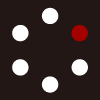
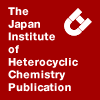
HETEROCYCLES
An International Journal for Reviews and Communications in Heterocyclic ChemistryWeb Edition ISSN: 1881-0942
Published online by The Japan Institute of Heterocyclic Chemistry
e-Journal
Full Text HTML
Received, 11th June, 2010, Accepted, 8th July, 2010, Published online, 9th July, 2010.
DOI: 10.3987/COM-10-S(E)55
■ Boromycin Derivatives: Synthesis and Antimalarial Activity in vitro and in vivo
Ayumi Tsutsui, Yujiro Furuya, Tomoyasu Hirose, Riyon Kim, Rokurou Masuma, Atsuko Matsumoto, Yoko Takahashi, Aki Ishiyama, Miyuki Namatame, Kazuhiko Otoguro, Satoshi Ōmura,* and Toshiaki Sunazuka*
Kitasato University, 5-9-1 Shirokane, Minato-ku, Tokyo 108-8642, Japan
Abstract
Derivatives of boromycin, which has significant antimalarial properties both in vitro and in vivo, were examined for similar the efficacy against both drug-resistance strain and drug-sensitive strain of malaria and as a means to elucidate structure-activity relationships. More potent in vitro compounds tended to lose their properties in vivo when administered orally. Some novel 16-O-acyl derivatives were found to be more potent and selective antimalarial compounds compared to boromycin. Furthermore, alkyne-bearing 16-O-alkylacyl compounds retained reasonably good efficacy in vivo when given per os.Malaria is a serious infectious disease worldwide, especially in sub-Saharan Africa and Asia. Malaria, caused by Plasmodium species, occurs in more than 90 countries, with more than 243 million cases in 2008 causing an estimated 863,000.1 Resistance to commonly-used antimalarial drugs develops
quickly and spreads rapidly, and has rendered most available antimalarials of very restricted use, apart from the newly-introduced artemisinin compounds which currently maintain their effectiveness extensively. New drugs, particularly those with novel modes of action, are urgently required. In addition, due to the impoverished conditions in which malarial prevails, drugs that are easily and cheaply administered are of greatest use. Oral medicines are therefore of significant interest. In our institute, we have a long-standing program of activity focusing on the search for natural products and their derivatives, which may be useful to combat neglected tropical diseases.2,3 Recently, we have further investigated, boromycin as a potential antimalarial agent. Boromycin was previously reported to show diverse bioactivity such as anti-Gram positive bacterial, anticoccidial and anti-HIV properties.4,5 It was independently isolated from a culture broth of Streptomyces sp. OH-1034 and found to be a G2 checkpoint inhibitor in 2004.6 In an early study, Hütter and co-workers demonstrated the antimalarial property of boromycin against Plasmodium berghei. (ED50 15 mg/kg p.o. oder s.c.).7 In this report, we describe the synthesis, antimalarial activitiy and cytotoxicity of boromycin and several derivatives.
We re-evaluated boromycin against the Plasmodium falciparum strain K1 (chloroquine-resistant) and FCR3 (drug-sensitive) strain in vitro, in comparison with clinically-used antimalarial drugs (Artemisinin and Chloroquine), and found inhibitory activities with IC50 values of 35 ng/mL and 36 ng/mL against the K1 and FCR3 strains, respectively (Table 1). Furthermore, in vivo antimalarial activity of boromycin per os (p.o.) at 10 mg/kg was determined using a rodent malaria-derived Plasmodium berghei strain according to the 4-days suppressive test of Peters and co-workers.5,8,9 Parasite growth was inhibited by 97.9%. The cytotoxicity of boromycin was measured by colorimetric MTT assay5,10,11 with human MRC-5 cells, producing an IC50 value of 450 ng/mL. We devised Selectivity
Indexes (cytotoxicity [IC50 for the MRC-5 cell]/antimalarial activity [IC50 for the K1 strain or the FCR3 strain], to allow better comparisons (Table 1).
Our results, indicated that the selectivity between cytotoxicity and antimalarial activity for boromycin is relatively low and that the compound is thus deemed unsuitable for development as a human medicine. We subsequently produced a variety of boromycin derivatives in order to test their efficacy and suitability for human use, as well as to help gain a better understanding of structure-activity relationships.
One particular derivative, devalinylboromycin (BRM-01), which is easy to prepare by hydrolysis of boromycin according to the protocol of previous report,12 showed extremely good in vitro efficacy against both K1 and FCR3 strains and had lower cytotoxicity than the parent compound (Table 1). Unfortunately, when tested in vivo BRM-01 showed no parasite growth inhibition at 10 mg/kg when administered orally. Consequently, to elucidate the antimalarial property and structure-activity relationships, a variety of derivatives were synthesized from the highly-promising devalinylboromycin (BRM-01). Modifications of the sterically less hindered alcohol (16-OH) 16-hydroxy group were carried out to prepare acetal, dithiocarbonate and ketone derivatives. Isovaleric acid was selected to prepare an acyl derivative (BRM-02), because isovaleryl moiety can imitate the valinyl function. Actually, isovalerylation of 16-OH hardly proceeded under reflux conditions with EDCI and DMAP (10% yield) due to sterically hindrance of the substrate. MOMCl was reacted with 16-OH of BRM-01 to give the acetal derivative in moderate isolated yield (45%, BRM-03). Other derivatives, dithiocarbonate (BRM-04) and ketone (BRM-05), were obtained under standard conditions in 76% and 84% yields, respectively. Subsequently, conversion of the cis-substituted olefin at C12-13 within BRM-01 produced the epoxide (BRM-06), diol (BRM-07) and saturated derivative (BRM-08). mCPBA together with NaHCO3 were added to a solution of BRM-01 in CH2Cl2 to give 12, 13-epoxide BRM-06, in 52% yield, as a nearly 1:1 diasteromeric mixture. Utilization of AD-mix-β to prepare the 12, 13-diol derivative resulted in relatively good conversion yield (67% without decomposed substrate) due to the gentle reactivity compared with standard dihydroxylation conditions, such as OsO4/NMO. Additionally, BRM-01 was quickly reduced to a saturated carbon skeleton by hydrogenation of the olefin with Pd/C under a hydrogen atmosphere to give 80% yield. The IC50 values of each compound were then determined, as summarized in Table 2. In comparison with BRM-01, the activity of BRM-02, which contains a 16-O-vareloyl residue, was halved, whereas the other compounds possessed relatively little antimalarial activity.
Significantly, the importance of the 12,13-olefin of BRM-01 was highlighted from the IC50 value of
BRM-06, BRM-07 and BRM-08. These results intimated that 16-O-acyl derivatives (such as BRM-02) would be good lead antimalarial compounds. Subsequently, several 16-O-alkylacyl derivatives of BRM-01 were synthesized to try and obtain more potent antimalarial compounds. The 16-O-acetyl BRM-09 was synthesized with Ac2O in pyridine (22% yield). The other esters, BRM-10 to BRM-20, were synthesized via EDCI or Yamaguchi esterification reaction13,14 in 35~100% yields. The IC50 values of these compounds were subsequently evaluated, as shown in Table 3. Generally, the 16-O-acyl compounds exhibited some activity against both K1 and FCR3 strains. Among them, BRM-11, 13, 14 and 20 demonstrated relatively good impact, with IC50 values of 39 ng/mL, 37 ng/mL, 17 ng/mL and 20 ng/mL against the P. falciparum K1 strain, respectively. In addition, BRM-14, surprisingly, proved twice more potent against the drug-resistant strain than against drug-sensitive parasites. Subsequently, in vivo tests were carried out using BRM-11, 14 and 20 in the P. berghei mouse model. BRM-11 and 14, at 10 mg/kg were administered intraperitoneally and both curtailed parasite growth by more than 70% (BRM-11; 77.9%, BRM-14; 72.3%). However, the inhibitory activity of BRM-20 was low (59.0%). Consequently, the former two compounds (BRM-11 and 14) were examined using oral at 10 mg/kg. BRM-14 showed an inhibition 46.2% while BRM-11 was rendered almost ineffective at 9.6% inhibition. The cytotoxicity of BRM-14 against MRC-5 cells indicated an IC50 value of 3.95 µg/mL creating high Selectivity Indexes, with values of 232.4 for the MRC-5 cell/K1 strain and 112.9 for MRC-5 cell/FCR3 strain, respectively, which were superior to boromycin and most other compounds but well bellow that of BRM-01. As the alkyne-bearing analogue (BRM-14) expressed moderate efficacy in vivo and showed relatively good Selectivity Index values in vitro, similar compounds, BRM-13 and 15, were also examined in vivo, administered i.p. and p.o. at 10 mg/kg, to identify the effect of the terminal alkynes. Administered intraperitoneally, BRM-13 and 15 exhibited good parasite growth inhibition, with values of 89.5% and 93.1%, respectivily. However, the impact of BRM-13 administered orally resulted in parasite growth inhibition of a moderate 59.2%, while BRM-15 administered p.o. produced a very weak result (24.7% inhibition).
In summary, we discovered boromycin, despite a relatively poor selectivity as an antimalarial in vitro, exhibited good impact against P. berghei in vivo. Conversely, a derivative, devalinilboromycin, possessed extremely promising antimalarial impact in vitro but the effect did not materialize in vivo when the compound was given orally. Moreover, we discovered that several boromycin derivatives that exhibited promising antimalarial properties in vitro lost this activity in vivo when administered orally. We hydpothesized that the 12,13-olefin of boromycin appeared to play a major role in the antimalarial activity. Subsequently, alkyne-bearing 16-O-alkylacyl derivatives (BRM-13 and 14) showed acceptable inhibitory activity against both chloroquine-resistant and drug-sensitive strains of P. falciparum in vitro, and were recognized as being good lead compounds, especially as they maintained a degree of
antimalarial activity when given by mouth in vivo. Further studies of these analogues are in progress.
ACKNOWLEDGMENTS
This study was supported, in part, by funds from Quality Assurance Framework of Higher Education from the Ministry of Education, Culture, Sports, Science and Technology (MEXT) in Japan, the All Kitasato Project Study (AKPS) and the 21st century COE program. We are grateful to Ms. Hitomi Sekiguchi and Ms. Aki Nishihara for their technical assistance and to Ms. Akiko Nakagawa, Dr. Kenichiro Nagai and Ms. Noriko Sato, School of Pharmacy, Kitasato University for measurements of mass and NMR spectra.
References
1. World Health Organization. World malaria Report 2009. Available at http://www.who.int/malaria/world_malaria_report_2009/en/index.html.
2. Y. Kojima, T. Sunazuka, K. Nagai, K. Hirose, M. Namatame, A. Ishiyama, K. Otoguro, and S. Ōmura, J. Antibiot., 2009, 62, 681. CrossRef
3. A. Ishiyama, K. Otoguro, M. Namatame, A. Nishihara, T. Furusawa, Y. Takahashi, R. Masuma, K. Shiomi, and S. Ōmura, J. Antibiot., 2008, 61, 254. CrossRef
4. J. Kohno, T. Kawahata, T. Otake, M. Morimoto, H. Mori, N. Uebe, M. Nishio, A. Kinumaki, A. Komatsubara, and K. Kawashima, Biosci. Biotech. Biochem., 1996, 60, 1036. CrossRef
5. K. Otoguro, A. Kohana, C. Manabe, A. Ishiyama, H. Ui, K. Shiomi, H. Yamada, and S. Ōmura, J. Antibiot., 2001, 54, 658.
6. M. Arai, Y. Koizumi, H. Sato, T. Kawabe, M. Suganuma, H. Kobayashi, H. Tomoda, and S. Ōmura, J. Antibiot., 2004, 57, 662.
7. R. Hütter, W. Keller-Schierlein, F. Knüsel, V. Prelog, G. C. Rodgers jr., P. Suter, G. Vogel, W. Voser, and H. Zähner, Helv. Chim. Acta, 1967, 50, 1533. CrossRef
8. K. Otoguro, A. Ishiyama, H. Ui, M. Kobayashi, C. Manabe, G. Yan, Y. Yakahashi, H. Tanaka, H. Yamada, and S. Ōmura, J. Antibiot., 2002, 55, 832.
9. W. Peters, H. Portus, and B. L. Robinson, Ann. Trop. Med. Parasitol, 1975, 69, 155.
10. T. Mossman, J. Immunol, Methods, 1983, 65, 55.
11. K. Otoguro, K. Komiyama, S. Ōmura, and C. A. Tyson, ATLA, 1991, 19, 352.
12. M. A. Avery, and J. D. White, Tetrahedron Lett., 1981, 22, 3123. CrossRef
13. J. Inanaga, K. Hirata, H. Saeki, T. Katsuki, and M. Yamaguchi, Bull. Chem. Soc. Jpn., 1979, 52, 1989. CrossRef
14. Experimental data of BRM-14; 1H NMR (600MHz, CDCl3) δ 6.01 (s, 1H), 5,49 (d, 1H, J = 11.3, 10.8 Hz), 5.39 (dd, 1H, J = 12.7, 6.15 Hz), 5.26 (dd, 1H, J= 12.7, 3.5), 5.18 (dt, 1H, J = 11.3, 12.2), 5.05 (d, 1H, J=7.4), 4.98 (d, 1H, J = 3.9), 4.80 (dd, 1H, J = 3.9, 6.7 Hz), 4.43 (s, 1H), 4.42 (s, 1H), 4.09 (m, 2H), 3.87 (dd, 1H, J = 1.7, 11.7 Hz), 3.71 (dd, 1H, J = 2.6, 12.1, Hz), 3.02 (dd, 1H, J = 7.6, 10.4, Hz), 2.60 (t, 1H, J = 13.0 Hz), 2.57 (m, 2H), 2.43 (m, 2H), 2.36 (m, 2H), 1.99 (m, 5H), 1.87 (m, 2H), 1.81 (m, 3H), 1.59 (m, 5H), 1.48 (m, 2H), 1.35 (d, 3H, J = 6.15 Hz), 1.34 (m, 4H), 1.18 (m, 4H), 1.10 (d, 3H, J = 6.7 Hz), 0.99 (d, 3H, J = 6.6 Hz), 0.96 (d, 3H, J = 6.7 Hz), 0.95 (s, 3H, 8-CH3), 0.71 (s, 6H, 8-CH3), 0.70 (s, 3H, 8’-CH3), 0.61 (s, 3H, 8’-CH3); 13C NMR (150MHz, CDCl3) δ 173.4 (C-17), 170.8 (C-1), 170.6 (C-1’), 135.2 (C-12), 122.3 (C-13), 106.0 (C-3’), 105.4 (C-3), 84.6 (C-9), 83.4 (C-21), 79.6 (C-15’), 79.5 (C-7’), 78.7 (C-2), 77.4 (C-2’), 77.2 (C-16’), 76.0 (C-13’), 75.8 (C-9’), 74.1 (C-15), 73.7 (C-16), 73.2 (C-7), 69.2 (C-22), 39.3 (C-8), 39.0 (C-8’), 36.8 (C-14’), 35.8 (C-12’), 33.4 (C-4), 33.0 (C-4’), 32.4 (C-18), 30.0 (C-10), 28.6 (C-5’), 28.2 (C-5), 27.8 (C-14), 27.2 (C-10’), 26.2 (C-11), 25.4 (C-6’), 25.1 (C-6), 23.5 (8-CH3), 23.0 (C-19), 20.9 (8’-CH3), 20.3 (C-11’), 20.0 (8-CH3), 19.5 (C-20), 18.8 (16’-CH3), 17.5 (C-17’), 16.5 (4’-CH3), 14.2 (16-CH3), 12.6 (8’-CH3); ESI-MS: m/z calcd for C46H70BO15Na2 [(M+2Na)+]: 919.46031. Found: m/z 919.46056.