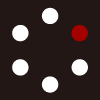
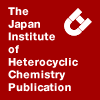
HETEROCYCLES
An International Journal for Reviews and Communications in Heterocyclic ChemistryWeb Edition ISSN: 1881-0942
Published online by The Japan Institute of Heterocyclic Chemistry
e-Journal
Full Text HTML
Received, 14th June, 2010, Accepted, 21st July, 2010, Published online, 22nd July, 2010.
DOI: 10.3987/COM-10-S(E)57
■ Simple Deuterium Introduction at α-Position of Carbonyl in Diazirinyl Derivatives for Photoaffinity Labeling
Yuta Murai, Miho Takahashi, Yasuyuki Muto, Yasumaru Hatanaka, and Makoto Hashimoto*
Graduate School of Agriculture, Hokkaido University, Kita 9 Nishi 9, Kita-ku, Sapporo, Hokkaido 060-0589, Japan
Abstract
Post-functional deuterium incorporation into diazirinyl acetophenone derivatives is reported. Treatment of the compounds with sodium hydroxide in CH3OD at rt for 10 min achieved deuterium incorporation at α-position of the carbonyl group without decomposition of diazirinyl ring. Further deuterium can introduce by reduction of benzyl carbonyl with triethylsilane-d-TFA. Straightforward deuterium introduction into diazirinyl derivatives may contribute to the functional analysis of low molecular compounds with diazirine-based photoaffinity labeling.Photoaffinity labeling is a powerful method in the study of biological structures and functions, and the selection of photophores is very important for successful photoaffinity labeling experiments. (3-Trifluoromethyl)phenyldiazirine (TPD), which is a precursor of carbene, is one of the most reliable photophores for photoaffinity labeling owing to the chemical stability of the precursor and high reactivity of the generated carbene.1 A tag for the detection and purification of photolabeled components has to be introduced into the ligand derivatives, and MS spectrometry will be useful to identify photolabeled components. Elucidation of the different mass numbers derived from a mixture of unlabeled and stableisotope-labeled photophores may be useful.2 Several protocols for the synthesis of a stableisotope-labeled TPD photophore have been reported.3, 4 Construction of the diazirinyl skeleton involves at least 5 step reactions from the corresponding halobenzene derivatives.5 Addition of a stable isotope after construction of the phenyl diazirinyl ring (post-functional) is the recommended synthetic strategy. We describe the effective synthesis of deuterium-labeled TPD compounds with H/D exchange at α-position of carbonyl compounds.
Deuterium labeling of organic compounds can be achieved by means of syntheses starting from suitable isotope marker precursors or via isotope exchange reactions. The latter approach appears to be more valuable since the deuterium can possibly be introduced post-functionally. H/D exchange reactions have been reported under alkaline6 or acidic7 conditions in protic polar solvents, such as CH3OD and D2O, and substitution of the functional group with a deuterium source and alkaline conditions in aprotic solvent such as CDCl3.8 Although easier H/D exchange was achieved under alkaline conditions in protic media, in the reported conditions, the reaction mixture should be refluxed for a long time, which seems to be a harsh condition for the diazirinyl moiety. The development of milder conditions for the H/D exchange is therefore required. In our strategy, the substrate was treated with sodium hydroxide in CD3OD at rt for a short time to make the reaction mixture homogeneous. Screening condition was performed with 2’-methoxy propiophenone 1 as the model compound (Scheme 1). Compound 1 was used at < 0.1 M in 1M NaOH in CD3OD and the rm was stirred at rt for 10 min. The rm was partitioned between 1M HCl-D2O and CDCl3. The incorporation of deuterium was estimated as over 94% by 1H-NMR in compound 1-d2 (Table 1, entry 1). There was no decrease in deuterium incorporation between before and after work up.
Compound 1 was reacted with CH3OD for the reaction and 1M HCl-H2O for work up to establish a less expensive method, and no differences in deuterium incorporation were observed (Table 1, entry 2). The selection of the organic solvent did not cause serious decrease of deuterium incorporation in 1-d2 (Table 1, entry 3~5). The reverse D/H exchange reaction also proceeded with CH3OH to deuterium at α-position of compound 1-d2 (Scheme 1).
The established reaction condition was applied to diazirinyl acetophenone derivatives 29 and deuterium incorporation was calculated as 93%. UV spectrum and 19F-NMR indicated that the diazirinyl ring did not decompose during the reaction. The deuterated derivative 2 was subjected to the reduction of benzoyl carbonyl moiety under Et3SiH – CF3COOH conditions, which did not affect the diazirinyl moiety9, and afforded trideuterated diazirinyl ethylbenzene derivative 3-d3. No decrease of deuterium incorporation was observed during the reduction. Pentadeuterated diazirinyl ethylbenzene derivatives can also be prepared under Et3SiD – CF3COOH conditions3 and incorporation was calculated as 95% and 93% at methylene and methyl of 3-d5, respectively.
acetophenone moiety was replaced with D (93%). 1H-NMR indicated neither incorporation at α-hydrogen against the ester moiety or hydrolysis of ester. Deuterium exchange at the ester α-position was observed with a long reaction time (> 1h), but incorporation of deuterium was low (<20%). Ester hydrolysis was observed when over 50 eq NaOH was used in 10 min. No deuterium incorporation at the α-position of the ester group was observed with the same treatment of methyl myristate. The results were consistent with a previous report on selective deuterium incorporation at the α-position of ketone in γ-ketoester under aqueous alkaline conditions.10 The D-incorporated skeleton derivative 4-d2 subjected to reduction of the benzyl carbonyl moiety under Et3SiD – CF3COOH conditions afforded tetradeuterium-incorporated diazirinyl fatty acid methyl esters 5-d4. The methyl ester 5-d4 was hydrolyzed in aqueous NaOH and methanol for 2 h to afford diazirinyl fatty acid derivatives 6-d4 with moderate yield without loss of deuterium. H/D exchange at α-hydrogen of diazirinyl acetophenone derivative was a very cheap and effective method to prepare diazirinyl deuterium-labeled derivatives and was useful to handle the photolabeled components with mass spectrometry analysis.
EXPERIMENTAL
1H- 13C- and 19F- NMR spectra were measured by JEOL ECA-500 spectrometers. ESI-MS spectra were obtained using LCT Primier XE (Waters). CD3OD (99.5% D) and CH3OD (99% D) were purchased from Cambridge Isotope Laboratories (Andover, USA) and Et3SiD (97% D) was purchased from Aldrich (St. Louis, USA). Deuterium incorporation was calculated with the integration of α-proton against methoxy proton as an internal standard.
H-D exchange at α-position of 2'-methoxypropiophenone (1 and 1-d2)
2'-Methoxypropiophenone 1 (0.0111 g, 0.068 mmol) was dissolved in 1M NaOH-CH3OD (1 mL). The reaction mixture was stirred at rt for 10 min, and then partitioned between CHCl3 and 1M HCl in H2O. The organic layer was washed with saturated aqueous NaCl, dried over MgSO4, filtrated and concentrated. The residue was purified with silica-column chromatography to afford a colorless oil (0.0102g, 0.061 mmol, 90%). The analytical data were identical to the literature.11 Deuterium incorporation was estimated as 95% and 94% before and after work up, respectively by 1H-NMR. The 2, 2-dideuterated analogue (1-d2) was converted to compound 1 with 1M NaOH - MeOH as described above. 1H-NMR measurement indicated that deuterium at the methyl position was converted to hydrogen at 97%.
1-(2-Methoxy-4-(3-(trifluoromethyl)-3H-diazirin-3-yl)phenyl)ethanone-2, 2, 2-d3 (2-d3)
H/D exchange reaction for compound 2 (0.0121 g, 0.047 mmol) was treated as described above to afford a colorless oil (0.0099 g, 82%). 1H-NMR (CDCl3) δ: 7.72 (1H, d, J = 8.3 Hz), 6.80 (1H, d, J = 8.3 Hz), 6.66 (1H, s), 3.90 (3H, s), 2.58 (0.21 H, m, D incorporation 93%), 13C -NMR (CDCl3) δ: 198.8, 160.5, 135.5, 132.0, 130.5, 123.4 (q, 1JCF = 274 Hz), 119.6, 110.9, 56.8, 31.0 (m), 29.6 (q, 2JCF = 40 Hz), 19F-NMR (CDCl3) δ: -66.5, ESI-MS m/z (MH+) 262 (d3, 100%), 261 (d2, 5%).
3-[4-(2, 2, 2-d3)-Ethyl-3-methoxyphenyl]-3-(trifluoromethyl)-3H-diazirine (3-d3)
Reduction was carried out as described in a previous report.9 Compound 2-d3 (0.0114 g, 0.044 mmol) was dissolved in TFA (0.2 ml). Triethylsilane (0.020 mL, 0.125 mmol) was added to the TFA solution at rt. After 10 min, the reaction mixture was subjected to work up to afford 3-d3 as a colorless oil (0.0087 g, 79%).
1H-NMR (CDCl3) δ: 7.15 (1H, d, J = 7.9 Hz), 6.74 (1H, d, J = 7.9 Hz), 6.57 (1H, s), 3.82 (3H, s), 2.62 (3H, br s), 1.14 (0.22 H, m, D incorporation 93%), 13C -NMR (CDCl3) δ: 157.5, 134.7, 129.2, 127.6, 122.2 (q, 1JCF = 274 Hz), 118.8, 107.8, 55.3, 28.5 (q, 2JCF = 40 Hz), 23.0, 13.5 (m), ESI-MS m/z (MH+) 248 (d3, 100%), 247 (d2, 5%).
3-[4-(1, 1, 2, 2, 2-d5)-Ethyl-3-methoxyphenyl]-3-(trifluoromethyl)-3H-diazirine (3-d5)
Reduction was performed with triethylsilane-D (0.0181 mL, 0.113 mmol) instead of triethylsilane3 for compound 2-d3 (0.0092 g, 0.037 mmol). The reaction mixture was treated as described above to afford 3-d5 as a colorless oil (0.0077 g, 83%). 1H-NMR (CDCl3) δ: 7.15 (1H, d, J = 7.9 Hz), 6.74 (1H, d, J = 7.9 Hz), 6.57 (1H, s), 3.82 (3H, s), 2.62 (0.10H, m, D incorporation 95%), 1.14 (0.14H, m, D incorporation 93%), 13C -NMR (CDCl3) δ: 157.5, 134.7, 129.2, 127.6, 122.2 (q, 1JCF = 274 Hz), 118.8, 107.8, 55.3, 28.5 (q, 2JCF = 40 Hz), 23.0(m), 13.5 (m), ESI-MS m/z (MH+) 250 (d5, 100%), 249 (d4, 10%).
2-Methoxy-ι-oxo-4-[3-(trifluoromethyl)-3H-diazirin-3-yl]benzenedecanoic acid methyl ester-9, 9-d2 (4-d2)
The H/D exchange reaction for compound 4 (0.0084 g, 0.02 mmol) was treated as described above to afford 4-d2 (0.0069 g, 85%). 1H-NMR (CDCl3) δ: 7.62 (1H, d, J = 7.9 Hz), 6.80 (1H, d, J = 7.9 Hz), 6.64 (1H, s), 3.88 (3H, s), 3.64 (3H, s), 2.89 (0.1H, m, D incorporation 95%), 2.27 (2H, t, J = 7.3 Hz), 1.65-1.55 (m, 4H), 1.27 (m, 8H). 13C -NMR (CDCl3) δ: 199.9, 174.3, 158.2, 133.9, 130.7, 129.7, 122.0 (q, 1JCF = 274 Hz), 118.8, 109.3, 55.7, 51.5, 39.0 (m), 34.0, 29.3, 29.2, 29.1, 29.0, 28.5 (q, 2JCF = 40 Hz), 25.0, 24.1, ESI-MS m/z (MH+) 417 (d2, 100%), 416 (d1, 7%).
2-Methoxy-4-[3-(trifluoromethyl)-3H-diazirin-3-yl]benzenedecanoic acid methyl ester-9, 9, 10, 10-d4 (5-d4)
To a stirred solution of 4-d2 (0.0114 g, 0.027 mmol) in trifluoroacetic acid (0.10 mL) at rt was added triethylsilane-D (0.12 mL, 0.75 mmol). The reaction mixture was stirred at rt for 10 min, and then worked up as described in a previous report3 to obtain a pale yellow oil (0.0086 g, 79%). 1H-NMR (CDCl3) δ: 7.12 (1H, d, J = 7.9 Hz), 6.72 (1H, d, J = 7.9 Hz), 6.57 (1H, s), 3.81 (3H, s), 3.66 (3H, s), 2.57 (0.04H, m, D incorporation 98%), 2.29 (2H, t, J = 7.6 Hz), 1.67 (m, 2H), 1.60 (0.11H, D incorporation 95%), 1.27 (m, 10H), 13C -NMR (CDCl3) δ: 174.4, 157.6, 133.4, 130.1, 127.5, 122.1 (q, 1JCF = 274 Hz), 118.6, 107.9, 55.3, 51.5, 35.8 (m), 34.1, 31.6 (m), 29.3, 29.2, 29.1, 29.0, 28.8, 28.5 (q, 2JCF = 40 Hz), 24.8, ESI-MS m/z (MH+) 405 (d4, 100%), 404 (d3, 12%).
2-Methoxy-4-[3-(trifluoromethyl)-3H-diazirin-3-yl]benzenedecanoic acid-9, 9, 10, 10-d4 (6-d4)
Methyl ester 5-d4 (0.0064 g, 0.016 mmol) was dissolved in MeOH (0.5 mL), and aqueous sodium hydroxide solution (1M, 0.1 mL) was added. The reaction mixture was stirred at rt for 2 h and then worked up as described in a previous report7 to obtain a pale yellow oil (0.0055 g, 88%). 1H-NMR (CDCl3) δ: 7.12 (1H, d, J = 7.6 Hz), 6.72 (1H, d, J= 7.6 Hz), 6.56 (1H, s), 3.81 (3H, s), 2.57 (0.04H, m, D incorporation 98%), 2.34 (2H, t, J = 7.6 Hz), 1.60 (0.11H, D incorporation 95%), 1.55 (m, 2H), 1.27 (m, 10H), 13C -NMR (CDCl3) δ: 176.0, 157.6, 133.5, 130.2, 127.6, 122.0 (q, 1JCF = 274 Hz), 118.8, 108.0, 56.2, 36.6 (m), 34.1, 31.6 (m), 29.4, 29.3, 29.2, 29.1, 28.6, 28.5 (q, 2JCF = 40 Hz), 24.8, ESI-MS m/z (MH+) 391 (d4, 100%), 390 (d3, 12%).
ACKNOWLEDGEMENTS
This research was partially supported by a Ministry of Education, Science, Sports and Culture Grant-in-Aid for Scientific Research in a Priority Area, 18032007, for Scientific Research in Innovative Areas, 20200038 and for Scientific Research (C), 19510210, 21510219 (M.H.)
References
1. M. Hashimoto and Y. Hatanaka, Eur. J. Org. Chem., 2008, 2513. CrossRef
2. S. M. Lamos, C. J. Krusemark, C. J. Mcgee, M. Scalf, L. M. Smith, and P. J. Belshaw, Angew. Chem. Int. Ed., 2006, 45,4329. CrossRef
3. M. Hashimoto and Y. Hatanaka, Chem. Pharm. Bull., 2004, 52, 1385. CrossRef
4. M. Hashimoto, T. Komano, K. Nabeta, and Y. Hatanaka, Chem. Pharm. Bull., 2005, 53, 140; CrossRef M. Hashimoto, Y. Kato, and Y. Hatanaka, Chem. Pharm. Bull., 2007, 55, 1540. CrossRef
5. Y. Hatanaka, M. Hashimoto, H. Kurihara, H. Nakayama, and Y. Kanaoka, J. Org. Chem., 1994, 59, 383; CrossRef Y. Hatanaka, H. Nakayama, and Y. Kanaoka, Rev. Heteroatom Chem., 1996, 14, 213.
6. C. A. Cope and B. M. Gale, J. Am. Chem. Soc., 1963, 85, 3747; CrossRef W. Carpenter, A. M. Duffied, and C. Djerassi, J. Am. Chem. Soc., 1968, 90, 160; CrossRef A. R. Lepley and W. A. Khan, J. Org. Chem., 1968, 33, 4362; CrossRef M. S. Mbarak and D. G. Peters, J. Org. Chem., 1982, 47, 3397; CrossRef C. H. Arrowsmith and A. J. Kresge, J. Am. Chem. Soc., 1986, 108, 782; C. H. Arrowsmith and A. J. Kresge, J. Am. Chem. Soc., 1986, 108, 7918; CrossRef C. Kunick and P. Messinger, Chem. Ber., 1986, 119, 1429; CrossRef T. Kusumoto, K. I. Sato, G. Kumaraswamy, T. Himaya, T. Isozaski, and Y. Suzuki, Chem. Lett., 1995, 1147; CrossRef C. Berthelette and J. Scheigetz, J. Labelled Compd. Radiopharm., 2004, 47, 891; CrossRef X. He, J. J. Morris, B. C. Noll, S. N. Brown, and K. W. Henderson, J. Am. Chem. Soc., 2006, 128, 13599. CrossRef
7. K. Fodor -Csorba G. Galli, S. Holly, and E. Gacs-Baitz, Tetrahedron Lett., 2002, 43, 3789. CrossRef
8. C. Sabot, K. A. Kumar, C. Antheaume, and C. Mioskowski, J. Org. Chem., 2007, 72, 5001. CrossRef
9. M. Hashimoto, Y. Hatanaka, and K. Nabeta, Heterocycles, 2003, 59, 395. CrossRef
10. H. E. Zimmerman, J. H. Penn, and M. R. Johnson, Proc. Natl. Acad. Sci. USA, 1981, 78, 2021. CrossRef
11. J. E. Hong, W. S. Shin, W. B. Jang, and D. Y. Oh, J. Org. Chem., 1996, 61 , 2199. CrossRef