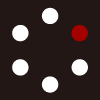
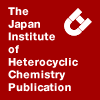
HETEROCYCLES
An International Journal for Reviews and Communications in Heterocyclic ChemistryWeb Edition ISSN: 1881-0942
Published online by The Japan Institute of Heterocyclic Chemistry
e-Journal
Full Text HTML
Received, 30th August, 2010, Accepted, 28th September, 2010, Published online, 30th September, 2010.
DOI: 10.3987/COM-10-S(E)118
■ Structures of Fukinone Epoxides. Configuration, Conformation, and CD Spectra
Yoshinori Saito and Motoo Tori*
Faculty of Pharmaceutical Sciences, Tokushima Bunri University, 180 Nishihamabouji, Yamashiro-machi, Tokushima, 770-8514, Japan
Abstract
The configuration and conformation of two diastereoisomeric fukinone epoxides were unambiguously established by NMR (NOESY), CD, and X-ray crystallographic analyses of their derivatives. The data show the absolute configurations of exo α,β-epoxy ketones can be predicted by an octant rule, not by a Reverse Octant Rule.The structure determination of epoxides is a cumbersome task for chemists, because the conformation of epoxides are difficult to assume and also its diastereoisomer has very similar structural features.1 Both diastereomeric isomers usually have similar conformation and thus it is not so easy to determine the stereochemistry by only measuring the NOESY spectrum. In such case, we sometimes use X-ray analysis, which gives us unambiguous results. We recently encountered troublesome examples of fukinone epoxide derivatives during the structure determination of natural products from Ligularia subspicata and L. lamarum.2 The search for these data resulted in vain.3-6 Mitsuhashi and co-workers described the synthetic work of bakkenolide A through fukinone epoxides.3 Although they reported the mp, the specific rotations, and NMR signals, they were not able to assign these configurations. Horinaka and Naya reported the autoxidation of fukinone and pulegone and assigned both isomers of the corresponding epoxides.5 However, none of the data was available nowadays.6 Therefore, we planned to confirm the absolute configuration of fukinone epoxides previously reported around in 1970, but yet difficult to obtain the original data. In addition to the relative configuration, it was also possible to assume the conformations, as well.
Fukinone (1) was oxidized with m-chloroperbenzoic acid in CH2Cl2 to give both kinds of epoxides 2 and 3 in the ratio of 2:1, respectively. Each product was separated by preparative HPLC. The CD spectrum of 2 showed the negative Cotton effect at 300.4 nm (Figure 1). In contrast to this result, the CD spectrum of 3 exhibited the positive Cotton effect at 309.7 nm (Figure 2). The 2D NMR spectra of both isomers were measured and the NOESY correlations of 2 was shown in Figure 1. These arrows clearly showed the A/B cis fused rings by the NOE between H-14 and H-10, H-14 and H-1β, H-15 and H-6α, H-15 and H-3β, H-4α and H-9α, and H-2α and H-9α. Because NOE between H-13 and H-6α was observed, the epoxide oxygen must be β in this case. The structure and conformation of this compound should be as indicated in Figure 1. Namely, the Me group at C-5 is axial for ring A, but equatorial for ring B (steroidal conformation). Contrary to these results, compound 3 does not adopt the conformation like 2. The NOESY spectrum of compound 3 (Figure 2) exhibited the correlations between H-15 and H-10, H-14 and H-9β, H-1α and H-6α, and H-3α and H-6α. Therefore, the Me group at C-5 must be equatorial for ring A (non-steroidal conformation). The difference in these conformations may be attributed to the steric repulsion between H-12 and the carbonyl oxygen atom. Because the NOE between H-13 and H-14 was observed, the epoxide oxygen should be α in this case. These assignments agree with the sign of the Cotton effects of these epoxides (Figures 1 and 2) predicted by the back octant rule. Reusch and Johnson reported the relative configuration of a pair of pulegone oxide isomers by measuring the spectroscopic data including CD spectra.7 They also argued the preferred conformation of these compounds. Soon after Djerassi et al. proposed a Reverse Octant Rule for endo α,β-epoxy ketone compounds. The predicted back octant is opposite to the normal rule.8 There is no systematic report on the correlation between CD spectra and the absolute configuration for exo α,β-epoxy ketone compounds. Therefore, we needed to obtain more convincing evidence.
In order to obtain more information, both epoxides were reduced with NaBH4 to give only one isomer 4 in case of β-epoxide 2. However, when α-epoxide 3 was reduced, a separable mixture of 6 and 7 was obtained, being slightly more 6. The convex face of β-epoxide 2 has no steric hindrance, while the concave face is hindered by C-11 and H-9α (Figure 1). Therefore, alcohol 4 was the sole product. However, α-epoxide 3 has the conformation as shown in Figure 2, and both sides of the carbonyl group almost equally accept the hydride attack. Alcohols 4 and 6 were alternatively obtained through reduction of fukinone 1 to alcohol 9, followed by epoxidation. Compounds 4 and 6 were separately treated with (1S)-(-)-camphanic chloride in the presence of DMAP to afford the corresponding esters, 59 and 8,10 respectively. Fortunately both esters were crystallized and the structures were revealed by the X-ray crystallographic analyses. As shown in Figure 3, both rings of ester 5 adopt the chair conformation with the α-axial hydroxy (ester) group. The conformation changed to non-steroidal, the Me group at C-5 being equatorial for ring A, due to the steric repulsion between a bulky ester group and H-12. The stereostructure for ester 8 was shown in Figure 4, being the hydroxy (ester) function α-axial. Therefore, the configurations of the epoxide oxygen atoms were unambiguously determined, and the structures of β-epoxide 2 and α-epoxide 3 were also established.
As a conclusion, these results clearly show that 2 is the β-epoxide and 3 is the α-epoxide, respectively. Their CD spectra (Figures 1 and 2) were also confirmed as predicted by the normal back octant rule. Compounds bearing exo α,β-epoxy ketones can be applied to the normal back octant rule. It is also noteworthy that both epoxides 2 and 3 adopt different conformations, while esters 5 and 8 the similar conformations, each other.
ACKNOWLEDGEMENTS
This paper is dedicated to Professor Albert Eschenmoser on the occasion of his 85th birthday. We thank Miss Y. Okamoto, Tokushima Bunri University, for measuring MS spectra.
EXPERIMENTAL
The IR spectra were measured with a SHIMADZU FT/IR-8400S spectrophotometer. The 1H, 13C, and 2D NMR spectra were taken with a Varian Unity 400MR (400 MHz) or Varian 500MR (500 MHz) spectrometer. The mass spectra including high-resolution mass spectra were taken with a JEOL JMS-700 MStation. The specific rotation was measured with a JASCO P-1030 and CD with a JASCO J-725. The X-ray analysis was performed with a Bruker AXS SMART APEX II analyzer. Chemcopak Nucleosil 50-5 was used for HPLC (JASCO pump system). Silica gel 60 F254 plates (Merck) were used for TLC.
Preparation of camphanic esters 5 and 8
Fukinone (1) (20.4 mg), isolated from several Ligularia species,11 was treated with mCPBA (77.9 mg) in CH2Cl2 at 0˚C for 1 h. The mixture was washed with Sat. NaHCO3 soln. after treatment with 1M Na2S2O3 soln. The residue was further washed with water and brine, dried (MgSO4) and was evaporated to afford a mixture of epoxides (Rf for 2 0.13, 3 0.15 (hexane:EtOAc=9:1)). Each isomer (2 and 3) was separated by HPLC to give 2 (12.2 mg) and 3 (4.8 mg). 2 (7.8 mg) was reduced with NaBH4 (4.4 mg) in MeOH-EtOAc (1:3) at 0 ˚C to give 4 (7.4 mg). 3 (4.8 mg) was also reduced to produce 6 (2.3 mg) and 7 (2.0 mg). 4 (2.9 mg) was treated with (1S)-(-)-camphanic chloride (33.3 mg) in CH2Cl2 in the presence of NEt3 and DMAP to afford 5 (4.8 mg). 6 (2.3 mg) was similarly treated to give 8 (4.0 mg).
epoxide 2: [α]D22 +22.3 (c 0.70, CHCl3); MS (CI) 236 [M]+, 219 (base), 109; HRMS (CI) Obs. m/z 236.1762 [M]+ (Calcd for C15H24O2 236.1776); FTIR 1722 cm-1; 1H NMR (500 MHz, CDCl3) δ 0.91 (3H, d, J = 6.6 Hz, H-15), 0.97 (3H, s, H-14), 1.23 (3H, s, H-12), 1.26–1.35 (1H, m, H-3a), 1.32–1.37 (1H, m, H-1a), 1.44 (3H, s, H-13), 1.52 (1H, qt, J = 13.2, 4.1 Hz, H-2a), 1.52–1.58 (1H, m, H-2b), 1.59–1.64 (1H, m, H-3b), 1.82 (1H, d, J = 14.8 Hz, H-6a), 1.81–1.89 (1H, m, H-1b), 1.85–1.91 (1H, m, H-4), 2.01 (1H, dtd, J = 14.2, 4.1, 2.7 Hz, H-10), 2.04 (1H, d, J = 14.8 Hz, H-6b), 2.24 (1H, dd, J = 14.2, 4.1 Hz, H-9a), 2.75 (1H, t, J = 14.2 Hz, H-9b); 13C NMR (125 MHz, CDCl3) δ 17.4 (C-15), 19.5 (C-12), 19.9 (C-2), 21.3 (C-13), 21.4 (C-14), 26.9 (C-1), 30.4 (C-4), 30.9 (C-3), 37.6 (C-5), 41.5 (C-6), 43.7 (C-9), 44.2 (C-10), 64.9 (C-11), 69.2 (C-7), 208.3 (C-8); CD (EtOH) [θ] -2860 (300.4 nm).
epoxide 3: [α]D22 +15.3 (c 0.35, CHCl3); MS (CI) 236 [M]+ (base), 219, 126, 109; HRMS (CI) Obs. m/z 236.1784 [M]+ (Calcd for C15H24O2 236.1776); FTIR 1720 cm-1; 1H NMR (500 MHz, CDCl3) δ 1.01 (3H, d, J = 7.1 Hz, H-15), 1.17 (3H, s, H-14), 1.22 (3H, s, H-12), 1.28–1.34 (1H, m, H-1a), 1.31–1.37 (1H, m, H-3a), 1.41 (3H, s, H-13), 1.44 (1H, d, J = 14.4 Hz, H-6a), 1.48–1.56 (2H, m, H-2), 1.51–1.58 (1H, m, H-1b), 1.55–1.61 (1H, m, H-4), 1.73–1.79 (1H, m, H-3b), 2.00–2.05 (1H, m, H-10), 2.32 (1H, dd, J = 14.9, 3.7 Hz, H-9a), 2.59 (1H, dd, J = 14.9, 5.6 Hz, H-9b), 2.61 (1H, d, J = 14.4 Hz, H-6b); 13C NMR (125 MHz, CDCl3) δ 15.1 (C-15), 19.3 (C-12), 20.3 (C-2), 20.7 (C-13), 24.4 (C-14), 28.8 (C-1, C-3), 37.0 (C-5), 37.4 (C-6), 37.5 (C-4), 38.7 (C-10), 45.1 (C-9), 64.7 (C-11), 68.9 (C-7), 207.8 (C-8); CD (EtOH) [θ] +780 (309.7 nm).
alcohol 4: [α]D22 +62.5 (c 0.74, EtOH); MS (CI) 239 [M+H]+, 221, 163, 149, 122 (base); HRMS (CI) Obs. m/z 239.2003 [M+H]+ (Calcd for C15H27O2 239.2011); FTIR 3450 cm-1; 1H NMR (500 MHz, C6D6) δ 0.74 (3H, d, J = 6.6 Hz, H-15), 0.89 (3H, s, H-14), 1.06–1.14 (1H, m, H-3a), 1.12 (1H, br d, J = 15.4 Hz, H-6a), 1.19 (3H, s, H-13), 1.30–1.40 (2H, m, H-2), 1.41–1.46 (1H, m, H-10), 1.39–1.49 (1H, m, H-4), 1.44 (3H, s, H-12), 1.42–1.50 (1H, m, H-3b), 1.43–1.57 (2H, m, H-1), 1.68 (1H, m, H-9a), 1.77 (1H, br d, J = 13.2 Hz, H-9b), 2.02 (1H, br d, J = 15.4 Hz, H-6b), 3.73 (1H, br t, J = 5.1 Hz, H-8); 13C NMR (125 MHz, C6D6) δ 17.0 (C-15), 20.6 (C-12), 21.6 (C-2), 23.0 (C-14), 23.5 (C-13), 28.7 (C-1), 31.2 (C-3), 34.5 (C-9), 35.1 (C-4), 38.0 (C-5, C-6), 39.6 (C-10), 61.9 (C-11), 67.0 (C-7), 73.2 (C-8).
ester 5: Colorless needle; mp 97.4–100.2 (from hexane); [α]D22 +33.9 (c 0.29, CHCl3); MS (CI) m/z 419 [M+H]+, 360, 221, 122 (base); HRMS (CI) Obs. m/z 419.2790 [M+H]+ (Calcd for C25H39O5 419.2798); FTIR 1790, 1747, 1730, 1269, 1169, 1099, 1059 cm-1; 1H NMR (500 MHz, CDCl3) δ 0.91 (3H, d, J = 6.8 Hz, H-15), 0.97 (3H, s, H-8'), 1.00 (3H, s, H-14), 1.08 (3H, s, H-9'), 1.12 (3H, s, H-11'), 1.15 (1H, d, J = 14.2 Hz, H-6a), 1.23–1.30 (1H, m, H-3b), 1.30 (3H, s, H-12), 1.33 (3H, s, H-13), 1.38–1.46 (2H, m, H-2), 1.45–1.52 (1H, m, H-1a), 1.46–1.53 (1H, m, H-4), 1.59–1.67 (2H, m, H-1b, 3a), 1.65–1.70 (1H, m, H-10), 1.65–1.72 (1H, m, H-5'a), 1.68–1.74 (1H, m, H-9a), 1.90–1.96 (1H, m, H-5'b), 1.96–2.02 (1H, m, H-6'a), 2.05–2.11 (1H, m, H-9b), 2.30 (1H, d, J = 14.2 Hz, H-6b), 2.41 (1H, ddd, J = 13.2, 10.5, 4.2 Hz, H-6'b), 4.97–5.01 (1H, m, H-8); 13C NMR (125 MHz, CDCl3) δ 9.7 (C-11'), 15.9 (C-15), 16.8 (C-9’), 16.9 (C-8'), 20.5 (C-12), 21.1 (C-2), 21.6 (C-13), 23.3 (C-14), 28.7 (C-1), 29.0 (C-5'), 30.0 (C-3), 30.9 (C-6'), 31.7 (C-9), 35.9 (C-6), 36.3 (C-10), 36.6 (C-5), 37.4 (C-4), 54.3 (C-7'), 54.9 (C-4'), 60.8 (C-11), 64.5 (C-7), 75.7 (C-8), 90.9 (C-1'), 166.6 (C-10'), 178.4 (C-3').
alcohol 6: [α]D22 +35.2 (c 0.92, EtOH); MS (CI) 239 [M+H]+, 221, 163, 122 (base); HRMS (CI) Obs. m/z 239.2016 [M+H]+ (Calcd for C15H27O2 239.2011); FTIR 3450 cm-1; 1H NMR (400 MHz, CDCl3) δ 0.83 (3H, d, J = 6.8 Hz, H-15), 0.87 (3H, s, H-14), 1.16 (1H, d, J = 14.6 Hz, H-6a), 1.19–1.27 (1H, m, H-3a), 1.34 (3H, s, H-13), 1.41–1.49 (2H, m, H-2), 1.45–1.53 (1H, m, H-10), 1.49 (3H, s, H-12), 1.46–1.55 (1H, m, H-3b), 1.53–1.62 (1H, m, H-1a), 1.63–1.72 (1H, m, H-1b), 1.71–1.79 (1H, m, H-9a), 1.73–1.81 (1H, m, H-4), 1.82 (1H, d, J = 6.3 Hz, OH), 1.90 (1H, ddd, J = 13.9, 10.0, 8.2 Hz, H-9b), 2.15 (1H, d, J = 14.6 Hz, H-6b), 3.83 (1H, dt, J = 8.2, 6.3 Hz, H-8); 13C NMR (100 MHz, CDCl3) δ 15.9 (C-15), 21.0 (C-2), 21.0 (C-12), 22.3 (C-14), 22.8 (C-13), 28.0 (C-1), 30.2 (C-3), 34.1 (C-4), 35.1 (C-9), 36.6 (C-5), 36.7 (C-6), 39.0 (C-10), 63.4 (C-11), 67.2 (C-7), 68.4 (C-8).
alcohol 7: MS (CI) 239 [M+H]+, 221, 163, 122 (base); HRMS (CI) Obs. m/z 239.2016 [M+H]+ (Calcd for C15H27O2 239.2011); 1H NMR (500 MHz, CDCl3) δ 0.75 (3H, d, J = 6.6 Hz, H-15), 0.90 (3H, s, H-14), 1.23 (1H, qd, J = 13.4, 4.9 Hz, H-3a), 1.23–1.28 (1H, m, H-1a), 1.34 (3H, s, H-13), 1.38 (3H, s, H-12), 1.36–1.43 (1H, m, H-3b), 1.42–1.48 (1H, m, H-9a), 1.44–1.49 (1H, m, H-2a), 1.52 (1H, qt, J = 13.4, 4.4 Hz, H-2b), 1.56 (1H, d, J = 14.9 Hz, H-6a), 1.69 (1H, d, J = 14.9 Hz, H-6b), 1.78–1.84 (1H, m, H-10), 1.88 (1H, tt, J = 13.4, 4.9 Hz, H-1b), 1.99–2.06 (1H, m, H-4), 2.35 (1H, ddd, J = 14.6, 13.7, 3.2 Hz, H-9b), 3.73 (1H, br s, H-8); 13C NMR (125 MHz, CDCl3) δ 16.1 (C-15), 19.5 (C-12), 21.0 (C-2), 21.0 (C-13), 22.0 (C-14), 26.7 (C-1), 29.9 (C-4), 30.7 (C-3), 32.2 (C-9), 33.5 (C-6), 35.7 (C-10), 37.0 (C-5), 60.1 (C-11), 65.4 (C-7), 70.1 (C-8).
ester 8: Colorless needle; mp 141.3–142.3 (from hexane); [α]D22 +19.6 (c 0.31, CHCl3); MS (CI) m/z 419 [M+H]+, 221 (base), 203, 122; HRMS (CI) Obs. m/z 419.2801 [M+H]+ (Calcd for C25H39O5 419.2798); FTIR 1788, 1747, 1263, 1171, 1107, 1061 cm-1; 1H NMR (500 MHz, CDCl3) δ 0.92 (3H, s, H-14), 0.95 (3H, d, J = 7.1 Hz, H-15), 0.99 (3H, s, H-8'), 1.06 (1H, d, J = 14.0 Hz, H-6a), 1.12 (3H, s, H-11'), 1.13 (3H, s, H-9'), 1.33 (3H, s, H-13), 1.31–1.37 (1H, m, H-3a), 1.40–1.46 (1H, m, H-1a), 1.42–1.50 (2H, m, H-2), 1.46 (3H, s, H-12), 1.50–1.55 (1H, m, H-4), 1.56–1.62 (1H, m, H-10), 1.67–1.73 (1H, m, H-5'a), 1.68–1.76 (1H, m, H-3b), 1.77 (1H, dt, J = 15.4, 3.7 Hz, H-9a), 1.81–1.88 (1H, m, H-1b), 1.91–1.97 (1H, m, H-5'b), 1.96–2.03 (1H, m, H-9b), 2.02–2.07 (1H, m, H-6'a), 2.45 (1H, ddd, J = 13.5, 10.8, 4.2 Hz, H-6'b), 2.49 (1H, d, J = 14.0 Hz, H-6b), 5.28–5.32 (1H, m, H-8); 13C NMR (125 MHz, CDCl3) δ 9.7 (C-11'), 15.4 (C-15), 16.6 (C-8'), 16.8 (C-9'), 20.8 (C-2), 21.3 (C-12), 21.7 (C-13), 23.7 (C-14), 29.1 (C-1), 29.2 (C-5'), 29.6 (C-3), 30.3 (C-6'), 32.4 (C-9), 33.7 (C-6), 35.5 (C-10), 37.2 (C-5), 38.3 (C-4), 54.3 (C-7'), 54.8 (C-4'), 64.7 (C-11), 65.1 (C-7), 72.4 (C-8), 91.3 (C-1'), 166.5 (C-10'), 178.2 (C-3').
References
1. M. Tori, K. Nakashima, M. Toyota, and Y. Asakawa, Tetrahedron Lett., 1993, 34, 3751; CrossRef M. Tori, H. Arbiyanti, Z. Taira, and Y. Asakawa, Tetrahedron Lett., 1992, 33, 4011; CrossRef Y. Asakawa, X. Lin, M. Tori, and K. Kondo, Phytochemistry, 1990, 29, 2597; CrossRef H. M. Chen, M. S. Cai, and Z. J. Jia, Phytochemistry, 1997, 45, 1441; CrossRef S. Ito, M. Sunagawa, M. Kodama, and H. Honma, J. Chem. Soc., Chem. Commun., 1971, 91. CrossRef
2. The structures of new compounds (a pair of 6-acetoxy-7,11-epoxyeremophilan-8-one) from Ligularia subspicata and L. lamarum collected in China and the diversity in these species will be reported in due course.
3. K. Hayashi, H. Nakamura, and H. Mitsuhashi, Chem. Pharm. Bull., 1973, 21, 2806.
4. In ref. 3, epoxide A had mp 64-66˚C, [α]D +24 (c 0.5 MeOH), (+)-Cotton effect, NMR (CCl4) δ 0.98 (3H, d, J = 6 Hz), 1.13 (6H, s), 1.32 (3H, s); epoxide B had mp 83-83.5 ˚C, [α]D +21 (c 0.51, MeOH), (-)-Cotton effect, NMR (CCl4) δ 0.90 (3H, d, J = 6 Hz), 0.96 (3H, s), 1.12 (3H, s), 1.35 (3H, s).
5. A. Horinaka and K, Naya, Bull. Chem. Soc. Jpn., 1979, 52, 1964. CrossRef
6. In ref. 5, epoxide 2 had mp 66-67˚C; epoxide 3 had mp 83-84 ˚C.
7. W. Reusch and C. K. Johnson, J. Org. Chem., 1963, 28, 2557; CrossRef W. Reusch and P. Mattison, Tetrahedron, 1968, 24, 4933. CrossRef
8. C. Djerassi, W. Klyne, T. Norin, G. Ohloff, and E. Klein, Tetrahedron, 1965, 21, 163; CrossRef G. Snatzke, Tetrahedron, 1965, 21, 421; CrossRef H. Wynberg and B. Marsman, J. Org. Chem., 1980, 45, 158. CrossRef
9. X-Ray crystallographic analysis of compound 5: All diagrams and calculations were performed using maXus (Bruker Nonius, Delft & MacScience, Japan). Mo Kα radiation, k = 0.71073 Å, Data collection: DIP Image plate, Program(s) used to refine structure: SHELXL-97 (Sheldrick, 1997); refinement on F2, full matrix least squares refinement. Crystal data: orthorhombic, p212121, a = 6.4711(8) Å, b = 10.6378(12) Å, c = 34.182(4) Å, α = 90˚, β=90˚, γ = 90˚, V = 2353.0(5) Å3, R = 0.0359. Crystallographic data for compound 5 have been deposited at the Cambridge Crystallographic Data Center as supplementary publication number CCDC 790131. Copies of the data can be obtained, free of charge, via www.ccdc.cam.ac.uk/data_request/cif, or by mailing to the Director, CCDC, 12 Union Road, Cambridge CB2 1EZ, UK (fax: +44 1223 336033 or e-mail: data_request@ccdc.cam.ac.uk).
10. X-Ray crystallographic analysis of compound 8: All diagrams and calculations were performed using maXus (Bruker Nonius, Delft & MacScience, Japan). Mo Kα radiation, k = 0.71073 Å, Data collection: DIP Image plate, Program(s) used to refine structure: SHELXL-97 (Sheldrick, 1997); refinement on F2, full matrix least squares refinement. Crystal data: monoclinic, p21, a = 11.603(13) Å, b = 7.146(8) Å, c = 14.644(16) Å, α = 90˚, β = 107.887(14)˚, γ = 90˚, V = 1155(2) Å3, R = 0.0784. Crystallographic data for compound 8 have been deposited at the Cambridge Crystallographic Data Center as supplementary publication number CCDC 790130. Copies of the data can be obtained, free of charge, via www.ccdc.cam.ac.uk/data_request/cif, or by mailing to the Director, CCDC, 12 Union Road, Cambridge CB2 1EZ, UK (fax: +44 1223 336033 or e-mail: data_request@ccdc.cam.ac.uk).
11. M. Tori, H. Nakamizo, K. Mihara, M. Sato, Y. Okamoto, K. Nakashima, M. Tanaka, Y. Saito, X. Gong, Y. Shen, R. Hanai, and C. Kuroda, Phytochemistry, 2008, 69, 1158; CrossRef M. Tori, A. Watanabe, S. Matsuo, Y. Okamoto, K. Tachikawa, S. Takaoka, X. Gong, C. Kuroda, and R. Hanai, Tetrahedron, 2008, 64, 4486. CrossRef