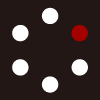
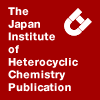
HETEROCYCLES
An International Journal for Reviews and Communications in Heterocyclic ChemistryWeb Edition ISSN: 1881-0942
Published online by The Japan Institute of Heterocyclic Chemistry
e-Journal
Full Text HTML
Received, 11th May, 2010, Accepted, 10th June, 2010, Published online, 11th June, 2010.
DOI: 10.3987/COM-10-S(E)27
■ Total Synthesis of (+)- and (-)-Galanthamine
Tomoaki Kato, Hiroki Tanimoto, Hisako Yamada, and Noritaka Chida*
Department of Applied Chemistry, Faculty of Science and Technology, Keio University, Hiyoshi, Kohoku-ku Yokohama 223-8522, Japan
Abstract
The stereoselective total synthesis of (+)-galanthamine [(+)-1], an antipode of the natural product, and (–)-galanthamine [(–)-1] starting from D-glucose is described. The cyclohexene unit in (+)-1 was prepared in an optically active form from D-glucose using Ferrier’s carbocyclization reaction, and the benzylic quaternary carbon was stereoselectively generated via chirality transfer by Johnson- or Eschenmoser-Claisen rearrangement. The dibenzofuran skeleton was effectively constructed by the bromonium ion-mediated intramolecular dealkylating etherification. After the introduction of a carbon-carbon double bond, the Pictet-Spengler type cyclization, followed by reduction of an amide function afforded (+)-1. Starting from D-glucose, (–)-galanthamine [(–)-1] was also totally synthesized.INTRODUCTION
(–)-Galanthamine [(–)-1], an alkaloid isolated from some species of the Amaryllidaceae family,1,2 has been reported to be a centrally acting acetylcholinesterase inhibitor3 and an allosteric modulator of the neuronal nicotinic receptor for acetylcholine.4 On the basis of these biological activities, (–)-galanthamine was developed as a medicine for Alzheimer’s disease and has been clinically used in Europe and the USA.2c,3b Its important and significant biological activity as well as its interesting structure have naturally received considerable attention from the synthetic community, and several synthetic approaches to 1 have been reported to date.2,5,6 A number of successful total syntheses of galanthamine, employing the biomimetic oxidative bisphenol coupling,2,5a,6a intramolecular Heck reaction,2,5b,6b,6d,6e,6f semipinacol rearrangement,5c double Michael-Claisen reaction cascade of acetone derivatives with acrylates,5d and intramolecular para-alkylation of a phenol derivative,5e for construction of the characteristic tetracyclic skeleton possessing a benzylic quaternary carbon, have appeared, however, reports of the chiral syntheses of 1 are rather limited.6 Due to the scarce supplies of galanthamine from natural sources7 and the necessity for preparing structural analogues for the development of more potent drugs, it is still important to establish a chiral and effective synthetic route to the alkaloid from readily available materials. In this paper, we report a stereoselective total synthesis of (+)-galanthamine [(+)-1] and its natural enantiomer (–)-1 starting from D-glucose.8
RESULTS AND DISCUSSION
Our earlier reports of the total synthesis of (+)-vittatine and (+)-haemanthamine, Amaryllidaceae alkaloids possessing the hexahydroindole skeleton with a 1,3-dioxolane ring at m- and p-positions in the phenyl group as the core structure, starting from D-glucose revealed that the methodology involving Claisen rearrangement on the chiral cyclohexenol derived from carbohydrates is effective for the stereoselective generation of quaternary carbons.9 Taking the successful synthesis of vittatine and haemanthamine into accounts, our retrosynthetic analysis for (+)-galanthamine (+)-1 suggested that the tetracyclic lactam 2, which had been utilized in the synthesis of racemic galanthamine by Guillou,5b would be a suitable intermediate for the total synthesis (Figure 1). The dibenzofuran skeleton in 2 was expected to be constructed by the bromonium ion-mediated intramolecular dealkylating etherification10 of cyclohexene 3 possessing phenolic ether functions at o- and m-positions, whose crucial quaternary carbon preparation was planned using the Claisen rearrangement9,11 of cyclohexenol derivative 4. The cyclohexenol 4, in turn, was envisioned to be synthesized by the coupling reaction of an aryl metal species with cyclohexenone (+)-5, which is a known compound12 and has been prepared in the optically pure form starting from D-glucose by way of the Ferrier’s carbocyclization.13 Since the enantiomeric cyclohexenone (–)-5 could also be synthesized from D-glucose,12 the synthetic way to (+)-galanthamine would be readily applicable to the synthesis of a natural enantiomer of galanthamine.
The treatment of (4R,6S)-6-benzyloxy-4-[(tert-butyldimethylsilyl)oxy]-2-cyclohexenone12 (+)-5, prepared from commercially available methyl 4,6-O-benzylidene-α-D-glucopyranoside 6 utilizing the catalytic Ferrier’s carbocyclization13c as the key transformation in a total of 8 steps with 38% overall yield, with 2,3-dimethoxyphenylmagnesium bromide at –78 °C gave 1,2-adducts 7 in 85% yield as a diastereomeric mixture (7a : 7b = 4 : 1) (Scheme 1). The configuration of C-1 in 7a was assigned by the observed NOE between H-6 and OH at C-1. When cyclohexenone (+)-5 was reacted with 2,3-dimethoxyphenyllithium in the presence of TMEDA, compound 7 was obtained in 60% yield with a reverse stereoselectivity (7a : 7b = 1 : 3).
The oxidation of a mixture of 7a and 7b (4 : 1) with pyridinium chlorochromate (PCC) in the presence of molecular sieves 4A (MS4A) afforded cyclohexenone 8 in 75% yield,14 which was reduced under the conditions of Luche15 at –78 °C to give cyclohexenol 4 and its C-1 epimer in 89 and 9% isolated yields, respectively (Scheme 2). The observed coupling constants in 4 (J1,6 = 7.8 Hz) and its C-1 epimer (J1,6 = 4.1 Hz) supported their assigned configurations. With cyclohexenol 4 possessing the desired stereochemistry in hand, the crucial Claisen rearrangement was next investigated. Johnson-Claisen rearrangement16 of 4 in triethyl orthoacetate in the presence of 2-nitrophenol9b,17 in a sealed tube at 140 °C for 52 h successfully afforded the rearranged product 3a in 80% yield. It is important to note that the Johnson-Claisen rearrangement of 4, possessing an o-methoxy substituent in the phenyl group, proceeded in a high yield when 2-nitrophenol was employed as the acid catalyst. It has been reported that the conventional Johnson-Claisen rearrangement (using propionic acid as the catalyst) of the simple cyclohexenol systems similar to 4 resulted in the very poor yields of the rearranged products, probably due to the acid sensitivity of the substrates and the steric congestion at the reaction center caused by the presence of the o-methoxy substituent in the phenyl group.10,11b,18 Indeed, the Johnson-Claisen rearrangements of 4 in the presence of propionic acid (10 ~ 130 mol% to 4) at 140 °C gave 3 in less than 40% yields, and the formation of a significant amount of unidentified by-products was observed. The acidity of 2-nitrophenol (pKa = 7.04) would be appropriate for the Johnson-Claisen rearrangement of 4; its weaker acidity than that of propionic acid (pKa = 4.62) suppressed the decomposition of the acid-labile substrate but could catalyze the formation of a ketene acetal. On the other hand, Eschenmoser-Claisen rearrangement19 of 4 (N,N-dimethylacetamide dimethyl acetal in o-xylene in a sealed tube at 150 °C) cleanly afforded 3b in an excellent yield (92%).
Next, we turned out attention to construct the dibenzofuran skeleton from 3a and 3b. The treatment of 3a with N-bromosuccinimide (NBS) in DMF induced the intramolecular dealkylating etherification via a bromonium ion intermediate10 3a’ to give bromo-dibenzofuran derivative 9 as a single product in 84% yield (Scheme 3). Similar reaction of 3b with NBS, however, afforded no dibenzofuran product and resulted in the formation of many unidentified products. Among them, γ-lactone 10 was isolated in 37% yield. It is known that the formation of a cyclic bromonium ion intermediate in the congested alkene systems is a reversible step, and the subsequent nucleophilic opening of the bromonium ion is a rate-determining one.10,20 The carbonyl group in amide 3b is more nucleophilic than the phenolic ether oxygen, thus providing lactone 10 via the epimeric cyclic bromonium ion intermediate 3b’. Hydrogenolysis of 9 in the presence of Pd on carbon and potassium carbonate in EtOH under atmospheric pressure of H2 caused the debromination as well as the deprotection of the O-benzyl group to provide 11. However, under these basic conditions, the de-O-benzylation process was found to be very sluggish, and resulted in the low yield of 11 (less than 40%). In contrast, under similar hydrogenolytic conditions without potassium carbonate, deprotection of the O-benzyl group smoothly proceeded, but the debromination process became slow. After some attempts, it was found that the two-steps in a one-pot reaction gave satisfactory results. Thus, deprotection of the O-benzyl group in 9 under neutral conditions (Pd on carbon, atmospheric pressure of H2 in EtOH) was first carried out. After completion of the de-O-benzylation (TLC monitoring), potassium carbonate was added to the reaction mixture and the hydrogenolysis of the C-Br bond was further continued in the same reaction vessel to give 11 in 85% yield. The structure of 11 was confirmed by NMR analyses of the derived benzoate 12; the observed large coupling constants (J5a,6ax, J6ax,7, J7,8ax and J8ax,9) revealed that the substituents at C-5a, C-7 and C-9 are all in the equatorial positions, and NOE experiments (correlations between H-5a and C-2’methylene, and H-9 and C-2’ methylene) clearly assigned the stereochemistry of the quaternary carbon at C-9a as R. Hydrogenolysis of 10 in the absence of potassium carbonate similarly removed the O-benzyl group, and under the reaction conditions, the O-TBS group was also detached to give bromo-diol 13 (25% yield from 3b). The IR spectrum of 13 suggested the presence of hydroxy and γ-lactone groups, and the 1H NMR analysis assigned the structure of 13 as depicted in Scheme 3.
To introduce the requisite carbon-carbon double bond, compound 11 was treated with (thiocarbonyl)diimidazole and DMAP in dichlorobenzene at 180 °C21 to afford 14 in 72% yield (Scheme 4). Hydrolysis of the ethyl ester function in 14, followed by condensation with methylamine under the conditions of Shioiri,22 provided amide 15 in 93% yield. The Pictet-Spengler type reaction of 15 with paraformaldehyde in the presence of TFA5b,5c generated the methano-bridge between the amide nitrogen and C-1, and induced the deprotection of the O-TBS group to give tetracyclic lactam 2, which is known as the intermediate for the synthesis of racemic galanthamine reported by the Guillou5b and Tu5c in 67% yield. However, the reproducibility of the reaction was found to be rather poor, and the yields of 2 sometimes dropped to 20-40%. Fortunately, it was found when the same reaction conditions were applied to allylic alcohol 16, compound 2 was obtained in better yields with good reproducibility. Removal of the O-TBS group in 15 afforded the known alcohol 16,5c whose reaction with paraformaldehyde and TFA provided 2 in 71% overall yield from 15. Finally, reduction of 2 with LiAlH4 cleanly afforded (+)-galanthamine [(+)-1] in 88% yield. The 1H and 13C NMR data of the synthetic 1 were totally identical with those of natural (–)-galanthamine and the [α]D value of the synthetic compound {[α]D24 +112 (c 0.50, EtOH): lit.,1c [α]D20 –118.8 (c 1.378, EtOH)} confirmed its unnatural absolute configuration. Thus, total synthesis of (+)-galanthamine starting from D-glucose has been accomplished. Starting from the enantiomeric cyclohexenone (–)-512 obtained from 6 in 12 step reactions, (–)-galanthamine [(–)-1] {[α]D23 –112 (c 0.19, EtOH)} was also totally synthesized via the same reaction sequence.
CONCLUSIONS
In summary, a new and non-biomimetic synthetic route to optically active galanthamine starting from D-glucose has been established. This synthesis, required 12 steps with a 13.6% overall yield from (+)-5 (20 steps, 5.2% overall yield from commercially available 6) for the synthesis of (+)-galanthamine, and 24 steps with 2.7% overall yield from 6 for the synthesis of (–)-galanthamine, demonstrated that the methodology involving the Claisen rearrangement on chiral cyclohexenol derived from D-glucose is effective for the chiral and stereoselective synthesis of galanthamine-type alkaloids. It was shown that the Eschenmoser-Claisen rearrangement is a powerful method for the construction of the sterically hindered quaternary carbon. The effectiveness of 2-nitrophenol as the acid catalyst for the Johnson-Claisen rearrangement is particularly noteworthy. It was also revealed that the intramolecular dealkylating etherification using NBS is a useful procedure for the construction of benzofuran skeletons that are commonly found in galanthamine-type as well as morphine-type biologically significant alkaloids.
EXPERIMENTAL
General. Melting points were determined on a Mitamura-Riken micro hot stage and were not corrected. Optical rotations were recorded using a sodium lamp (589 nm) with a JASCO DIP-370 instrument with 1 dm tube. Infrared (IR) spectra were measured with a JASCO FT/IR-200 spectrometer with a KBr cell. 1H NMR spectra were recorded at 300 MHz on a Varian MVX-300 spectrometer for solutions in CDCl3, unless otherwise noted. Chemical shifts are reported as δ values in ppm. Abbreviations used are: b (broad peak), s (singlet), d (doublet), t (triplet), q (quartet) and m (complex multiplet). 13C NMR spectra were recorded at 75 MHz on a Varian MVX-300 spectrometer for solutions in CDCl3, unless otherwise noted. Chemical shifts are reported as δ values in ppm. Mass spectra are measured by a JEOL GC Mate spectrometer with EI (70 eV) or FAB (glycerol matrix) mode. Organic extracts were dried over anhydrous Na2SO4 and concentrated below 40 °C under reduced pressure. Column chromatography was carried out with silica gel (Merck Kieselgel 60 F254, 230-400 mesh). Preparative TLC was performed with Merck PLC plate (Kieselgel 60 F254, 0.5 mm thickness).
(1R,4R,6S)-6-Benzyloxy-4-[(tert-butyldimethylsilyl)oxy]-1-(2,3-dimethoxyphenyl)-2-cyclohexen-1-ol (7a) and Its (1S)-Epimer (7b). Preparation with Grignard reagent: To a solution of (+)-5 (42.0 mg, 0.126 mmol) in THF (2.1 mL) under argon at –78 ºC was added 2,3-dimethoxyphenylmagnesium bromide (0.5 M solution in THF, 0.80 mL, 0.40 mmol). After being stirred at –78 ºC for 4 h, the reaction mixture was quenched with saturated aqueous NH4Cl solution at –78 ºC and the products were extracted with EtOAc. The extract was washed with saturated aqueous NaHCO3 solution and dried. The organic solvent was concentrated to give a residue, which was purified by column chromatography (3 g, toluene then EtOAc/toluene = 1/100 as an eluent) to afford 7a (40.3 mg, 68%) as a colorless oil: [α]D28 +3.4 (c 1.54, CHCl3); IR (neat) νmax = 3440, 2950, 2930, 2880, 2860, 1470, 1260, 1070 cm-1; 1H NMR δ 7.34–7.22 (5H, m), 7.02 (1H, dd, J = 8.1 and 7.8 Hz), 6.94 (1H, dd, J = 7.8 and 1.8 Hz), 6.89 (1H, dd, J = 8.1 and 1.8 Hz), 6.62 (1H, s), 5.81 (1H, ddd, J = 10.1, 1.7 and 0.9 Hz), 5.61 (1H, dd, J = 10.1 and 1.8 Hz), 4.67 and 4.62 (each 1H, 2d, J = 12.3 Hz), 4.37 (1H, dddd, J = 9.1, 6.3, 1.8 and 1.7 Hz), 3.85 and 3.84 (each 3H, 2s), 3.74 (1H, dd, J = 12.6 and 3.2 Hz), 2.09 (1H, dddd, J = 12.6, 6.3, 3.2 and 0.9 Hz), 1.59 (1H, ddd, J = 12.6, 12.6 and 9.1 Hz), 0.90 (9H, s), 0.09 and 0.06 (each 3H, 2s); 13C NMR δ 152.6, 148.9, 138.5, 132.4, 132.3, 131.9, 128.2, 128.0, 127.5, 123.1, 122.7, 112.4, 82.4, 79.1, 71.7, 67.8, 61.5, 55.9, 34.6, 25.9, 18.2, –4.57, –4.64; HRMS (FAB) m/z calcd for C27H39O5Si (M+H)+ 471.2567, found 471.2571. Anal. Calcd for C27H38O5Si: C, 68.90; H, 8.14%. Found: C, 68.60; H, 8.04%. Further elution gave 7b (10.1 mg, 17%) as a colorless oil: [α]D20 +111 (c 1.04, CHCl3); IR (neat) νmax = 3520, 2950, 2930, 2860, 1470, 1270, 1090 cm-1; 1H NMR δ 7.16–7.24 (4H, m), 7.07 (1H, dd, J = 8.0 and 8.0 Hz), 6.98–7.04 (2H, m), 6.90 (1H, dd, J = 8.0 and 1.5 Hz), 5.86 (1H, ddd, J = 10.0, 2.0 and 1.2 Hz), 5.66 (1H, dd, J = 10.0 and 2.2 Hz), 4.40 (1H, dddd, J = 10.1, 5.3, 2.2 and 2.0 Hz), 4.32 and 4.13 (each 1H, 2d, J = 11.7 Hz), 4.06 (1H, dd, J = 12.0 and 3.9 Hz), 3.92 (1H bs), 3.87 and 3.73 (each 3H, 2s), 2.11 (1H, dddd, J = 12.0, 5.3, 3.9 and 1.2 Hz), 1.98 (1H, ddd, J = 12.0, 12.0 and 10.1 Hz), 0.92 (9H, s), 0.11 and 0.10 (each 3H, 2s); 13C NMR δ 152.9, 146.4, 139.0, 138.0, 133.6, 130.9, 128.1, 127.6, 127.5, 123.4, 119.5, 111.8, 78.5, 72.5, 72.2, 68.2, 60.5, 55.8, 35.0, 25.8, 18.2, –4.68, –4.67; HRMS (FAB) m/z calcd for C27H39O5Si (M+H)+ 471.2567, found 471.2561. Anal. Calcd for C27H38O5Si: C, 68.90; H, 8.14%. Found: C, 68.62; H, 8.23%.
Preparation with aryllithium reagent: To a solution of 1,2-dimthoxybenzene (1.23 g, 8.90 mmol) in Et2O (8.6 mL) at 0 ºC under Ar were added TMEDA (1.34 mL, 8.90 mmol) and n-BuLi (1.52 M hexane solution, 5.86 mL, 8.90 mmol). After being stirred at 0 °C for 45 min, the resultant yellow suspension was cooled to –78 ºC. To this mixture at –78 ºC under Ar was added a solution of (+)-5 (296.1 mg, 0.891 mmol) in Et2O (1.5 mL) dropwise through a cannula. After being stirred at –78 °C for 30 min, the mixture was quenched with saturated aqueous NH4Cl solution at –78 ºC and the products were extracted with EtOAc. The extract was washed successively with saturated aqueous NH4Cl aqueous solution and saturated aqueous NaHCO3 solution, and then dried. The organic solvent was concentrated to give a residue, which was purified by column chromatography (15 g, EtOAc/toluene = 1/100 → 1/50 → 1/2 as an eluent) to afford 7a (61.1 mg, 15%), 7b (188.5 mg, 45%), and recovered starting material (+)-5 (80.1 mg, 27% recovery).
(4S,6R)-4-Benzyloxy-6-[(tert-butyldimethylsilyl)oxy]-3-(2,3-dimethoxyphenyl)-2-cyclohexen-1-one (8). To a solution of a diastereomeric mixture of 7a and 7b (7a : 7b = 4:1, 679.0 mg, 1.443 mmol) in 1,2-dichloroethane (34 mL) at rt were added activated MS4A (679 mg) and PCC (1.24 g, 5.77 mmol), and the mixture was stirred at 60 ºC for 1 h. Silica gel (10 g) was added to the reaction mixture and the resulting mixture was stirred vigorously at rt for 5 min. The mixture was diluted with Et2O and the insoluble material was removed by filtration through a pad of silica gel. The filtrate was concentrated to give a residue, which was purified by column chromatography (20 g, EtOAc/toluene = 1/50 as an eluent) to afford 8 (504.9 mg, 75%) as a colorless oil: [α]D29 –125 (c 1.34, CHCl3); IR (neat) νmax = 2950, 2930, 2860, 1690, 1470, 1270, 1160, 1110 cm-1; 1H NMR δ 7.23–7.16 (3H, m), 7.08 (1H, dd, J = 8.1 and 7.7 Hz), 7.01–6.92 (3H, m), 6.80 (1H, dd, J = 7.7 and 1.5 Hz), 6.09 (1H, d, J = 2.2 Hz), 5.03 (1H, ddd, J = 10.7, 5.0 and 2.2 Hz), 4.47 (2H, s), 4.32 (1H, dd, J = 13.2 and 5.0 Hz), 3.90 and 3.81 (each 3H, 2s), 2.65 (1H, ddd, J = 11.8, 5.0 and 5.0 Hz), 2.24 (1H, ddd, J = 13.2, 11.8 and 10.7 Hz), 0.95 (9H, s), 0.22 and 0.12 (each 3H, 2s); 13C NMR δ 198.0, 162.2, 152.4, 146.1, 137.9, 132.1, 128.1, 127.6, 127.5, 127.4, 124.2, 121.5, 113.2, 74.8, 72.9, 71.1, 60.9, 56.0, 39.7, 25.8, 18.5, –4.3, –5.4; HRMS (FAB) m/z calcd for C27H37O5Si (M+H)+ 469.2410, found 469.2411. Anal. Calcd for C27H36O5Si: C, 69.20; H, 7.74%. Found: C, 68.97; H, 7.87%.
(1R,4S,6R)-4-Benzyloxy-6-[(tert-butyldimethylsilyl)oxy]-3-(2,3-dimethoxyphenyl)-2-cyclohexen-1-ol (4) and Its (1S)-Epimer. To a solution of 8 (13.3 mg, 0.0284 mmol) in MeOH (0.2 mL) and CH2Cl2 (0.3 mL) was added cerium chloride heptahydrate (15.9 mg, 0.0427 mmol) at rt. After being stirred for 1 h, the reaction mixture was cooled to –78 ºC. To the mixture was added NaBH4 (1.1 mg, 0.029 mmol) at –78 ºC, and the resulting mixture was stirred at –78 °C for 30 min. The reaction mixture was quenched with water at –78 ºC and the products were extracted with EtOAc. The extract was washed with brine and then dried. The organic solvent was concentrated to give a residue, which was purified by column chromatography (1 g, EtOAc/toluene = 1/100 as an eluent) to afford 4 (11.9 mg, 89%) as a colorless oil: [α]D28 –82 (c 0.84, CHCl3); IR (neat) νmax = 3450, 2960, 2930, 2890, 2860, 1580, 1470, 1260, 1100 cm-1; 1H NMR δ 7.22–7.14 (3H, m), 7.02 (1H, dd, J = 8.0 and 7.7 Hz), 7.00–6.93 (2H, m), 6.89 (1H, dd, J = 8.0 and 1.4 Hz), 6.81 (1H, dd, J = 7.7 and 1.4 Hz), 5.73 (1H, dd, J = 2.1 and 2.0 Hz), 4.83 (1H, dddd, J = 10.0, 5.9, 2.1 and 2.0 Hz), 4.39 and 4.33 (each 1H, 2d, J =11.3 Hz), 4.28 (1H, ddd, J = 7.8, 2.0 and 2.0 Hz), 3.88 and 3.78 (each 3H, 2s), 3.71 (1H, ddd, J = 12.2, 7.8 and 3.4 Hz), 2.28 (1H, ddd, J = 12.2, 5.9 and 3.4 Hz), 2.22 (1H, bs), 1.82 (1H, ddd, J = 12.2, 12.2 and 10.0 Hz), 0.93 (9H, s), 0.14 and 0.13 (each 3H, 2s); 13C NMR δ 152.5, 146.6, 140.6, 138.6, 134.4, 129.7, 128.0, 127.6, 127.2, 123.9, 122.6, 111.8, 75.6, 74.3, 73.6, 70.9, 60.6, 55.9, 37.5, 25.8, 18.1, –4.3, –4.5; HRMS (FAB) m/z calcd for C27H39O5Si (M+H)+ 471.2567, found 471.2572. Anal. Calcd for C27H38O5Si: C, 68.90; H, 8.14%. Found: C, 68.62; H, 8.08%. Further elution afford the 1-epimer of 4 (1.2 mg, 9%) as a colorless oil: [α]D21 –37 (c 0.64, CHCl3); IR (neat) νmax = 3540, 2960, 2930, 2880, 2860, 1580,1470, 1260, 1230, 1090 cm-1; 1H NMR δ 7.21–7.11 (3H, m), 7.02 (1H, dd, J = 8.3 and 7.1 Hz), 6.96–6.85 (4H, m), 5.94 (1H, dd, J = 5.1 and 1.9 Hz), 4.71 (1H, dddd, J = 10.2, 6.0, 1.9 and 0.6 Hz), 4.36 (2H, s), 4.13 (1H, dddd, J = 5.0, 4.1, 1.0 and 0.6 Hz), 3.88 (1H, ddd, J = 11.6, 4.1 and 4.1 Hz), 3.87 and 3.79 (each 3H, 2s), 2.77 (1H, bs), 2.13 (1H, ddd, J = 11.6, 11.6 and 10.2 Hz), 2.00 (1H, dddd, J = 11.6, 6.0, 4.1 and 1.0 Hz), 0.93 (9H, s), 0.14 (6H, s); 13C NMR δ 152.3, 146.6, 144.4, 138.7, 134.3, 127.9, 127.5, 127.09, 127.06, 123.9, 122.7, 112.0, 74.8, 69.9, 68.8, 66.2, 60.6, 56.0, 31.9, 25.8, 18.1, –4.4, –4.8; HRMS (FAB) m/z calcd for C27H39O5Si (M+H)+ 471.2567, found 471.2569.
(1S,4R,6S)-6-Benzyloxy-4-[(tert-butyldimethylsilyl)oxy]-1-(2,3-dimethoxyphenyl)-2-cyclohexene-1-acetic Acid Ethyl Ester (3a). To a solution of 4 (71.7 mg, 0.152 mmol) in triethyl orthoacetate (10 mL) was added 2-nitrophenol (27.6 mg, 0.198 mmol) at rt. This mixture in a sealed tube filled with Ar was heated at 140 ºC for 52 h. The reaction mixture was diluted with EtOAc and washed with saturated aqueous NaHCO3 solution, and then dried. The organic solvent was concentrated to give a residue, which was purified by column chromatography (4.5 g, EtOAc/hexane = 1/30) to afford 3a (65.9 mg, 80%) as a colorless oil: [α]D26 –7.2 (c 0.38, CHCl3); IR (neat) νmax = 2960, 2940, 2860, 1730, 1580, 1470, 1260, 1230, 1100 cm-1; 1H NMR δ 7.35–7.21 (6H, m), 6.95 (1H, dd, J = 8.0 and 8.0 Hz), 6.83 (1H, dd, J = 8.0 and 1.4 Hz), 6.03 (1H, dd, J = 10.2 and 1.7 Hz), 5.66 (1H, ddd, J = 10.2, 1.5 and 1.0 Hz), 4.66 and 4.53 (each 1H, 2d, J = 11.7 Hz), 4.30 (1H, dddd, J = 9.3, 6.8, 1.7 and 1.5 Hz), 4.00 (2H, q, J = 7.1 Hz), 3.83 (1H, dd, J = 12.2 and 2.7 Hz), 3.82 and 3.75 (each 3H, 2s), 3.26 and 3.03 (each 1H, 2d, J = 14.9 Hz), 2.06 (1H, dddd, J = 12.2, 6.8 Hz, 2.7 and 1.0 Hz), 1.83 (1H, ddd, J = 12.2, 12.2 and 9.3 Hz), 1.13 (3H, t, J = 7.1 Hz), 0.87 (9H, s), 0.05 and 0.03 (each 3H, 2s); 13C NMR (CDCl3, 75 MHz) δ 171.8, 153.2, 149.5, 138.7, 134.2, 133.0, 130.4, 128.1, 127.9, 127.4, 124.1, 122.1, 111.6, 78.6, 71.7, 68.2, 60.3, 59.8, 55.8, 48.2, 42.4, 33.2, 25.9, 18.2, 14.1, –4.5, –4.6; LRMS (EI) m/z 540 (M+, 5%), 483 (4), 406 (41), 402 (15), 175 (31), 115 (19), 91 (77), 75 (100); HRMS (EI) m/z calcd for C31H44O6Si (M+) 540.2907, found 540.2908. Anal. Calcd for C31H44O6Si: C, 68.85; H, 8.20%. Found: C, 68.74; H, 8.19%.
2-[(1S,4R,6S)-6-Benzyloxy-4-[(tert-butyldimethylsilyl)oxy]-1-(2,3-dimethoxyphenyl)-2-cyclohexene-1-yl]-N,N-dimethylacetamide (3b). To a solution of 4 (112.2 mg, 0.2384 mmol) in o-xylene (11.2 mL) was added N,N-dimethylacetamide dimethyl acetal (0.350 mL, 2.66 mmol) at rt. This mixture in a sealed tube filled with Ar was heated at 150 ºC for 23 h. The reaction mixture was concentrated to give a residue, which was purified by column chromatography (5 g, EtOAc/toluene = 1/3) to afford 3b (118.3 mg, 92%) as a colorless oil: [α]D26 +5.8 (c 0.60, CHCl3); IR (neat) νmax = 2930, 2960, 2860, 1650, 1470, 1260, 1100 cm-1; 1H NMR δ 7.46 (1H, dd, J = 8.0 and 1.5 Hz), 7.38–7.21 (5H, m), 6.97 (1H, dd, J = 8.0 and 8.0 Hz), 6.81 (1H, dd, J = 8.0 and 1.5 Hz), 6.21 (1H, dd, J = 10.2 and 1.7 Hz), 5.61 (1H, ddd, J = 10.2, 2.0 and 1.2 Hz), 4.71 and 4.50 (each 1H, 2d, J = 12.1 Hz), 4.40 (1H, dddd, J = 9.5, 6.3, 2.0 and 1.7 Hz), 4.08 (1H, dd, J = 12.2 and 2.4 Hz), 3.83 and 3.78 (each 3H, 2s), 3.40 and 2.74 (each 1H, 2d, J = 15.6 Hz), 2.92 and 2.86 (each 3H, 2s), 2.15 (1H, dddd, J = 12.2, 6.3, 2.4 and 1.2 Hz), 1.96 (1H, ddd, J = 12.2, 12.2 and 9.5 Hz), 0.86 (9H, s), 0.044 and 0.035 (each 3H, 2s); 13C NMR δ 171.4, 153.2, 148.9, 139.0, 136.0, 133.9, 129.8, 128.2, 127.9, 127.3, 123.7, 122.5, 111.0, 78.4, 71.4, 68.7, 60.3, 55.7, 48.7, 39.2, 37.6, 35.3, 33.4, 25.9, 18.2, –4.5, –4.6; LRMS (EI) m/z 539 (M+, 15.4%), 508 (59.2), 448 (66.0), 405 (100), 333 (69.2), 316 (43.0), 199 (59.9); HRMS (EI) m/z calcd for C31H45NO5Si (M+) 539.3067, found 539.3065.
(5aS,6R,7R,9S,9aS)-9-Benzyloxy-6-bromo-7-[(tert-butyldimethylsilyl)oxy]-6,7,8,9-tetrahydro-4-methoxy-9a(5aH)-dibenzofuranacetic Acid Ethyl Ester (9). To a solution of 3a (66.3 mg, 0.123 mmol) in DMF (4.3 mL) was added NBS (43.6 mg, 0.245 mmol) at 0 ºC. After being stirred at 0 °C for 12 h, the reaction mixture was quenched with saturated aqueous NaHCO3 solution at 0 ºC and the products were extracted with EtOAc. The extract was washed with saturated aqueous NaHCO3 solution and then dried. The organic solvent was concentrated to give a residue, which was purified by column chromatography (3 g, EtOAc/toluene = 1/100 as an eluent) to afford 9 (62.3 mg, 84%) as a colorless oil: [α]D26 +26 (c 1.78, CHCl3); IR (neat) νmax = 2960, 2930, 2860, 1730, 1640, 1590, 1490, 1460, 1260, 1200, 1140, 1090 cm-1; 1H NMR δ 7.44–7.28 (5H, m), 7.22 (1H, dd, J = 6.8 and 1.4 Hz), 6.82–6.76 (2H, m), 5.18 (1H, d, J = 7.6 Hz), 4.71 and 4.52 (each 1H, 2d, J = 11.6 Hz), 4.19–4.00 (3H, m), 3.87 (3H, s), 3.76–3.62 (2H, m), 2.89 and 2.48 (each 1H, 2d, J = 16.1 Hz), 2.27 (1H, ddd, J = 12.7, 3.4 and 3.4 Hz), 1.53 (1H, m), 1.22 (3H, t, J = 7.2 Hz), 0.86 (9H, s), 0.13 and 0.07 (each 3H, 2s); 13C NMR δ 171.0, 146.6, 145.9, 138.1, 132.0, 128.5, 127.81, 127.78, 122.3, 118.8, 113.2, 90.2, 75.4, 72.1, 69.8, 60.5, 60.1, 56.3, 54.5, 39.7, 36.3, 25.7, 18.0, 14.1, –4.4, –4.5; LRMS (EI) m/z 606 [M+ (81Br), 4%], 604 [M+ (79Br), 4], 549 (4), 547 (4), 531 (4), 529 (3), 337 (42), 247 (93), 91 (100), 73 (39); HRMS (EI) m/z calcd for C30H4179BrO6Si (M+) 604.1856, found 604.1857.
(5aR,7R,9S,9aS)-7-[(tert-Butyldimethylsilyl)oxy]-6,7,8,9-tetrahydro-9-hydroxy-4-methoxy-9a(5aH)-dibenzofurnacetic Acid Ethyl Ester (11). To a solution of 9 (27.0 mg, 0.0447 mmol) in EtOH (1.5 mL) was added 10 % Pd on carbon (12 mg) and the mixture was stirred under an atmospheric pressure of H2 at rt for 1 h. After confirming the disappearance of 9 by TLC analysis, potassium carbonate (6.2 mg, 0.045 mmol) was added to the mixture and the resulting mixture was further stirred under an atmospheric pressure of H2 at rt for 1 h. The reaction mixture was filtrated through a pad of Celite. The filtrate was concentrated to give a residue, which was purified by column chromatography (1.5 g, EtOAc/toluene = 1/30 as an eluent) to afford 11 (16.6 mg, 85%) as a colorless oil: [α]D23 +44 (c 0.56, CHCl3); IR (neat) νmax = 3530, 2950, 2940, 2860, 1730, 1620, 1590, 1490, 1450, 1260, 1190, 1180, 1120 cm-1; 1H NMR δ 7.15 (1H, dd, J = 7.0 and 2.0 Hz), 6.83 (1H, dd, J = 8.1 and 7.0 Hz), 6.78 (1H, dd, J = 8.1 and 2.0 Hz), 4.75 (1H, dd, J = 8.6 and 6.4 Hz), 4.06 (3H, m), 3.86 (3H, s), 3.75 (1H, dddd, J = 9.8, 9.8, 4.7 and 4.4 Hz), 3.47 (1H, d, J = 4.4 Hz), 2.90 and 2.59 (each 1H, 2d, J = 14.8 Hz), 2.23 (1H, dddd, J = 12.7, 6.4, 4.7 and 1.7 Hz), 2.04 (1H, dddd, J = 10.7, 4.4, 4.4 and 1.7 Hz), 1.73-1.52 (2H, m), 1.16 (3H, t, J = 7.1 Hz), 0.83 (9H, s), 0.033 and 0.025 (each 3H, 2s); 13C NMR δ 172.2, 147.2, 145.6, 131.2, 121.6, 118.5, 112.3, 85.5, 71.2, 65.1, 60.9, 56.0, 52.7, 42.3, 39.6, 37.5, 25.7, 18.0, 14.0, –4.8 (2C); LRMS (EI) m/z 436 (M+, 26%), 379 (32), 287 (61), 241 (85), 235 (78), 234 (89), 213 (100), 199 (66), 161 (76), 75 (63), 73 (56); HRMS (EI) m/z calcd for C23H36O6Si (M+) 436.2281, found 436.2280. Anal. Calcd for C23H36O6Si: C, 63.27; H, 8.31%. Found: C, 62.98; H, 8.25%.
(5aR,7R,9S,9aR)-9-Benzoyloxy-7-[(tert-butyldimethylsilyl)oxy]-6,7,8,9-tetrahydro-4-methoxy-9a(5aH)-dibenzofurnacetic Acid Ethyl Ester (12). To a solution of 11 (7.5 mg, 0.017 mmol) in pyridine (0.5 mL) were added benzoyl chloride (3.0 μL, 0.026 mmol) and DMAP (0.2 mg, 2 μmol) at rt. After being stirred at rt for 27 h, the reaction mixture was quenched with saturated aqueous NH4Cl solution and the products were extracted with Et2O. The extract was washed with brine and then dried. The organic solvent was concentrated to give a residue, which was purified by column chromatography (0.5 g, EtOAc/hexane = 1/50 as an eluent) to afford 12 (11.3 mg, 100%) as a colorless oil: [α]D23 –38 (c 0.42, CHCl3); IR (neat) νmax = 2950, 2930, 2860, 1730, 1490, 1455, 1270, 1095 cm-1; 1H NMR δ 8.11 (2H, m), 7.63 (1H, m), 7.52 (2H, m), 7.27 (1H, dd, J = 7.5 and 1.0 Hz), 6.92 (1H, dd, J = 7.5 and 8.0 Hz), 6.83 (1H, dd, J = 8.0 and 1.0 Hz), 5.55 (1H, dd, J = 11.9 and 3.9 Hz), 5.23 (1H, dd, J = 9.0 and 6.4 Hz), 4.10-4.00 (2H, m), 3.88 (3H, s), 3.94 - 3.82 (1H, m), 2.67 and 2.57 (each 1H, 2d, J = 15.3 Hz), 2.37 (1H, dddd, J = 13.4, 6.4, 4.9 and 1.7 Hz), 2.24 (1H, dddd, J = 11.9, 4.6, 3.9 and 1.7 Hz), 1.69 (1H, ddd, J = 11.9, 11.9 and 10.5 Hz), 1.61 (1H, ddd, J = 13.4, 10.2 and 9.0 Hz), 1.19 (3H, t, J = 7.1 Hz), 0.82 (9H, s), 0.05 and 0.03 (each 3H, 2s); 13C NMR δ 170.7, 165.9, 147.2, 145.9, 133.2, 131.4, 130.7, 129.6, 128.6, 121.8, 117.7, 112.2, 84.1, 72.0, 64.6, 60.6, 55.9, 52.3, 40.1, 38.1, 37.1, 25.7, 17.9, 13.9, –4.78, –4.81; LRMS (EI) m/z 540 (M+, 5%), 483 (18), 343 (35), 241 (35), 179 (32), 105 (100), 77 (38); HRMS (EI) m/z calcd for C30H40O7Si (M+) 540.2543, found 540.2539.
(3aS,4S,6R,7R,7aR)-7-Bromo-3a-(2,3-dimethoxyphenyl)-4,6-dihydroxy-hexahydro-2(3H)-benzofuranone (13). To a solution of 3b (29.1 mg, 0.0539 mmol) in DMF (3.5 mL) was added NBS (18.2 mg, 0.102 mmol) at 0 ºC and the mixture was stirred at rt. After being stirred for 3 h, NBS (18.2 mg, 0.102 mmol) was added at 0 ºC and mixture was further stirred at rt for 2 h. The reaction mixture was quenched with saturated aqueous NaHCO3 solution at 0 ºC and the products were extracted with EtOAc. The extract was washed with saturated aqueous NaHCO3 solution and then dried. Removal of the solvent left a residue, which was purified by column chromatography (1.5 g, EtOAc/toluene = 1/100 as an eluent) and further purified by preparative TLC (EtOAc/toluene =1/5) to afford 10 (11.8 mg, 37%) as a colorless oil. Compound 10 (11.8 mg, 0.199 mmol) was dissolved in EtOH (2 mL). To the mixture was added 10 % Pd on carbon (8 mg) and the mixture was stirred under an atmospheric pressure of H2 at rt for 2 h. The mixture was filtrated through a pad of Celite and the filtrate was concentrated to give a residue, which was purified by column chromatography (1 g, EtOAc/toluene = 1/1 as an eluent) to afford 13 (5.3 mg, 25% from 3b) as an amorphous solid: [α]D24 –14 (c 0.27, CHCl3); IR (neat) νmax = 3450, 1780, 1470, 1270 cm-1; 1H NMR δ 7.07 (1H, dd, J = 8.5 and 8.3 Hz), 6.95 (1H, dd, J = 8.3 and 1.5 Hz), 6.90 (1H, dd, J = 8.5 and 1.5 Hz), 5.54 (1H, d, J = 9.5 Hz), 4.89 (1H, dd, J = 3.7 and 3.4 Hz), 4.34 (1H, ddd, J = 3.2, 2.7 and 2.7 Hz), 4.15 (1H, dd, J = 9.5 and 2.7 Hz), 3.95 and 3.87 (each 3H, 2s), 2.95 and 2.76 (each 1H, 2d, J = 17.3 Hz), 2.57 (1H, ddd, J = 15.6, 3.7 and 3.2 Hz), 2.15 (1H, ddd, J = 15.6, 3.4 and 2.7 Hz); 13C NMR δ 173.7, 153.4, 147.5, 131.6, 123.8, 119.5, 113.3, 81.9, 71.1, 68.9, 60.4, 57.9, 55.8, 55.1, 38.4, 32.3; LRMS (EI) m/z 388 [M+ (81Br), 9%], 386 [M+(79Br), 9], 356 (92), 354 (100), 289 (10), 243 (31), 189 (53), 77 (47); HRMS (EI) m/z calcd for C16H1979BrO6 (M+) 386.0365, found 386.0369.
(5aR,7S,9aR)-7-[(tert-Butyldimethylsilyl)oxy]-6,7-dihydro-4-methoxy-9a(5aH)-dibenzofuranacetic Acid Ethyl Ester (14) To a solution of 11 (12.4 mg, 0.0284 mmol) in 1,2-dichlorobenzene (2.0 mL) were added thiocarbonyldiimidazole (20.2 mg, 0.113 mmol) and DMAP (0.4 mg, 0.3 μmol). The reaction mixture was heated at reflux temperature. After being refluxed for 6 h, the reaction mixture was cooled to 0 °C and quenched with saturated aqueous NH4Cl solution. The products were extracted with EtOAc. The extract was washed with saturated aqueous NaHCO3 solution and then dried. Removal of the solvent left a residue, which was purified by column chromatography (1 g, EtOAc/hexane = 1/10 as an eluent) to afford 14 (8.6 mg, 72%) as a colorless oil: [α]D28 –22 (c 0.80, CHCl3); IR (neat) νmax = 2960, 2930, 2860, 1730, 1620, 1590, 1500, 1460, 1260, 1200, 1100 cm-1; 1H NMR δ 6.88–6.71 (3H, m), 5.93 (1H, dd, J = 10.0 and 2.0 Hz), 5.82 (1H, ddd, J = 10.0, 1.8 and 1.2 Hz), 5.24 (1H, dd, J = 11.6 and 5.6 Hz), 4.35 (1H, dddd, J = 10.2, 4.4, 2.0 and 1.8 Hz), 4.08 (2H, q, J = 7.1 Hz), 3.87 (3H, s), 2.62 and 2.54 (each 1H, 2d, J = 14.6 Hz), 2.33 (1H, dddd, J = 11.6, 5.6, 4.4 and 1.2 Hz), 1.70 (1H, ddd, J = 11.6, 11.6 and 10.2 Hz), 1.21 (3H, t, J = 7.1 Hz), 0.85 (9H, s), 0.060 and 0.055 (each 3H, 2s); 13C NMR δ 170.4, 145.8, 145.3, 134.6, 133.1, 126.8, 121.5, 115.4, 111.6, 84.4, 65.2, 60.4, 55.9, 48.3, 44.7, 37.0, 25.7, 18.0, 14.2, –4.6, –4.8; LRMS (EI) m/z 418 (M+, 8%), 361 (23), 343 (66), 297 (42), 269 (66), 254 (72), 181 (50), 75 (100); HRMS (EI) m/z calcd for C23H34O5Si (M+) 418.2176, found 418.2173. Anal. Calcd for C23H34O5Si: C, 65.99; H, 8.19%. Found: C, 65.81; H, 8.22%.
(5aR,7S,9aR)-7-[(tert-Butyldimethylsilyl)oxy]-6,7-dihydro-4-methoxy-N-methyl-9a(5aH)-dibenzofuranacetamide (15). To a solution of 14 (7.6 mg, 0.018 mmol) in EtOH (0.4 mL) and water (0.1 mL) was added lithium hydroxide monohydrate (0.9 mg, 0.02 mmol) at rt. After being stirred at rt for 20 h, the reaction mixture was quenched with saturated aqueous NH4Cl solution at 0 ºC and the products were extracted with Et2O. The extract was washed with brine and then dried. Removal of the solvent gave a carboxylic acid as a syrup, which was used for the next reaction without further purification. To a solution of a crude carboxylic acid in DMF (0.4 mL) were added methylammonium chloride (6.1 mg, 0.091 mmol), diethyl cyanophosphonate (0.027 mL, 0.18 mmol) and Et3N (0.025 mL, 0.18 mmol) at –10 ºC. After being stirred at –10 ºC for 2 h, the reaction mixture was quenched with saturated aqueous NH4Cl solution and the products were extracted with EtOAc. The extract was washed with brine and then dried. The organic solvent was concentrated to give a residue, which was purified by column chromatography (0.5 g, EtOAc/hexane = 1/1) to afford 15 (6.8 mg, 93% for 2 steps) as a crystalline residue: mp 58–60 ºC; [α]D24 –3.5 (c 0.98, CHCl3); IR (KBr disk) νmax = 3310, 2950, 2930, 2860, 1650, 1490, 1260, 1090 cm-1; 1H NMR δ 6.83 (1H, dd, J = 8.0 and 7.3 Hz), 6.74 (1H, dd, J = 8.0 and 1.5 Hz), 6.71 (1H, dd, J = 7.3 and 1.5 Hz), 5.99 (1H, dd, J = 10.1 and 2.0 Hz), 5.82 (1H, ddd, J = 10.1, 1.9 and 1.2 Hz), 5.19 (1H, dd, J = 11.6 and 2.0 Hz), 5.18 (1H, bs,), 4.37 (1H, dddd, J = 10.4, 4.9, 2.0 and 1.9 Hz), 3.86 (3H, s), 2.69 (3H, d, J = 4.9 Hz), 2.48 and 2.34 (each 1H, 2d, J = 13.8 Hz), 2.31 (1H, dddd, J = 11.7, 4.9, 2.0 and 1.2 Hz), 1.69 (1H, ddd, J = 11.7, 11.6 and 10.4 Hz), 0.84 (9H, s), 0.054 and 0.047 (each 3H, 2s); 13C NMR δ 170.0, 145.9, 145.4, 134.2, 133.3, 127.1, 121.4, 115.4, 111.7, 84.9, 65.2, 56.0, 48.4, 47.0, 36.9, 26.2, 25.7, 18.0, –4.6, –4.8; LRMS (EI) m/z 403 (M+, 9%), 346 (42), 328 (100), 75 (45), 73 (40); HRMS (EI) m/z calcd for C22H33NO4Si (M+) 403.2179, found 403.2165.
(5aR,7S,9aR)-6,7-Dihydro-7-hydroxy-4-methoxy-N-methyl-9a(5aH)-dibenzofuranacetamide (16). To a solution of 15 (7.6 mg, 0.019 mmol) in THF (0.4 mL) was added TBAF (1.0 M THF solution, 0.038 mL, 0.038 mmol) at rt and the mixture was stirred at 40 ºC for 10 h. The reaction mixture was quenched with saturated aqueous NH4Cl solution and the products were extracted with EtOAc. The extract was washed with brine and then dried. Removal of the solvent left a residue, which was purified by column chromatography (0.3 g, EtOAc as an eluent) to afford 16 (5.8 mg, 100%) as a colorless oil: [α]D24 –13 (c 0.58, CHCl3); IR (neat) νmax = 3310, 3100, 3000, 2940, 2840, 1650, 1490, 1460, 1280, 1070 cm-1; 1H NMR δ 6.90 (1H, dd, J = 7.8 and 7.8 Hz), 6.80 (1H, dd, J = 7.8 and 1.2 Hz), 6.78 (1H, dd, J = 7.8 and 1.2 Hz), 5.98 (1H, ddd, J = 10.1, 4.6 and 0.5 Hz), 5.73 (1H, ddd, J = 10.1, 1.0 and 1.0 Hz), 5.47 (1H, bs), 5.08 (1H, dd, J = 4.1 and 3.2 Hz), 4.20 (1H, m), 3.86 (3H, s), 2.74 (3H, d, J = 4.9 Hz), 2.61 and 2.57 (each 1H, 2d, J = 14.9 Hz), 2.43 (1H, dddd, J = 14.7, 4.1, 4.1 and 1.0 Hz), 2.22 (1H, ddd, J = 14.7, 4.9 and 3.2 Hz), 2.18 (1H, d, J = 9.7 Hz); 13C NMR δ 169.6, 145.9, 145.2, 133.9, 129.6, 129.2, 122.2, 114.9, 111.9, 86.2, 62.4, 55.9, 47.3, 44.5, 31.9, 26.3; LRMS (EI) m/z 289 (M+, 34%), 216 (100), 201 (33), 199 (42), 103 (39); HRMS (EI) m/z calcd for C16H19NO4 (M+) 289.1314, found 289.1311.
(4aR,6S,8aR)-4a,5,11,12-Tetrahydro-6-hydroxy-3-methoxy-11-methyl-6H-benzofuro[3a,3,2-ef][2]benzazepin-10(9H)-one (2). To a solution of 16 (3.9 mg, 0.014 mmol) in 1,2-dichloroethane (1.2 mL) were added paraformaldehyde (1.6 mg, 0.054 mmol) and TFA (0.02 mL) at rt. After being stirred at rt for 2.5 h, the reaction mixture was quenched with saturated aqueous NaHCO3 solution at 0 ºC and the products were extracted with CHCl3. The extract was washed with brine and then dried. Removal of the solvent left a residue, which was purified by column chromatography (0.5 g, MeOH/CHCl3 = 1/200) to afford 2 (2.9 mg, 71%) as a crystalline residue: mp 213–214 ºC; [α]D25 +115 (c 0.63, CHCl3); IR (KBr disk) νmax = 3450, 2930, 1650, 1640, 1510, 1440, 1280, 1060 cm-1; 1H NMR δ 6.70 (2H, s), 6.03 (1H, ddd, J = 10.1, 5.1 and 1.4 Hz), 5.49 (1H, d, J = 10.1 Hz), 4.74 (1H, dd, J = 2.0 and 1.7 Hz), 4.47 and 4.35 (each 1H, 2d, J = 16.1 Hz ) 4.22 – 4.09 (1H, m), 3.85 and 3.02 (each 3H, 2s), 2.82 and 2.75 (each 1H, 2d, J = 13.7 Hz), 2.68 (1H, dddd, J = 15.8, 3.9, 1.7 and 1.4 Hz), 2.48 (1H, d, J = 11.5 Hz), 2.12 (1H, ddd, J = 15.8 Hz, 5.4 and 2.0 Hz); 13C NMR δ 170.9, 146.6, 144.8, 132.1, 128.3 (2C), 125.1, 120.0, 111.9, 88.3, 61.5, 56.1, 52.0, 43.3, 41.6, 35.9, 29.2; LRMS (EI) m/z 302 [(M+H)+, 9%], 301 (M+, 100), 283 (24), 230 (21), 224 (23), 211 (21), 181 (19), 165 (25), 115 (36), 91 (22), 77 (24); HRMS (EI) m/z calcd for C17H19NO4 (M+) 301.1314, found 301.1317. Anal. Calcd for C17H19NO4・1/2H2O: C, 65.79; H, 6.50; N, 4.51%. Found: C, 65.46; H, 6.37; N, 4.50%.
The similar treatment of compound 15 (3.5 mg, 0.0087 mmol) also provided 2 (1.8 mg, 67%).
(+)-Galanthamine [(+)-1]. To a solution of 2 (9.4 mg, 0.031 mmol) in THF (1.5 mL) under Ar at 0 ºC was added LiAlH4 (2.0 M solution in THF, 0.062 mL, 0.124 mmol) and the mixture was stirred at reflux temperature for 3 h. The reaction mixture was quenched with 3 mol/L aqueous NaOH solution at 0 ºC and the products were extracted with CH2Cl2. The extract was washed with brine and then dried over sodium carbonate. The organic solvent was concentrated to give a residue, which was purified by column chromatography (0.5 g, MeOH/CHCl3 = 1/20) to afford (+)-1 (7.9 mg, 88%) as a crystalline residue: mp 124–125 ºC (lit.,1c mp 125–126 °C); [α]D23 +112 (c 0.50, EtOH); IR (KBr disk) νmax = 3360, 2920, 1620, 1590, 1510, 1440, 1280, 1050 cm-1; 1H NMR δ 6.66 (1H, d, J = 8.3 Hz), 6.62 (1H, d, J = 8.3 Hz), 6.06 (1H, dd, J = 10.3 and 1.2 Hz), 6.00 (1H, ddd, J = 10.3, 4.9 and 1.3 Hz), 4.61 (1H, bs), 4.14 (1H, dd, J = 4.9 and 4.9 Hz), 4.08 (1H, d, J = 15.1 Hz), 3.83 (3H, s), 3.68 (1H, d, J = 15.1 Hz), 3.26 (1H, ddd, J = 14.6, 13.0 and 1.7 Hz), 3.05 (1H, ddd, J = 14.6, 3.9 and 3.2 Hz), 2.68 (1H, dddd, J = 15.6, 3.2, 1.5 and 1.3 Hz), 2.40 (3H, s), 2.08 (1H, ddd, J = 13.7, 13.0 and 3.2 Hz), 2.00 (1H, ddd, J = 15.6, 4.9 and 2.4 Hz), 1.57 (1H, ddd, J = 13.7, 3.9 and 1.7 Hz); 13C NMR δ 145.8, 144.2, 133.0, 128.5, 127.7, 126.7, 122.2, 111.2, 88.7, 62.0, 60.4, 55.9, 53.7, 48.1, 41.8, 33.6, 29.9; LRMS (EI) m/z 288 ((M+H)+, 18%), 287 (M+, 100), 286 (84), 269 (14), 230 (20), 174 (19); HRMS (EI) m/z calcd for C17H19NO4 (M+) 287.1521, found 287.1521.
Synthesis of (–)-Galanthamine [(–)-1] from (–)-5. Starting from (–)-5,12 (–)-galanthamine [(–)-1] was synthesized by the same procedure as described for the preparation of (+)-1 from (+)-5. ent-7a: [α]D21 –4.6 (c 1.40, CHCl3). ent-7b: [α]D21 –116 (c 1.16, CHCl3). ent-8: [α]D24 +120 (c 1.12, CHCl3). ent-4: [α]D24 +84 (c 1.28, CHCl3). ent-3a: [α]D24 +8.0 (c 0.76, CHCl3). ent-9: [α]D24 –27 (c 0.92, CHCl3). ent-11: [α]D24 –48 (c 0.76, CHCl3). ent-14: [α]D24 +21 (c 1.11, CHCl3). ent-15: [α]D24 +3.2 (c 1.69, CHCl3). ent-16: [α]D26 +11 (c 1.11, CHCl3). ent-2: [α]D24 –110 (c 0.45, CHCl3). (–)-1: [α]D23 –112 (c 0.19, EtOH) {lit.,1c [α]D20 –118.8 (c 1.378, EtOH)}.
References
1. (a) N. F. Proskurnina and A. P. Yakovleva, Zhur. Obschchei. Khim., 1952, 22, 1899; (b) S. Kobayashi, T. Shingu, and S. Uyeo, Chem. Ind., 1956, 177; (c) ‘The Merck Index, Twelfth Edition,’ ed. by S. Budavari, Merck & CO., Inc., Whitehouse Station, NJ, 1996, p. 736.
2. For comprehensive reviews on biological activities and synthetic studies of galanthamine-type alkaloids, see (a) S. F. Martin, ‘The Alkaloids,’ Vol. 30, ed. by A. Brossi, Academic Press, New York, NY, 1987, pp. 251-376; (b) O. Hoshino, ‘The Alkaloids’, Vol. 51, ed. by G. A. Cordell, Academic Press, New York, NY, 1998, pp. 323-424; (c) J. Marco-Contelles, M. D. C. Carreiras, C. Rodriguez, M. Villarroya, and A. G. Garcia, Chem. Rev., 2006, 106, 116; CrossRef (d) Z. Jin, Nat. Prod. Rep., 2009, 26, 363. CrossRef
3. (a) M. Rainer, Drugs Today, 1997, 33, 273; CrossRef H. A. M. Mucke, Drugs Today, 1997, 33, 259; CrossRef E. Giacobini, Neurochem. Int., 1998, 32, 413; CrossRef M. Weinstock, CNS Drugs, 1999, 12, 307; J. J. Sramek, E. J. Frackiewicz, and N. R. Culter, Expert Opin. Invest. Drugs, 2000, 9, 2393; CrossRef (b) G. Orhan, I. Orhan, and B. Sener, Lett. Drug Des. Disc., 2006, 3, 268. CrossRef
4. S. Lilienfeld, CNS Drug Rev., 2002, 8, 159.
5. Recent reports on racemic syntheses of galanthamine, see (a) M. Node, S. Kodama, Y. Hamashima, T. Baba, N. Hamamichi, and K. Nishide, Angew. Chem. Int. Ed., 2001, 40, 3060; CrossRef (b) C. Guillou, J.-L. Beunard, E. Gras, and C. Thal, Angew. Chem. Int. Ed., 2001, 40, 4745; CrossRef (c) X.-D. Hu, Y. Q. Tu, E. Zhang, S. Gao, S. Wang, A. Wang, C.-A. Fan, and M. Wang, Org. Lett., 2006, 8, 1823; CrossRef (d) T. Ishikawa, K. Kudo, K. Kuroyabu, S. Uchida, T. Kudoh, and S. Saito, J. Org. Chem., 2008, 73, 7498; CrossRef (e) P. Magnus, N. Sana, B. P. Fauber, and V. Lynch, J. Am. Chem. Soc., 2009, 131, 16045. CrossRef
6. For chiral total syntheses of (+)- and (–)-galanthamine, see (a) K. Tomioka, K. Shimizu, S.-i. Yamada, and K. Koga, Heterocycles, 1977, 6, 1752; CrossRef K. Shimizu, K. Tomioka, S.-i. Yamada, and K. Koga, Heterocycles, 1977, 8, 277; CrossRef K. Shimizu, K. Tomioka, S.-i. Yamada, and K. Koga, Chem. Pharm. Bull., 1978, 26, 3765; (–)-Galanthamine, see (b) B. M. Trost and W. Tang, Angew. Chem. Int. Ed., 2002, 41, 2795; CrossRef B. M. Trost, W. Tang, and F. D. Toste, J. Am. Chem. Soc., 2005, 127, 14785; CrossRef (c) S. Kodama, Y. Hamashima, K. Nishide, and M. Node, Angew. Chem. Int. Ed., 2004, 43, 2659; CrossRef M. Node, S. Kodama, Y. Hamashima, T. Katoh, K. Nishide, and T. Kajimoto, Chem. Pharm. Bull., 2006, 54, 1662; CrossRef (d) V. Satcharoen, N. J. McLean, S. C. Kemp, N. P. Camp, and R. C. D. Brown, Org. Lett., 2007, 9, 1867; CrossRef (e) J. M. Reddy, K. V. Kumar, V. Raju, B. V. Bhaskar, V. Himabindu, A. Bhattacharya, V. Sundaram, R. Banerjee, G. M. Reddy, and R. Bandichhor, Synth. Commun., 2008, 38, 2138; CrossRef For preparation of (–)-galanthamine via spontaneous resolution of racemic narwedine, see (f) W.-C. Shieh and J. A. Carlson, J. Org. Chem., 1994, 59, 5463; CrossRef (g) B. Küenburg, L. Czollner, J. Fröhlich, and U. Jordis, Org. Process Res. Dev., 1999, 3, 425. CrossRef
7. J. Bastida, F. Viladomat, J. M. Llabrés, S. Quiroga, C. Codina, and M. Rubiralta, Planta Med., 1990, 56, 123. CrossRef
8. A part of this work has been reported as a preliminary communication, see H. Tanimoto, T. Kato, and N. Chida, Tetrahedron Lett., 2007, 48, 6267. CrossRef
9. (a) M. Bohno, H. Imase, and N. Chida, Chem. Commun., 2004, 1086; CrossRef (b) M. Bohno, K. Sugie, H. Imase, Y. B. Yusof, T. Oishi, and N. Chida, Tetrahedron, 2007, 63, 6977. CrossRef
10. Construction of benzofuran skeletons by way of the bromonium ion-mediated cyclization using Br2, see J. Mulzer, J. W. Bats, B. List, T. Opatz, and D. Trauner, Synlett, 1997, 441. CrossRef
11. For utilization of Claisen rearrangement in the synthesis of related alkaloids, see (a) G. E. Keck and R. R. Webb, II, J. Org. Chem., 1982, 47, 1302; CrossRef (b) S. Labidalle, Z. Y. Min, A. Reynet, H. Moskowitz, J.-M. Vierfond, and M. Miocque, Tetrahedron, 1988, 44, 1159; CrossRef (c) P. J. Parsons, C. S. Penkett, and A. J. Shell, Chem. Rev., 1996, 96, 195; CrossRef (d) J. M. Schkeryantz and W. H. Pearson, Tetrahedron, 1996, 52, 3107; CrossRef (e) N. Chida, K. Sugihara, S. Amano, and S. Ogawa, J. Chem. Soc. Perkin Trans. 1, 1997, 275; CrossRef (f) J. Mulzer and D. Trauner, Chirality, 1999, 11, 475; CrossRef see also ref. 10. (g) F. W. Ng, H. Lin, and S. J. Danishefsky, J. Am. Chem. Soc., 2002, 124, 9812; CrossRef (h) H. Tanimoto, R. Saito, and N. Chida, Tetrahedron Lett., 2008, 49, 358. CrossRef
12. S. Imuta, H. Tanimoto, K. M. Momose, and N. Chida, Tetrahedron, 2006, 62, 6926. CrossRef
13. (a) R. J. Ferrier and S. Middleton, Chem. Rev., 1993, 93, 2779; CrossRef (b) R. J. Ferrier and S. Middleton, Top. Curr. Chem., 2001, 215, 277; CrossRef (c) N. Chida, M. Ohtsuka, K. Ogura, and S. Ogawa, Bull. Chem. Soc. Jpn., 1991, 64, 2118. CrossRef
14. Oxidation of a mixture of 7a and 7b with PCC in the presence of NaOAc (1 equiv to a 4:1 mixture of 7a and 7b) in 1,2-dichloroethane at 60 °C also afforded 8 in 75% yield. It was found that the use of MS4A as an additive in the PCC oxidation of 7a and 7b showed good reproducibility when the reaction was carried out in large scale.
15. A. L. Gemal and J.-L. Luche, J. Am. Chem. Soc., 1981, 103, 5454. CrossRef
16. W. S. Johnson, L. Werthemann, W. R. Bartlett, T. J. Brocksom, T.-T. Li, D. J. Faulkner, and M. R. Petersen, J. Am. Chem. Soc., 1970, 92, 741; CrossRef A. M. M. Castro, Chem. Rev., 2004, 104, 2939. CrossRef
17. T. Fukazawa, Y. Shimoji, and T. Hashimoto, Tetrahedron: Asymmetry, 1996, 7, 1649. CrossRef
18. D. Trauner, J. W. Bats, A. Werner, and J. Mulzer, J. Org. Chem., 1998, 63, 5908. CrossRef
19. A. E. Wick, K. Steen, and A. Eschenmoser, Helv. Chim. Acta, 1964, 47, 2425. CrossRef
20. (a) M.-F. Ruasse, S. Motallebi, and B. Galland, J. Am. Chem. Soc., 1991, 113, 3440; CrossRef (b) G. Bellucci, R. Bianchini, C. Chiappe, R. Stanley, and H. Slebocka-Tilk, J. Am. Chem. Soc., 1991, 113, 8012. CrossRef
21. D. R. Williams, P. J. Coleman, and S. S. Henry, J. Am. Chem. Soc., 1993, 115, 11654. CrossRef
22. S.-i. Yamada, Y. Kasai, and T. Shioiri, Tetrahedron Lett., 1973, 14, 1595; CrossRef T. Shioiri, Y. Yokoyama, Y. Kasai, and S.-i. Yamada, Tetrahedron, 1976, 32, 2211 CrossRef