, J = 8.0 Hz), 8.13 (2H, d, J = 7.4 Hz). ESI-TOF-MS m/z calcd for C25H23N3O3: 414.1818 [M + H]+. Found: 414.1845.
(4S,5S)-4-(2-Anilinocarbonylpyrrolidin-1-ylcarbonyl)-5-(1-naphthyl)-2-phenyl-4,5-dihydrooxazole [(4S,5S)-2d]: 1H NMR (600 MHz, CDCl3) δ = 1.44–1.52 (1H, m), 1.77–1.82 (2H, m), 2.26–2.30 (1H, m), 3.23–3.27 (1H, m), 3.52–3.57 (1H, m), 4.40–4.41 (1H, m), 5.65 (1H, d, J = 10.3 Hz), 6.63 (1H, d, J = 10.3 Hz), 6.86 (1H, dd, J = 7.4, 7.4 Hz), 7.07–7.11 (3H, m), 7.19 (1H, d, J = 8.0 Hz), 7.26 (2H, dd, J = 6.8, 8.0 Hz), 7.38 (1H, dd, J = 7.4, 8.0 Hz), 7.47 (1H, d, J = 7.4 Hz), 7.49 (2H, dd, J = 7.4, 8.0 Hz), 7.57 (2H, d, J = 8.0 Hz), 7.66 (1H, d, J = 7.4 Hz), 7.76 (1H, d, J = 8.0 Hz), 8.16 (2H, d, J = 6.8 Hz), 8.69 (1H, s); 13C NMR (150 MHz, CDCl3) δ = 25.0, 25.4, 48.0, 60.3, 70.8, 81.0, 120.0 (2C), 121.1, 123.6, 123.7, 125.2, 126.0, 126.9, 128.3 (2C), 128.5 (2C), 128.6 (2C), 128.7, 128.8, 129.1, 129.5, 130.1, 132.1, 133.0, 138.1, 166.8, 167.0, 169.0. ESI-TOF-MS m/z calcd for C31H27N3O3: 490.2131 [M + H]+. Found: 490.2139.
(4R,5R)-4-(2-Anilinocarbonylpyrrolidin-1-ylcarbonyl)-5-(1-naphthyl)-2-phenyl-4,5-dihydrooxazole [(4R,5R)-2d]: 1H NMR (600 MHz, CDCl3) δ = 1.06–1.11 (1H, m), 1.62–1.68 (1H, m), 1.95–2.01 (2H, m), 3.09–3.12 (1H, m), 3.34–3.38 (1H, m), 3.63–3.64 (1H, m), 5.55 (1H, d, J = 10.3 Hz), 6.64 (1H, d, J = 10.3 Hz), 6.97 (1H, dd, J = 7.4, 7.4 Hz), 7.16 (2H, dd, J = 7.4, 8.0 Hz), 7.34, (2H, d, J = 8.0 Hz), 7.43–7.48 (4H, m), 7.52–7.60 (2H, m), 7.78 (2H, dd, J = 7.4, 8.0 Hz), 7.85 (1H, d, J = 8.0 Hz), 7.89 (1H, d, J = 8.0 Hz), 8.12 (2H, d, J = 7.4 Hz), 9.20 (1H, s); 13C NMR (150 MHz, CDCl3) δ = 23.8, 25.2, 46.8, 60.6, 61.6, 71.9, 80.4, 119.8 (2C), 121.6, 123.6, 124.7, 125.3, 125.6, 126.6, 126.9, 128.3 (2C), 128.4 (2C), 128.6 (2C), 129.1, 129.5, 130.5, 131.9, 133.0, 137.8, 166.7, 167.9, 168.8. ESI-TOF-MS m/z calcd for C31H27N3O3: 490.2131 [M + H]+. Found: 490.2116.
(4R,5R)-4-[2-(o-tert-Butylphenylaminocarbonyl)pyrrolidin-1-ylcarbonyl]-5-(1-naphthyl)-2-phenyl-4,5-dihydrooxazole [(4R,5R)-2e]: 1H NMR (600 MHz, CDCl3) δ = 0.39–0.45 (1H, m), 1.08–1.13 (1H, m), 1.29 (9H, s), 1.61–1.69 (1H, m), 2.03–2.07 (1H, m), 3.15–3.20 (1H, m), 3.40–3.44 (1H, m), 3.79 (1H, br d, J = 7.6 Hz), 5.63 (1H, d, J = 10.3 Hz), 6.71 (1H, d, J = 10.3 Hz), 7.08–7.12 (2H, m), 7.14–7.15 (1H, m), 7.30–7.32 (1H, m), 7.47 (2H, dd, J = 7.6, 7.6 Hz), 7.50 (1H, dd, J = 7.9, 7.9 Hz), 7.52 (1H, dd, J = 7.9, 7.9 Hz), 7.55 (1H, dd, J = 7.9, 7.9 Hz), 7.58 (1H, dd, J = 7.6, 7.6 Hz), 7.83 (2H, br d, J = 7.9 Hz), 7.88 (1H, d, J = 7.9 Hz), 7.96 (1H, d, J = 8.6 Hz), 8.11 (2H, d, J = 7.6 Hz), 8.49 (1H, s); 13C NMR (150 MHz, CDCl3) δ = 24.0, 25.7, 30.5 (3C), 34.5, 46.9, 60.8, 72.0, 80.7, 121.7, 125.0, 125.5, 125.7, 126.3, 126.3, 126.3, 126.4, 127.2, 128.4 (2C), 128.7 (2C), 128.9, 129.3, 129.3, 129.8, 130.6, 132.0, 133.2, 134.8, 144.1, 166.9, 168.6, 168.9. ESI-TOF-MS m/z calcd for C35H35N3O3: 546.2757 [M + H]+. Found: 546.2788.
(4S,5S)-4-(2-Morpholinocarbonylpyrrolidin-1-ylcarbonyl)-5-(1-naphthyl)-2-phenyl-4,5-dihydro-oxazole [(4S,5S)-2g]: 1H NMR (600 MHz, CDCl3) δ = 1.56–1.60 (1H, m), 1.62–1.68 (2H, m), 1.91–1.95 (1H, m), 3.09–3.13 (1H, m), 3.17–3.21 (1H, m), 3.24–3.29 (1H, m), 3.33–3.41 (3H, m), 3.43–3.49 (2H, m), 3.59–3.77 (2H, m), 4.32 (1H, dd, J = 4.2, 7.9 Hz), 5.66 (1H, d, J = 10.3 Hz), 6.63 (1H, d, J = 10.3 Hz), 7.44 (1H, dd, J = 7.6, 7.6 Hz), 7.48 (2H, dd, J = 7.6, 7.6 Hz), 7.52 (1H, dd, J = 7.6, 7.6 Hz), 7.54 (1H, dd, J = 7.6, 7.6 Hz), 7.57 (1H, dd, J = 7.6, 7.6 Hz), 7.74 (1H, d, J = 7.6 Hz), 7.81 (1H, d, J = 7.6 Hz), 7.84 (1H, d, J = 7.6 Hz), 7.90 (1H, d, J = 7.6 Hz), 8.15 (2H, d, J = 7.6 Hz). 13C NMR (150 MHz, CDCl3) δ = 25.0, 28.5, 42.0, 46.2, 47.5, 54.5, 66.5, 66.6, 71.9, 81.2, 122.4, 123.9, 125.0, 125.6, 126.1, 127.2, 128.4 (2C), 128.6, 128.7 (2C), 128.9, 129.9, 131.5, 132.0, 133.5, 166.1, 166.3, 169.5. ESI-TOF-MS m/z calcd for C29H29N3O4: 484.2236 [M + H]+. Found: 484.2224.
(4R,5R)-4-(2-Morpholinocarbonylpyrrolidin-1-ylcarbonyl)-5-(1-naphthyl)-2-phenyl-4,5-dihydro-oxazole [(4R,5R)-2g]: 1H NMR (600 MHz, CDCl3) δ = 0.62–0.66 (1H, m), 0.94–0.98 (1H, m), 1.27–1.40 (1H, m), 1.63–1.69 (1H, m), 3.08–3.11 (1H, m,), 3.16–3.23 (1H, m), 3.39–3.52 (6H, m), 3.58–3.66 (2H, m), 3.78 (1H, dd, J = 4.0, 8.6 Hz), 5.57 (1H, d, J = 10.3 Hz), 6.67 (1H, d, J =10.3 Hz), 7.45 (2H, dd, J = 7.4, 7.4 Hz), 7.49 (1H, dd, J = 7.4, 7.4 Hz), 7.53 (2H, dd, J = 8.0, 8.0 Hz), 7.59 (1H, dd, J = 6.9, 8.0 Hz), 7.79 (1H, d, J = 6.9 Hz), 7.82 (1H, d, J = 8.0 Hz), 7.90 (1H, d, J = 8.0 Hz), 8.01 (1H, d, J = 8.0 Hz), 8.12 (2H, d, J = 7.4 Hz); 13C NMR (150 MHz, CDCl3) δ = 23.8, 28.2, 42.2, 46.0, 46.8, 55.2, 66.5, 66.7, 71.8, 77.2, 80.5, 121.9, 125.2, 125.5, 125.7, 126.4, 127.3, 128.3 (2C), 128.6, 128.8 (2C), 129.1, 130.1, 131.1, 131.8, 133.2, 166.6, 171.2. ESI-TOF-MS m/z calcd for C29H29N3O4: 484.2236 [M + H]+. Found: 484.2204.
(E)-1a: 1H NMR (600 MHz, CDCl3) δ = 1.08–1.19 (1H, m), 1.45–1.65 (2H, m), 2.00–2.19 (1H, m), 2.47–2.54 (1H, m), 3.64–3.70 (1H, m), 4.38 (1H, dd, J = 2.9, 8.2 Hz), 6.70 (1H, s), 7.23 (2H, dd, J = 7.6, 7.6 Hz), 7.39 (1H, dd, J = 7.6, 7.6 Hz), 7.46 (1H, d, J = 8.2 Hz), 7.50 (1H, dd, J = 8.2, 8.2 Hz), 7.55 (1H, dd, J = 8.2, 8.2 Hz), 7.71 (2H, d, J = 7.6 Hz), 7.75 (1H, d, J = 8.2 Hz), 8.02 (1H, d, J = 8.2 Hz), 11.00 (1H, s); 13C NMR (150 MHz, CDCl3) δ = 23.4, 29.6, 47.7, 60.7, 118.2, 123.4, 124.7, 125.7, 126.1, 126.7, 127.7 (2C), 128.2 (2C), 128.5, 128.6, 128.7, 129.6, 131.0, 131.5, 132.0, 133.2, 166.3, 167.1, 174.6.
(E)-1d: 1H NMR (600 MHz, CDCl3) δ = 1.14 (1H, br s), 1.53–1.74 (2H, m), 2.10–2.12 (1H, m), 2.60 (1H, br s), 3.79 (1H, br s), 4.52–4.53 (1H, m), 6.59 (2H, dd, J = 7.4, 7.4 Hz), 7.09 (1H, d, J = 6.8 Hz), 7.16 (1H, dd, J = 6.8, 7.4 Hz), 7.30 (1H, s), 7.32–7.36 (5H, m), 7.49 (1H, dd, J = 7.4, 7.4 Hz), 7.56 (1H, dd, J = 7.4, 7.4 Hz), 7.62 (2H, d, J = 7.4 Hz), 7.71 (1H, d, J = 8.0 Hz), 7.92 (2H, d, J = 7.4 Hz), 8.04 (1H, d, J = 8.5 Hz), 9.34, (1H, s), 11.04 (1H, s); 13C NMR (150 MHz, CDCl3) δ = 23.4, 29.9, 47.9, 61.7, 118.1, 120.4 (2C), 123.3, 123.9, 124.3, 125.5, 126.0, 126.6, 127.2 (3C), 128.1 (2C), 128.6 (4C), 129.2, 130.8, 131.1, 131.7, 133.1, 138.6, 166.33, 167.6, 169.7.
ACKNOWLEDGMENTS
This research was partially supported by a “Scientific Frontier Research Project” from the Ministry of Education, Sports, Culture, Science and Technology, Japan.
References
1. a) S. R. L. Everitt and Y. Inoue, ‘Organic Molecular Photochemistry,’ ed. by V. Ramamurthy and K. S. Schanze, Marcel Dekker, New York, 1999, pp. 71–130; b) Y. Inoue, ‘Chiral Photochemistry,’ ed. by Y. Inoue and V. Ramamurthy, Marcel Dekker, New York, 2004, pp. 129–177; c) N. Hoffmann and J.-P. Pete, ‘Chiral Photochemistry,’ ed. by Y. Inoue and V. Ramamurthy, Marcel Dekker, New York, 2004, pp. 179–233; d) A. Bauer, F. Westkamper, S. Grimme, and T. Bach, Nature, 2005, 436, 1139. CrossRef
2. a) K. Maekawa, K. Kubo, T. Igarashi, and T. Sakurai, Heterocycles, 2002, 57, 1591; CrossRef b) K. Maekawa, K. Kubo, T. Igarashi, and T. Sakurai, Tetrahedron, 2004, 60, 1183; CrossRef c) K. Maekawa, K. Kubo, T. Igarashi, and T. Sakurai, Tetrahedron, 2005, 61, 11211. CrossRef
3. a) Y. Sasaki, K. Maekawa, H. Watanabe, T. Matsumoto, K. Kubo, T. Igarashi, and T. Sakurai, Tetrahedron Lett., 2007, 48, 4765; CrossRef b) H. Watanabe, K. Maekawa, T. Igarashi, and T. Sakurai, Heterocycles, 2007, 74, 149. CrossRef
4. a) K. Maekawa, T. Sasaki, K. Kubo, T. Igarashi, and T. Sakurai, Tetrahedron Lett., 2004, 45, 3663; CrossRef b) K. Maekawa, N. Hishikawa, K. Kubo, T. Igarashi, and T. Sakurai, Tetrahedron, 2007, 63, 11267. CrossRef
5. Y. Sato, A. Yoshida, T. Igarashi, and T. Sakurai, Heterocycles, 2010, 81, 997. CrossRef
6. J. A. Riddick, W. B. Bunger, and T. K. Sakano, ‘Organic Solvents,’ 4th ed., Wiley, Chichester, 1986.