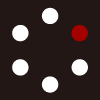
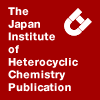
HETEROCYCLES
An International Journal for Reviews and Communications in Heterocyclic ChemistryWeb Edition ISSN: 1881-0942
Published online by The Japan Institute of Heterocyclic Chemistry
e-Journal
Full Text HTML
Received, 29th June, 2010, Accepted, 6th August, 2010, Published online, 10th August, 2010.
DOI: 10.3987/COM-10-S(E)81
■ New Oxidation Products from (-)-Epigallocatechin Gallate in Neutral Solution
Takayo Ohyabu, Shoko Taniguchi, Hideyuki Ito, and Tsutomu Hatano*
Graduate School of Medicine, Dentistry and Pharmaceutical Sciences, Okayama University, 1-1-1 Tsushima-naka, Okayama 700-8530, Japan
Abstract
Two new products, EGCG-MOx-D5 (3) and EGCG-MOx-D6 (4), on the oxidation of (-)-epigallocatechin gallate (EGCG) (1) in its aqueous solution were isolated and their structures were elucidated. These structures and those of previously established ones suggested the pathways of the changes in the oxidation. Rapid decrease of 1 accompanied by the formations of the oxidative products suggested the participation of these products in the biological and pharmacological effects reported to be those of 1.INTRODUCTION
(-)-Epigallocatechin gallate (EGCG) (1) is a major tea constituent and has been found to have various pharmacological effects such as inhibitory effects on tumor promotion,1 and also on the antibiotic resistance shown by methicillin-resistant Staphylococcus aureus (MRSA).2 However, this compound is labile in neutral solution. We investigated on its structural changes in solution, and identify the structures of some monomeric [(-)-gallocatechin gallate and products named EGCG-MOx-Ms] and dimeric products [theasinensins and EGCG-MOx-Ds].3-5 The decrease of 1 in solution was suppressed in the presence of a metal-chelating agent, and the dependence of the changes on metal ions was also seen.3 Further investigation on the structural changes of 1 lead to the isolation of two further new compounds, which is described in this paper. Rapid decrease of EGCG (1) accompanied by the formations of the oxidative products suggested the participation of these products in the biological and pharmacological effects reported to be those of EGCG (1), and this means the importance of the structural studies on these products. Actually, the effectiveness of theasinensin A (2), the representative product from 1, on MRSA was indicative for the participation of such products on the reported effect of 1.3
RESULTS AND DISCUSSION
EGCG (1) was kept in an aqueous solution of pH 7.0 at 35 oC, and the products were separated by column chromatography on MCI-gel CHP-20P and then that on YMC-gel ODS AQ120-50S or on Sephadex LH-20, to give 3 and 4 (Figure 1), in addition to those described in previous reports.3-5
Compound 3 was obtained as a light-brown amorphous powder. The high-resolution electrospray-ionization mass spectrometry (HR-ESI-MS) indicated its molecular formula C44H32O23 by the [M+H]+ ion peak. The 1H NMR spectrum (600 MHz, in acetone-d6 containing D2O) showed two sets of m-coupled doublets [δ 5.87, 5.90 (1H each, d, J = 2.5 Hz; H-8 and H-6 of A-ring in 3); δ 5.98, 6.01 (1H each, d, J = 2.5 Hz; H-6 and H-8 of D-ring in 3)] derived from A-ring of 1, two sets of four protons [δ 4.80 (1H, br s), 5.71 (1H, br m), 2.77 (1H, br d, J = 18 Hz), 2.82 (1H, dd, J = 18, 5 Hz) (H-2, H-3, H-4a and H-4b of C-ring in 3); δ 4.99 (1H, br s), 5.46 (1H, br m) , 3.07 (1H, br d, J = 18 Hz), 2.83 (1H, dd, J = 18, 5 Hz) (H-2, H-3, H-4a and H-4b of F-ring in 3)] derived from C-ring of 1, and two 2H singlets [δ 7.03 (galloyl at O-3 in C-ring of 3) and 7.15 (galloyl at O-3 in F-ring of 3)] due to galloyl groups of two molecules of 1. In addition to these signals, 3 showed two 1H singlets at δ 6.82 and δ 7.74. The one at δ 6.82 is attributable to B-ring H-6 in 3 (which is derived from B-ring of 1), while the remaining one appeared in the lower field suggested some structural change of B-ring of the other molecule of 1 (i.e., E-residue in 3). The 1H-1H correlation spectroscopy (COSY) showed the long-range coupling between the signals of δ 4.80 and δ 6.82, substantiating the connectivity of B-C rings and respective assignments of C-ring H-2 and B-ring H-6. However, the signal at δ 7.74 (E-residue H-6) did not show such correlation on this COSY spectrum.
The 13C NMR spectrum showed carbon signals of A-/D-rings, C-/F-rings, B-ring, and two galloyl groups, derived from two molecules of 1 (See Experimental). In addition to these signals, six carbon signals attributed to E-residue were observed at δC 109.5, 122.6, 154.8, 155.0, 163.5, and 164.4. Among them, one at δC 109.5 was attributed to C-6, and this assignment was supported by the corresponding cross peak (with E-residue H-6) in the HSQC spectrum. Two of the remaining five quaternary carbon signals at δ 163.5 and 164.4 were due to carbons of ester carbonyl/carboxyl groups (E-residue C-4 and C-5). The HMBC spectrum of 3 showed correlations of F-ring H-2 with three carbon signals at δC 154.8 (C-1), 122.6 (C-2), 109.5 (C-6) of E-residue, and also those of E-residue H-6 with four carbon signals at δC 75.7 (F-ring C-2), and at δC 154.8 (C-1), 122.6 (C-2), and 164.4 (C-5), indicating the sequence of the E-residue carbons (Figure 2).
The molecular formula indicated by the mass spectrometry required one of the two carboxyl groups in E-residue is esterified with a hydroxyl group. Indeed, B-ring C-3 (δC 143.1) shifted upfield relative to the corresponding carbon of 2 (δC 144.8). Therefore, the structure where the carboxyl group C-4 forms a lactone with the hydroxyl group at B-ring C-3 (Figure 2) was assigned to this compound.
Compound 4 was obtained as a light-brown amorphous powder. The HR-ESI-MS indicated its molecular formula C44H34O23 by the [M + NH4]+ ion peak, corresponding to the dimeric nature among the products from 1. The 1H NMR spectrum showed signals of A-/D-ring protons [δ 5.87 (1H, d, J = 2.5 Hz), 5.94-5.95 (3H in total, br s)], C-/F-ring protons [δ 5.41 (1H, br s, H-2), 5.91 (1H, br, H-3), 2.92 (1H, br d, J = 17.5 Hz, H-4a), 3.36 (1H, dd, J = 17.5, 4 Hz, H-4b) (C-ring); δ 4.26 (1H, br s, H-2), 5.67 (1H, br, H-3), 2.81 (1H, dd, J = 18, 4 Hz, H-4a), 3.05 (1H, br d, J = 18 Hz, H-4b) (F-ring)], and protons of two galloyl groups [δ 7.11 and 7.13 (2H each, s)]. In addition to these signals, the signals of an aromatic methine proton at δ 6.92 (1H, s, B-ring H-6), two methine protons which coupled with each other [δ 4.68 (1H, d, J = 4 Hz, E-residue H-2) and 5.07 (1H, d, J = 4 Hz, E-residue H-3)], and a set of methylene proton signals [δ 3.19 (1H, br d, J = 16 Hz) and 3.5 (overlapped with the water signal) (E-residue H-6a and H-6b)] were also shown. The presence of the latter proton on the methylene carbon was supported by the cross peak between these two protons in the 1H-1H COSY. These signals, attributed to B-ring and E-residue in formula 4, were regarded to be those derived from B-rings of two molecules of 1. The COSY spectrum also showed the presence of the long-range coupling between B-ring H-6 (δ 6.92) and C-ring H-2 (δ 5.41), indicating the connectivity of the B-C rings.
The 13C NMR spectrum showed six carbon signals attributable to part E, in addition to the carbon signals of A-/D-rings, C-/F-rings, B-ring and two galloyl groups (see Experimental). The chemical shifts and the HSQC spectrum indicated that E-residue involves one methylene [δC 37.0 (bearing H-6a and H-6b) (C-6)], two methine [δC 48.5 (bearing H-2 at δ 4.68) (C-2), δC 79.8 (bearing H-3 at δ 5.07) (C-3)], one a quaternary bearing an oxygen [δC 90.7 (C-1)], and two of carboxyl/ester carbonyl [δC 171.5 (C-4) and 172.0 (C-5)] carbons. These assignments were substantiated by the following correlations observed by the HMBC measurement where the long-range JCH value was set at 5 Hz (see Figure 3): F-ring H-2 with C-1, C-2, C-6 (E-residue) [and a four-bond correlation with C-3 (E-residue)]; E-residue H-2 with C-1, C-2, C-3 (B-ring), C-1, C-3 (E-residue), and C-2 (F-ring); E-residue H-3 with C-1, C-2, C-4 (E-residue), and C-2 (B-ring); E-residue H-6 with C-2 (F-ring), C-1, C-2, and C-5 (E-residue) [and a four-bond correlation with C-3 (E-residue)].
The molecular formula shown by the HR-ESI-MS was the same as that of EGCG-MOx-D1 (5)4 (Figure 4), and the 13C NMR spectral data was also closely similar to those for 5.4 Considering this, the similarity in the pattern of the short-wavelength region in the CD spectrum of 4 ([θ]224 –3.4 x 104, [θ]208 +1.9 x 104) to that of 5 ([θ]222 –2.2 x 104, [θ]208 +1.1 x 104)5 suggested that the stereochemical relationship concerning the B/E moieties for 4 is the same as that in the case of 5. While, the coupling constant H-2 – H-3 of the E-residue in 4 (4 Hz) was different from the corresponding one in 5 (6 Hz). The structure isomeric to 5 concerning E-residue C-3 was thus assigned for 4.
These structures and those of previously reported ones indicated structural diversity of the products formed on the oxidation of the pyrogallol ring of 1 and its dimeric products, and suggested the pathway of the changes in the oxidation process.5 The plausible relationship of compounds 3 and 4 with 1 may be shown by Figure 5.
Occurrence of analogous structural changes was also suggested by structures of several hydrolyzable tannins such as chebulagic acid,6 which were considered to be oxidative products of dehydroellagitannins.7 Therefore, the findings on the changes due to oxidation of the pyrogallol moiety of EGCG (1) and its dimeric products may be applicable to the consideration of the oxidation pathway in the plants producing hydrolyzable tannins.
EXPERIMENTAL
Specific rotations were recorded on a JASCO DIP-1000 digital polarimeter. CD spectra were measured on a JASCO J-720W spectrophotometer. ESIMS were performed using Micromass Auto Spec OA-Tof spectrophotometer with positive-ion mode with a solvent of 50% MeOH + 0.1% AcONH4. The 1H and 13C NMR spectra were recorded on a Varian INOVA AS 600 spectrophotometer (600 MHz for 1H, and 150.7 MHz for 13C) at 300 K. Chemical shifts are given in δ (ppm) values relative to that of the solvent signal [acetone-d6 (δH 2.04; δC 29.8)] on the tetramethylsilane scale.
Materials
EGCG (1) used in this study was isolated from Camellia japonica leaves,3 and kept in a sealed tube. The purity which was estimated using HPLC was over 99%.3
Isolation of products 3 and 4 from 1 at pH 7.0 in a phosphate buffer
EGCG (1) (1.0 g) dissolved in 0.1 M H3PO4 – 0.1 M KH2PO4 buffer (pH 7.0) (1.0 L) was kept at 35 oC for 20 h.8 The solution was acidified to pH 4.0 with 1M HCl, and subjected to column chromatography on MCI-gel CHP-20P with increasing concentration of MeOH in water as eluants. The eluate with 20% MeOH (115 mg) was then chromatographed on YMC-gel AQ120-50S with aq. MeOH to give 3 (3.4 mg). The eluate with 30% MeOH (94 mg) from the MCI-gel column was chromatographed on Sephadex LH-20 with increasing concentrations of MeOH in EtOH to give 4 (6.8 mg).
EGCG-MOx-D5 (3). A light-brown amorphous powder, [α]D20 –106.9 (c 0.29, MeOH). ESI-MS m/z: 929 [M + H]+, 951 [M + Na]+, 967 [M + K]+. HRESIMS m/z: 929.1394 [M + H]+ (calcd. for C44H32O23 + H, 929.1413). UV λ max (MeOH) nm (log ε): 275 (4.37). CD (in MeOH): [θ]280 –1.9 x 104, [θ]240 +1.1 x 103, [θ]219 –6.4 x 104). 1H NMR: see text. 13C NMR (acetone-d6 + D2O; 9:1): δ 26.1 (F-ring C-4), 27.2 (C-ring C-4), 68.3 (C-ring C-3), 69.1 (F-ring C-3), 75.7 (F-ring C-2), 75.8 (C-ring C-2), 95.46 (D-ring C-8), 95.53 (A-ring C-8), 96.4 (A-ring C-6), 96.7 (D-ring C-6), 97.9 (D-ring C-4a), 98.6 (A-ring C-4a), 108.3 (B-ring C-6), 109.5 (E-residue C-6), 110.0 (2 x C, C-2 and C-6 of galloyl at C-ring O-3), 110.1 (2 x C, C-2 and C-6 of galloyl at F-ring O-3), 111.8 (B-ring C-2), 120.9 (C-1 of galloyl at F-ring O-3), 121.5 (C-1 of galloyl at C-ring O-3), 122.6 (E-residue C-2), 130.5 (B-ring C-1), 133.4 (B-ring C-4), 138.8 (C-4 of galloyl at C-ring O-3), 138.9 (C-4 of galloyl at F-ring O-3), 143.1 (B-ring C-3), 145.8 (2 x C, C-3 and C-5 of galloyl at C-ring O-3), 145.9 (2 x C, C-3 and C-5 of galloyl at F-ring O-3), 146.4 (B-ring C-5), 154.8 (E-residue C-1), 155.0 (E-residue C-3), 155.8, 157.0, 157.1, 157.26, 157.30, 157.6 (A- and D-rings C-5, C-7, and C-8a), 163.5 (E-residue C-4), 164.4 (E-residue C-5), 166.4 (C-7 of galloyl at C-ring O-3), 167.0 (C-7 of galloyl at F-ring O-3).
EGCG-MOx-D6 (4). A light-brown amorphous powder, [α]D20 –41.0 (c 0.61, MeOH). ESI-MS m/z: 931 [M + H]+, 953 [M + Na]+, 969 [M + K]+. HRESIMS m/z: 948.1842 [M + NH4]+ (calcd. for C44H34O23 + NH4, 948.1835). UV λ max (MeOH) nm (log ε): 277 (4.38); CD (MeOH): [θ]286 –1.0 x 104, [θ]242 +7.8 x 103, [θ]224 –3.4 x 104, [θ]208 +1.9 x 104. 1H NMR: see text. 13C NMR (acetone-d6 + D2O; 9:1): δ 26.4 (F-ring C-4), 26.8 (C-ring C-4), 37.0 (E-residue C-6), 48.6 (E-residue C-2), 66.0 (F-ring C-3), 70.0 (C-ring C-3), 75.1 (C-ring C-2), 78.6 (F-ring C-2), 79.8 (E-residue C-3), 90.7 (E-residue C-1), 95.5 (A-ring C-6), 95.6, 96.4, 97.8 (A-ring C-6; D-ring C-6 and C-8), 98.3 (D-ring C-4a), 99.3 (A-ring C-4a), 109.9 (2 x C, C-2 and C-6 of galloyl at F-ring O-3), 110.0 (2 x C, C-2 and C-6 of galloyl at C-ring O-3), 110.4 (B-ring C-6), 116.7 (B-ring C-2), 120.8 (C-1 of galloyl at F-ring O-3), 121.6 (C-1 of galloyl at C-ring O-3), 128.7 (B-ring C-1), 129.8 (B-ring C-4), 139.0 (C-4 of galloyl at C-ring O-3), 139.3 (C-4 of galloyl at F-ring O-3), 145.9 (2 x C, C-3 and C-5 of galloyl at C-ring O-3), 146.2 (2 x C, C-3 and C-5 of galloyl at F-ring O-3), 147.0 (B-ring C-5), 148.0 (B-ring C-3), 155.8, 157.33, 157.5 (D-ring C-5, C-7, and C-8a), 157.1, 157.30 (2 x C) (A-ring C-5, C-7, and C-8a), 166.4 (C-7 of galloyl at F-ring O-3), 167.2 (C-7 of galloyl at C-ring O-3), 171.5 (E-residue C-4), 172.0 (E-residue C-5).
ACKNOWLEDGMENTS
The NMR instrument used in this study is the property of the SC-NMR laboratory of Okayama University.
References
1. S. Yoshizawa, T. Horiuchi, H. Fujiki, T. Yoshida, T. Okuda, and T. Sugimura, Phytotherapy Res., 1987, 1, 44. CrossRef
2. S. Shiota, M. Shimizu, T. Mizushima, H. Ito, T. Hatano, T. Yoshida, and T. Tsuchiya, Biol. Pharm. Bull., 1999, 22, 1388.
3. T. Hatano, M. Kusuda, M. Hori, S. Shiota, T. Tsuchiya, and T. Yoshida, Planta Med., 2003, 69, 984. CrossRef
4. T. Hatano, M. Hori, M. Kusuda, T. Ohyabu, H. Ito, and T. Yoshida, Heterocycles, 2004, 63, 1547. CrossRef
5. T. Hatano, T. Ohyabu, and T. Yoshida, Heterocycles, 2005, 65, 303. CrossRef
6. T. Yoshida, T. Okuda, T. Koga, and N. Toh, Chem. Pharm. Bull., 1982, 30, 2655.
7. T. Okuda, Phytochemistry, 2005, 66, 2012. CrossRef
8. Although the major products of this reaction with a shorter incubation time were theasinensins A and D as reported previously,3 prolonged treatment gave various products including 3 and 4.