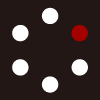
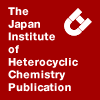
HETEROCYCLES
An International Journal for Reviews and Communications in Heterocyclic ChemistryWeb Edition ISSN: 1881-0942
Published online by The Japan Institute of Heterocyclic Chemistry
e-Journal
Full Text HTML
Received, 1st July, 2010, Accepted, 12th August, 2010, Published online, 13th August, 2010.
DOI: 10.3987/COM-10-S(E)85
■ Design and Synthesis of Telomestatin Derivatives Containing Methyl Oxazole and Their G-Quadruplex Stabilizing Activities
Satoki Majima, Masayuki Tera, Keisuke Iida, Kazuo Shin-ya, and Kazuo Nagasawa*
Division of Biotechnology and Life Science, Institute of Symbiotic Science and Technology, Tokyo University of Agriculture and Technology, 2-24-16 Nakamachi, Koganei, Tokyo 184-8588, Japan
Abstract
Telomestatin derivatives of L2A2-6M(2)OTD (3b) and L2A2-6M(4)OTD (3c), which have a macrocyclic hexaoxazole skeleton (6OTD) containing bis- and tetra-methyl oxazoles, were synthesized as a novel G-quadruplex ligand. This new class of “methyl oxazole containing” ligands was revealed to stabilize various G-quadruplex forming oligonucleotides more potently than “no methyl oxazole containing” L2A2-6OTD (3a).INTRODUCTION
Guanine-rich DNA sequences can adopt a specific secondary structure called G-quadruplexes,1 which exist in telomeric DNA sequences2 as well as in promoter regions of some oncogenes in vitro. In telomeric DNA sequences, G-quadruplex structures not only inhibit telomerase activity, but also dissociate the telomere related proteins including TRF23 and Pot14 from 3’-overhang chromosomes and thus induce certain cancer cell lines into apoptosis. On the other hand, guanine-rich base sequences in the promoter region of c-kit,5 bcl-26 and c-myc7 (involved in cell cycle and apoptosis) fold G-quadruplex structures, and suppress the transcriptional level of their mRNA. Thus, a stabilization of the G-quadruplex is recognized as one of the promising approaches for cancer treatments, and a number of small compounds for stabilizing G-quadruplex structures (G-quadruplex ligands) have been developed.8 In 2001, telomestatin (1) was reported as one of the most potent G-quadruplex stabilizing ligands by Seto and Shin-ya.9 Telomestatin (1) binds to the G-quadruplex in telomere with an end-stacking mode and shows potent telomerase inhibitory activity. Over the past decade, telomestatin (1) has widely been applied to the discovery of telomeric G-quadruplex functions.10 Recently, we have designed and synthesized macrocyclic hexaoxazoles of 6OTDs (2) as a G-quadruplex ligand.11 The 6OTD (2) has the same size and similar planarity with 1, and believed to end-stack with a G-quartet through the π-π interaction. The macrocyclic oxazole structure in 1 and 2 contributes to stacking with a G-quartet, however, the effects of characteristic “methyl oxazole” moiety in 1 (at C6 and C9) has not gained much attention. In this paper, we describe the synthesis of L2A2-6M(2)OTD (3b) and L2A2-6M(4)OTD (3c) containing bis- and tetra- “methyl oxazoles”, respectively, inspired from the skeleton of 1 (Figure 1). Furthermore, the stabilizing ability of the G-quadruplexes by these new ligands was evaluated by fluorescence resonance energy transfer (FRET) melting assays.12
RESULTS AND DISCUSSION
A novel class of 6OTD, i.e., L2A2-6M(2)OTD (3b) and L2A2-6M(4)OTD (3c), was synthesized from oxazoles 49b and 611a (Scheme 1). Deprotection of N,O-acetonide and the Boc group in 4 was employed with 4 M HCl to give amine 5. Methyl ester of 6 was hydrolyzed with lithium hydroxide to give carboxylic acid 7. Then, the amine 5 was reacted with the carboxylic acid 7 in the presence of 4-(4,6-dimethoxy-1,3,5-triazin-2-yl)-4-methylmorpholinium chloride (DMT-MM)13 and N-methylmorpholine (NMM) to give β-hydroxy amide 8 in 85% from 6. Cyclodehydration of 8 with diethylaminosulfurtrifluoride (DAST)14 followed by oxazole formation with bromotrichloromethane in the presence of 1,8-diazabicyclo[5.4.0]undec-7-ene (DBU)15 gave trioxazole 9a in 43% from 8.
Hydrolysis of the methyl ester 9a was conducted with lithium hydroxide to give carboxylic acid 10, which was subsequently reacted with amine 11b obtained from 9b11b to give 12b by using DMT-MM and NMM in 68% yield from 9a. Deprotection of the Cbz group in 12b followed by hydrolysis of methyl
methyl ester gave amino acid 13b, which was further subjected to macrocylization using diphenylphosphoryl azide (DPPA) and Eti-Pr2N in the presence of 4-dimethylaminopyridine (DMAP) under high dilution conditions (3 mM) in CH2Cl2-DMF (2:1) to give 14b in 40% yield from 12b. After conversion of the Boc group in 14b into amine, the resulting amine 15b was reacted with acetic anhydride to give L2A2-6M(2)OTD (3b) in 78% yield from 14b. A tetra-methyl oxazole derivative of L2A2-6M(4)OTD (3c) was also synthesized from 9a and 11a with a similarity to 3b via 12a, 13a, and 14a.
The G-quadruplex stabilizing ability of L2A2-6M(2)OTD (3b) and L2A2-6M(4)OTD (3c) was examined by FRET melting assays.16 In this assay, a ligand-induced stabilization of a folded G-quadruplex is evaluated by an incremental measurement of the melting temperature as ΔTm. The ΔTm values of the four kinds of (GFOs, 0.2 µM) in the presence of 3a-c at a concentration of 2 µM are summarized in Table 1. The ΔTm value of telo21 in the presence of 3a-c were 11.7, 14.0, 16.7 ºC, respectively i.e., 3c showed most potent G-quadruplex stabilizing activity among them. The other GFOs of kit22, bcl27 and myc22 showed similar tendency with these ligands. Thus, it was revealed that with a higher increment of “methyl-oxazole” moieties in macrocyclic hexaoxazole, G-quadruplex stabilizing activity was increased. Thus, incorporation of the “methyl oxazoles” into a macrocyclic skeleton in 6OTD was realized to be an effective strategy on augmenting the interaction with the G-quadruplex. Furthermore, no significant interactions between the double-stranded DNA of ds-26 and 3a-c were observed even at high concentrations (Table 1 and Figure 2). Thus, 3b and 3c are selective ligands for GFOs likewise 3a.
In conclusion, we report a synthesis of a novel G-quadruplex ligand of L2A2-6M(2)OTD (3b) and L2A2-6M(4)OTD (3c), a macrocyclic polyoxazole containing methyl oxazoles. It was revealed that the “methyl oxazole” moiety in 6M(2)OTD and 6M(4)OTD has an effect on the stabilizing activity for G-quadruplexes.
EXPERIMENTAL
General
Flash chromatography was performed on silica gel 60 (spherical size 0.040~0.100 mm; Kanto). Optical rotations were measured on a JASCO P 2200 polarimeter, using the sodium D line. 1H NMR and 13C NMR spectra were recorded on JEOL JNM-ECX 300, 400 and 500. The spectra are referenced internally according to residual solvent signals of CDCl3 (1H NMR; δ = 7.26 ppm, 13C NMR; δ = 77.0ppm), CD3OD (1H NMR; δ = 3.30 ppm, 13C NMR; δ = 49.0 ppm) DMSO-d6 (1H NMR; δ = 2.50 ppm, 13C NMR; δ = 39.5 ppm). Data for 1H NMR are recorded as follows: chemical shift (δ = ppm) multiplicity (s, singlet; d doublet; t, triplet; m, multiplet; br, broad), integration, coupling constant (Hz).
Mass spectra were recorded on JEOL JMS-T100X spectrometer with ESI-MS mode using method as solvent. All oligonucleotides purified were obtained from Sigma Genosys and dissolved in double-distilled water to be used without further purification. FRET melting assays were made with an excitation wavelength of 470-505 nm and a detection wavelength of 523-543 nm using The DNA Engine Opticon 2-Real-Time Cycler PCR detection system (Bio-Rad).
Synthesis of 5: To a solution of 4 (3.4 g, 9.9 mmol) in dioxane (100 mL) was added 12 M HCl (33 mL), and the mixture was stirred for 20 min at rt. The reaction mixture was concentrated in vacuo to give 5, which was used without further purification for the synthesis of 8.
Synthesis of 7: To a solution of 6 (3.8 g, 8.0 mmol) in THF-H2O (3:1, 80 mL) was added lithium hydroxide (662 mg, 15.8 mmol) at rt. After stirring at rt for 4 h, to the reaction mixture was added 1 M HCl. The resultant mixture was concentrated in vacuo to give 7, which was used without further purification for the synthesis of 8.
Synthesis of 8: To a solution of carboxylic acid 7 in THF-H2O (3:1, 160 mL) was added amine 5, NMM (1.75 mL, 16 mmol) and DMT-MM (3.2 g, 11.8 mmol), and the mixture was stirred at rt for 14 h. To the reaction mixture was added EtOAc, and the organics were washed with sat-NaHCO3, 1 M HCl and brine. The organic layer was dried over MgSO4, filtered and concentrated in vacuo. The residue was purified on silica gel (hexane-EtOAc = 1:2) to give peptide 8 (4.4 g, 85%, 2 steps from 6). Spectral data for 8: [α]25D -32.3 (c 1.1, CHCl3); 1H NMR (300 MHz, CDCl3) δ 8.19 (s, 1H), 7.70 (m, 1H), 7.34 (m, 5H), 5.48 (s, 2H), 5.30 (δ, J = 6.4 Hz, 1H), 5.11 (s, 2H), 4.87 (br, 1H), 4.56 (br, 2H), 3.92 (s, 3H), 3.09 (br, 2H), 2.59 (s, 3H), 1.91-1.74 (m, 4H), 1.48-1.41 (m, 13H), 1.28 (d, J = 6.4 Hz); 13C NMR (400 MHz, CDCl3) δ 163.7, 162.1, 161.3, 160.8, 156.1, 155.8, 153.9, 144.2, 136.1, 133.0, 128.4, 128.1, 79.1, 77.2, 67.7, 67.0, 52.2, 48.9, 40.0, 33.3, 29.3, 28.3, 22.4, 19.3, 11.6; HRMS (ESI, M+Na) calcd for C31H41N5O10Na 666.2751, found 666.2759.
Synthesis of 9a: To a solution of peptide 8 (4.3g, 6.7 mmol) in CH2Cl2 (150 mL) was added DAST (0.87 mL, 6.6 mmol) in the presence of Na2CO3 (698 mg, 6.6 mmol) and the reaction mixture was stirred at 0 °C under N2 for 30 min. To the reaction mixture was added H2O, and organic layer was extracted with CH2Cl2. The extracts were washed with H2O and brine, dried over MgSO4, filtered and concentrated in vacuo. The residue was purified on silica gel (hexane-EtOAc = 1:2) to give oxazoline (3.5 g, 83%). The oxazoline was dissolved in CH2Cl2 (80 mL), and BrCCl3 (1.10 mL, 11.2 mmol) and DBU (1.67mL, 11.2 mmol) was added to the solution. The resulting mixture was stirred at 0 °C for 18 h. To the reaction mixture was added 1 M HCl, and organic layer was extracted with CH2Cl2. The extracts were washed with H2O and brine, dried over MgSO4, filtered and concentrated in vacuo. The residue was purified on silica gel (hexane-EtOAc = 1:1) to give 9a (1.9 g, 43%, in 2 steps from 8). Spectral data for 9a: [α]25D -26.7 (c 1.35, CHCl3); 1H NMR (400 MHz, CDCl3) δ 8.29 (s, 1H), 7.35 (m, 5H), 5.52 (d, J = 8.3 Hz, 1H), 5.12 (s, 2H), 4.96 (br, 1H), 4.56, (br, 1H), 3.93 (s, 3H), 3.08 (br, 2H), 2.79 (s, 3H), 2.70 (s, 3H), 2.00-1.85 (m, 2H), 1.50-1.35 (m, 13H); 13C NMR (500 MHz, CDCl3) δ 162.7, 161.5, 156.6, 156.0, 155.7, 154.7, 150.9, 150.6, 143.3, 136.1, 134.1, 128.4, 128.0, 125.4, 124.5, 79.0, 67.0, 52.1, 49.2, 39.9, 33.6, 29.4, 28.3, 22.3, 11.8, 11.7; HRMS (ESI, M+Na) calcd for C31H37N5O9Na 646.2489, found 646.2469.
Synthesis of 12b: To a solution of 9a (840 mg, 1.4 mmol) in THF-H2O (3:1, 120 mL) was added lithium hydroxide (122 mg, 2.9 mmol) at rt, and the resulting mixture was stirred for 30 min. To the reaction mixture was added 1 M HCl, and resultant was concentrated in vacuo to give carboxylic acid 10, which was used without further purification. To a solution of 9b (950 mg, 1.5 mmol) in MeOH-THF (1:1, 100 mL) was added 20% Pd(OH)2/C (190 mg), and the mixture was stirred at rt for 1 h under an atmosphere of hydrogen (balloon). The reaction mixture was diluted with MeOH and filtered through a pad of celite. The organics were concentrated in vacuo to give amine 11b, which was used without further purification. To a solution of carboxylic acid 10 in THF-H2O (3:1, 100 mL) was added amine 11b, NMM (0.33 mL, 3.0 mmol) and DMT-MM (410 mg, 2.3 mmol), and the mixture was stirred at rt for 7 h. The reaction mixture was concentrated in vacuo, and the resulting mixture was extracted with EtOAc. The organic layer was washed with 1 M HCl, sat-NaHCO3, and brine, dried over MgSO4, filtered and concentrated in vacuo. The residue was purified on silica gel (hexane-EtOAc = 1:4) to give bis-trioxazole 12b (1.0 g, 68%, 3 steps). Spectral data for 12b: [α]25D +6.8 (c 1.4, CHCl3); 1H NMR (300 MHz, CDCl3) δ 8.34 (s, 2H), 8.29 (s, 1H), 8.28 (s, 1H), 7.50 (d, J = 9.2 Hz, 1H), 7.50 (m, 5H), 5.57 (br, 1H), 5.43 (m, 1H), 5.12 (s, 2H), 5.03 (br, 1H), 4.57 (br, 2H), 3.93 (s, 3H), 3.10 (br, 4H), 2.80 (s, 3H), 2.72 (s, 3H), 2.18-1.71 (m, 4H), 1.72-1.41 (m, 26H); 13C NMR (400 MHz, CDCl3) δ 165.5, 161.9, 161.5, 159.6, 156.7, 156.2, 156.0, 155.9, 155.8, 154.7, 154.4, 151.0, 150.8, 143.4, 141.6, 139.6, 139.1, 136.7, 136.0, 134.1, 130.8, 129.6, 128.4, 128.1, 125.4, 124.6, 79.1, 79.0, 67.2, 52.1, 49.3, 46.7, 40.2, 39.8, 33.6, 33.5, 29.5, 29.4, 28.3, 22.7, 22.3, 11.8, 11.8; HRMS (ESI, M+Na) calcd for C51H60N10O15Na 1075.4137, found 1075.4151.
Synthesis of 12a: To a solution of 9a (850 mg, 1.4 mmol) in THF-H2O (3:1, 100 mL) was added lithium hydroxide (120 mg, 2.8 mmol) at rt, and the resulting mixture was stirred for 1 h. To the reaction mixture was added 1 M HCl, and resultant was concentrated in vacuo to give carboxylic acid 10, which was used without further purification. To a solution of 9a (1.1 g, 1.9 mmol) in MeOH-THF (1:1, 100 mL) was added Pd(OH)2/C (190 mg), and the mixture was stirred at room temperature for 1 h under an atmosphere of hydrogen (balloon). The reaction mixture was diluted with MeOH and filtered through a pad of celite. The filtrate was concentrated in vacuo to give amine 11a, which was used without further purification. To a solution of carboxylic acid 10 in THF-H2O (3:1, 100 mL) was added amine 11a, NMM (300 µL, 2.8 mmol) and DMT-MM (575 mg, 2.1 mmol), and the mixture was stirred at rt for 17 h. The reaction mixture was concentrated in vacuo and the resulting mixture was extracted with EtOAc. The organic layer was washed with 1 M HCl and H2O, dried over MgSO4, filtered and concentrated in vacuo. The residue was purified on silica gel (CHCl3-EtOAc = 1:1) to give bis–trioxazole 12a (1.5 g, 95%, 3 steps). Spectral data for 12a: [α]25D +3.7 (c 1.8, CHCl3); 1H NMR (400 Mz, CD3Cl) δ 8.29 (s, 1H), 8.27 (s, 1H), 7.48 (d, J = 9.2 Hz, 1H), 7.34 (m, 5H), 5.52 (d, J = 8.7 Hz, 1H), 5.43 (m, 1H), 5.12 (s, 2H), 4.96 (br, 1H), 4.56 (br, 2H), 3.93 (s, 3H), 3.10 (br, 4H), 2.79 (s, 3H), 2.78 (s, 3H), 2.72 (s, 3H), 2.70 (s, 3H), 2.17-1.84 (m, 4H), 1.50-1.40 (m, 26H); 13C NMR (400 MHz, CDCl3) δ 172.1, 167.6, 162.8, 162.6, 162.1, 161.5, 160.0, 156.7, 156.0, 155.9, 155.8, 154.9, 154.7, 150.9, 150.8, 150.6, 143.4, 141.1, 136.4, 136.1, 134.1, 128.4, 128.1, 125.4, 124.6, 124.4, 79.1, 67.4, 52.1, 49.2, 48.9, 46.6, 40.1, 39.9, 33.7, 33.5, 29.6, 29.5, 28.3, 22.8, 22.4, 14.0, 11.8; HRMS (ESI, M+Na) calcd for C53H64N10O15Na 1103.4450, found 1103.4463.
Synthesis of 14b: To a solution of bis-trioxazole 12b (580 mg, 0.55 mmol) in THF-H2O (3:1, 60 mL) was added lithium hydroxide (46 mg, 1.1 mmol), and the mixture was stirred at rt for 4 h. To the reaction mixture was added 1 M HCl, and resultants were concentrated in vacuo to give carboxylic acid, which was used without further purification. To the carboxylic acid solution in MeOH-THF (1:1, 50 mL) was added 20% Pd(OH)2/C (116 mg), and resulting mixture was stirred for 3 h under hydrogen (balloon). The reaction mixture was filtered through a pad of celite and the filtrate was concentrated in vacuo to give amino acid 13b, which was used without further purification. To a solution of the crude amino acid 13b in DMF-CH2Cl2 (1:2, 180 mL) was added DMAP (134 mg, 1.1 mmol), Eti-Pr2N (187 µL, 1.1 mmol), and DPPA (237 µL, 1.1 mmol). The resulting mixture was stirred for 24 h at 90 °C under a nitrogen atmosphere. To the reaction mixture was added H2O and the organic layer was extracted with EtOAc. The extracts were washed with brine, dried over MgSO4, filtered, and concentrated in vacuo. The residue was purified on silica gel (CHCl3-EtOAc = 1:1) to give 14b (194 mg, 40%, 3 steps). Spectral data for 14b: [α]25D -5.4 (c 0.63, CHCl3); 1H NMR (400 MHz, CDCl3) δ 8.51 (m, 2H), 8.25 (s, 1H), 8.22 (s, 1H), 8.18 (s, 2H) 5.44 (m, 1H), 5.35 (m, 1H), 4.58 (br, 2H), 3.07 (br, 4H), 2.74 (s, 3H), 2.68 (s, 3H), 2.23-1.84 (m, 4H), 1.57-1.17 (m, 26H); 13C NMR (400 MHz, CDCl3) δ 164.8, 162.2, 160.0, 159.8, 156.0, 156.0, 155.9, 155.1, 154.6, 150.3, 150.1, 140.8, 140.6, 139.0, 138.3, 136.9, 136.6, 130.9, 129.6, 125.7, 124.6, 78.9, 47.7, 47.6, 40.3, 34.6, 31.9, 31.5, 29.6, 29.5, 28.4, 22.6, 21.9, 14.1, 11.8; HRMS (ESI, M+Na) calcd for C42H50N10O12Na 909.3507, found 909.3492.
Synthesis of 14a: To a solution of bis-trioxazole 12a (1.5 g, 1.33 mmol) in THF-H2O (3:1, 60 mL) was added lithium hydroxide (110 mg, 2.66 mmol), and the mixture was stirred at rt for 1 h. To the reaction mixture was added 1 M HCl, and resulting mixture was concentrated in vacuo to give carboxylic acid, which was used without further purification. To the carboxylic acid solution in MeOH-THF (1:1, 60 mL) was added Pd(OH)2/C (250 mg), and resulting mixture was stirred for 2 h under a hydrogen atmosphere (balloon). The reaction mixture was filtered through a pad of celite and the filtrates were concentrated in vacuo to give amino acid 13a. To a solution of the amino acid 13a in DMF-CH2Cl2 (1:2, 400 mL) was added DMAP (650 mg, 5.32 mmol), Eti-Pr2N (452 µL, 2.66 mmol), and DPPA (1.15 mL, 5.32 mmol), and the resulting mixture was stirred at 90 °C for 3 h under a nitrogen atomosphere. To the reaction mixture was added H2O and the organic layer was extracted with EtOAc. The extracts were washed with brine, dried over MgSO4, filtered, and concentrated in vacuo. The residue was purified on silica gel (CHCl3-EtOAc = 3:1) to give 14a (506 mg, 0.55 mmol, 41%, 3 steps). Spectral data for 14a: [α]25D +4.3 (c 2.1, CHCl3); 1H NMR (400 MHz, CDCl3) δ 8.44 (d, J = 7.5 Hz, 2H), 8.22 (s, 2H), 5.34 (m, 2H), 4.61 (br, 2H), 3.05 (m, 4H), 2.71 (s, 6H), 2.65 (s, 6H), 2.06 (m, 2H), 1.91 (m, 2H), 1.53-1.19 (m, 26H); 13C NMR (400 MHz, CDCl3) δ 162.2, 160.0, 155.9, 155.0, 150.1, 149.9, 140.5, 136.6, 125.7, 124.6, 78.9, 47.4, 40.3, 34.6, 29.5, 28.3, 21.9, 11.7; HRMS (ESI, M+Na) calcd for C44H54N10O12Na 937.3820, found 937.3825.
Synthesis of 15b: To a solution of 14b (130 mg, 0.146 mmol) in THF-MeOH (1:1, 20 mL) was added 12 M HCl (4 mL), and the mixture was stirred for 10 min at rt. The reaction mixture was concentrated in vacuo to give 15b (95 mg, 86%). Spectral data for 15b: [α]25D -34.6 (c 2.6, MeOH); 1H NMR (400 MHz, DMSO-d6) δ 9.13 (s, 1H), 9.11 (s, 1H), 8.92 (s, 1H), 8.89 (s, 1H), 8.31 (d, J = 7.3, 1H), 8.28 (d, J = 7.3, 1H), 7.78 (br 4H), 5.45 (m, 1H), 5.35 (m, 1H), 2.75 (s, 3H), 2.71 (s, 3H), 2.07 (m, 2H), 1.92 (m, 2H), 1.65-0.98 (m, 8H); 13C NMR (400 MHz, DMSO-d6 ) δ 164.4, 162.0, 158.9, 158.7, 158.1, 157.8, 155.6, 155.6, 154.5, 154.5, 154.4, 151.4, 151.1, 142.5, 142.3, 141.8, 141.1, 136.0, 135.9, 129.7, 128.5, 124.7, 123.7, 69.8, 47.2, 47.1, 33.3, 26.7, 20.9, 11.5; HRMS (ESI, M+Na) calcd for C32H35N10O8Na 687.2639, found 687.2601.
Synthesis of 15a: To a solution of 14a (270 mg, 0.295 mmol) in THF-MeOH (1:1, 10 mL) was added 12 M HCl (2 mL), and the mixture was stirred for 2 h at rt. The reaction mixture was concentrated in vacuo to give 15a (200 mg, 86%). Spectral data for 15a: [α]25D +38.9 (c 0.5, MeOH); 1H NMR (400 MHz, DMSO-d6) δ 8.89 (s, 2H), 8.24 (d, J = 6.9, 2H), 7.82 (br, 4H), 5.36 (br, 2H), 3.16 (br, 4H), 2.75 (br, 6H), 2.71 (br, 6H), 2.05 (br, 2H), 1.91 (br, 2H), 1.68-0.58 (m, 8H); 13C NMR (400 MHz, DMSO-d6) δ 162.0, 158.9, 158.1, 157.8, 155.6, 154.4, 151.3, 151.1, 142.3, 135.8, 124.7, 123.7, 48.6, 46.9, 45.6, 33.4, 26.7, 20.9, 11.5, 8.9; HRMS (ESI, M+Na) calcd for C34H39N10O8 715.2952, found 715.2962.
Synthesis of L2A2-6M(2)OTD (3b): To a solution of 15b (36 mg, 0.047 mmol) in acetic anhydride (4 mL) was added pyridine (1 mL), and the mixture was stirred at 40 ºC for 15 h. The reaction mixture was concentrated in vacuo. The residue was purified on silica gel (EtOAc-MeOH = 4:1) to give L2A2-6M(2)OTD (3b) (33 mg, 91%). Spectral data for 3b: [α]25D +30.9 (c 1.5, MeOH); 1H NMR (400 MHz, DMSO-d6) δ 9.11 (s, 1H), 9.08 (s, 1H), 8.89 (s, 1H), 8.86 (s, 1H), 8.35 (d, J = 7.5 Hz, 1H), 8.31 (d, J = 7.5, 1H), 7.82 (br, 2H), 5.41 (dd, J = 5.7, 12.6 Hz, 1H), 5.32 (dd, J = 5.2, 12.6 Hz, 1H), 2.94 (br, 4H), 2.74 (s, 3H), 2.70 (s, 3H), 2.02 (br, 2H), 1.89 (br, 2H), 1.71 (s, 6H), 1.35-1.12 (m, 8H); 13C NMR (400 MHz, DMSO-d6); δ 170.3, 168.9, 164.6, 162.2, 158.9, 158.7, 155.7, 155.5, 154.5, 154,4, 151.3, 151.0, 142,5, 142,2, 141,7, 141,0, 136,0, 135,8, 129,7, 128,3, 124,6, 123,6, 69.8, 59.7, 47.3, 47,1, 38.3, 33,4, 28,7, 22,5, 21,4, 21,3, 20,7, 14,1, 11,5; HRMS (ESI, M+Na) calcd for C36H38N10O10Na 793.2670, found 793.2687.
Synthesis of L2A2-6M(4)OTD (3c): To a solution of 15a (16 mg, 0.022 mmol) in acetic anhydride (3 mL) was stirred at 40 °C for 7 h. The reaction mixture was concentrated in vacuo and the residue was purified on silica gel (EtOAc-MeOH = 4:1) to give L2A2-6M(4)OTD (3c) (17 mg, 97%). Spectral data for 3c: [α]25D +7.0 (c 0.5, MeOH); 1H NMR (300 MHz, CDCl3) δ 8.90 (s, 1H), 8.87 (s, 1H), 8.26 (br, 2H), 7.86 (br, 2H), 5.34 (br, 2H), 2.95 (br 4H), 2.73 (s, 6H), 2.69 (s, 6H), 2.02 (br, 2H), 1.89 (br, 2H), 1.73 (s, 6H), 1.36-1.10 (m, 8H); 13C NMR (300 MHz, CDCl3) δ 170.5, 162.7, 160.1, 156.0, 155.2, 150.3, 150.2, 140.7, 136.7, 125.5, 124.4, 47.2, 39.3, 34.2, 29.7, 28.6, 23.2, 21.8, 11.8, 11.7; HRMS (ESI, M+Na) calcd for C38H42N10O10Na 821.2983, found 821.2982.
FRET melting assays: FRET melting assays were performed as previously reported methods.12 The dual fluorescently labeled oligonucleotides were used in this protocol. The donor fluorophore was 6-carboxyfluorescein, FAM, and the acceptor fluorophore was 6-carboxytetramethylrhodamine, TAMRA. The oligonucleotides were initially dissolved as a 100 µM stock solution in MilliQ water; further dilutions were carried out in 60 mM potassium cacodylate buffer (pH 7.4). Dual-labeled DNA was denatured at a concentration of 0.4 µM by heating at 94 ºC for 5 min followed by cooling to rt to anneal. We added the various concentrations of ligands (3a-c) into different samples, using a total reaction volume of 40 µL, with 0.2 µM of labelled oligonucleotide. Following experiments should keep the temperature-procedure in real-time PCR and the procedure was finished as following: 25 °C for 10 minutes, then a stepwise increase of 1 °C every minute from 25 °C to reach 99 °C. During the procedures, the fluorescence of FAM was measured after each the temperature.
ACKNOWLEDGEMENTS
This work was supported in part by a Grant-in-Aid for Exploratory Research (21655060) and a grant under the Industrial Technology Research Grant Program (01A04006b) from the New Energy and Industrial Technology Development Organization (NEDO) of Japan. Dr. M.Tera and K. Iida are grateful for a JSPS Research Fellowship for Young Scientists.
References
1. For selected reviews see: (a) D. J. Patel, A. T. Phan, and V. Kuryavyi, Nucleic Acids Research, 2007, 35, 7429; CrossRef (b). S. Neidle and G. N. Parkinson, Biochimie, 2008, 90, 1184; CrossRef (c). Y. Danzhou and K. Okamoto, Future Med. Chem., 2010, 2, 619.
2. Intramolecular telomeric G-quadruplex in sodium solution, see: (a) Y. Wang, and D. J. Patel, Structure, 1993, 1, 263; CrossRef Intramolecular telomeric G-quadruplex in potassium solution, see: (b) Y. Xu, Y. Noguchi, and H. Sugiyama, Bioorg. Med. Chem., 2006, 14, 5584; CrossRef (c) A. Ambrus, D. Chen, J. Dai, T. Bialis, R. A. Jones, and D. Yang, Nucleic Acids Res., 2006, 34, 2723; CrossRef (d) K. N. Luu, A. T. Phan, V. Kuryavyi, L. Lacroix, and D. J. Patel, J. Am. Chem. Soc., 2006, 128, 9963; CrossRef (e) A. T. Phan, V. Kuryavyi, K. N. Luu, and D. J. Patel, Nucleic Acids Res., 2007, 35, 6517; CrossRef Crystal structure of intramolecular telomeric G-quadruplex in the presence of potassium cation, see: (f) G. N. Parkinson, M. P. Lee, and S. Neidle, Nature, 2002, 417, 876. CrossRef
3. H. Tahara, K. Shin-ya, H. Seimiya, H. Yamada, T. Tsuruo, and T. Ide, Oncogene, 2006, 25, 1955. CrossRef
4. D. Gomez, T. Wenner,B. Brassart, C. Douarre, M.-F. O’Donohue, V. E. Khoury,K. Shin-ya, H. Morjani, C. Trantesaux, and J. F. Riou, J. Biol. Chem., 2006, 281, 38721. CrossRef
5. G-Quadruplex structure of c-kit promoter region, see: (a) S. Rankin, A. P. Reszka, J. Huppert, M. Zloh, G. N. Parkinson, A. K. Todd, S. Ladame, S. Balasubramanian, and S. Neidle, J. Am. Chem. Soc., 2005, 127, 10584; CrossRef For c-kit transcription, see: (b) M. Bejugam, S. Sewitz, P. S. Shirude, R. Rodriguez, R. Shahid, and S. Balasubramanian, J. Am. Chem. Soc., 2007, 129, 12926. CrossRef
6. G-Quadruplex structure of bcl-2 promoter region, see: (a) J. Dai, T. S. Dexheimer, D. Chen, M. Carver, A. Ambrus, R. A. Jones, and D. Yang, J. Am. Chem. Soc., 2006, 128, 1096; CrossRef (b) For bcl-2 transcription, see: X.-D. Wang, T.-M. Ou, Y.-J. Lu, Z. Li, Z. Xu, C. Xi, J.-H. Tan, S.-L. Huang, L.-K. An, D. Li, L.-Q. Gu, and Z.-S. Huang, J. Med. Chem., 2010, 53, 4390. CrossRef
7. G-quadruplex structure of c-myc promoter region, see: (a) T. Simonsson, P. Pecinka1, and M. Kubista, Nucleic Acids Res., 1998, 26, 1167; CrossRef For c-myc transcription, see: (b) A. Siddiqui-Jain, C. L. Grand, D. J. Bearss, and L. H. Hurley, Proc. Natl. Acad. Sci. USA, 2002, 99, 11593. CrossRef
8. For selected reviews see: (a) A. Arora, N. Kumar, T. Agarwal, and S. Maiti, FEBS Journal, 2010, 277, 1345; CrossRef (b) T. Ou, Y. Lu, J. Tan, Z. Huang, K.-Y. Wong, and L. Gu, ChemMedChem, 2008, 3, 690. CrossRef
9. Isolation of telomestatin, see: (a) K. Shin-ya, K. Wierzba, K. Matsuo, T. Ohtani, Y. Yamada, K. Furihata, Y. Hayakawa, and H. Seto, J. Am. Chem. Soc., 2001, 123, 1262; CrossRef Total synthesis of telomestatin, see: (b) T. Doi, M. Yoshida, K. Shin-ya, and T. Takahashi, Org. Lett., 2006, 8, 4165. CrossRef
10. Selected reports of telomestatin as G-quadruplex ligands, see: (a) M.-Y. Kim, H. Vankayalapati, K. Shin-ya, K. Wierzba, and L. H. Hurley, J. Am. Chem. Soc., 2002, 124, 2098; CrossRef (b) F. Rosu, V. Gabelica, K. Shin-ya, and E. D. Pauw, Chem. Commun., 2003, 2702; CrossRef (c) E. M. Rezler, J. Seenisamy, S. Bashyam, M.-Y. Kim, E. White, W. D. Wilson, and L. H. Hurley, J. Am. Chem. Soc., 2005, 127, 9439; CrossRef (d) P. Shirude, E. Gillies, S. Ladame, F. Godde, K. Shin-Ya, I. Huc, and S. Balasubramanian, J. Am. Chem. Soc., 2007, 129, 11890. CrossRef
11. (a) M. Tera, Y. Sohtome, H. Ishizuka, T. Doi, M. Takagi, K. Shin-ya, and K. Nagasawa, Heterocycles, 2006, 69, 505; CrossRef (b) M. Tera, H. Ishizuka, M. Takagi, M. Suganuma, K. Shin-ya, and K. Nagasawa, Angew. Chem. Int. Ed., 2008, 47, 5557; CrossRef (c) M. Tera, K. Iida, H. Ishizuka, M. Takagi, M. Suganuma, T. Doi K. Shin-ya, and K. Nagasawa, ChemBioChem, 2009, 10, 431; CrossRef (d) K. Iida, M. Tera, T. Hirokawa, K. Shin-ya, and K. Nagasawa, Chem. Commun., 2009, 6481; CrossRef (e) K. Iida, M. Tera, T. Hirokawa, K. Shin-ya, and K. Nagasawa, J. Nucleic Acids, 2010, in press; (f) M. Tera, K. Iida, K. Ikebukuro, H. Seimiya, K. Shin-ya, and K. Nagasawa, Org. Biomol. Chem., 2010, 8, 2749. CrossRef
12. (a) A. D. Cian, L. Guittat, M. Kaiser, B. Sacca, S. Amrane, A. Bourdoncle, P. Alberti, M. P. Teulade-Fichou, L. Lacroix, and J. L. Mergny, Methods, 2007, 42, 183; CrossRef (b) J. L. Mergny and J.-C. Maurizot, ChemBioChem, 2001, 2, 124. CrossRef
13. M. Kunishima, C. Kawachi, F. Iwasaki, K. Terao, and S. Tani, Tetrahedron Lett., 1999, 40, 5327. CrossRef
14. (a) G. Burrell, J. M. Evans, G. E. Jones, and G. Stemp, Tetrahedron Lett., 1990, 31, 3649; CrossRef (b) A. J. Phillips, Y. Uto, P. Wipf, M. J. Reno, and D. R. Williams, Org. Lett., 2000, 2, 1165. CrossRef
15. D. R. Williams, D. J. Lowder, Y. G. Gu, and D. A. Brooks, Tetrahedron Lett., 1997, 38, 331. CrossRef
16. In FRET melting assays, four representative single stranded G-quadruplex forming oligonucleotides (GFOs), i.e., telo21, kit22, bcl27 and myc22 conjugated with dyes at both 5’ and 3’ ends were used in the presence of potassium cation. GFOs and double-stranded oligonucleotides used in this paper are as follows.