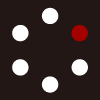
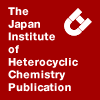
HETEROCYCLES
An International Journal for Reviews and Communications in Heterocyclic ChemistryWeb Edition ISSN: 1881-0942
Published online by The Japan Institute of Heterocyclic Chemistry
e-Journal
Full Text HTML
Received, 24th April, 2010, Accepted, 31st May, 2010, Published online, 2nd June, 2010.
DOI: 10.3987/COM-10-S(E)23
■ Iterative Extension of Thiophene Ring Leading to Head-to-Tail-Type Oligothiophenes via Stepwise CH Arylation and Halogen Exchange Sequence
Shunsuke Tanba, Atsushi Sugie, Naoyuki Masuda, Daiki Monguchi, Nagatoshi Koumura, Kohjiro Hara, and Atsunori Mori*
Department of Chemical Science and Engineering, Faculty of Engineering, Kobe University, Rokkodai-cho, Nada-ku, Kobe 657-8501, Japan
Abstract
Iterative extension of the thiophene ring unit leads to oligothiophenes. The coupling reaction at the CH bond of thiophene with halo-thiophene occurs at the 5-position of 2-bromothiophene with 2-iodothiophene in the presence of a palladium catalyst and AgNO3/KF as an activator to give the corresponding bithiophene, whose carbon-bromine bond remains. Halogen exchange converts the bromine atom to iodide, which also allows further reaction to form thiophene-thiophene bond. Oligothiophenes are obtained by repeating such sequense.INTRODUCTION
As demand increases for oligothiophenes and polythiophenes as advanced electronic and photonic materials, such as organic TFT, liquid crystals, photovoltaic cells, etc.,1,2 preparative methodologies of oligothiophenes have become a significant issue in organic synthesis.3,4 We have recently shown that palladium-catalyzed homocoupling of bromothiophene derivatives, which occurs at the carbon-hydrogen bond of thiophene adjacent to the sulfur atom and the carbon-bromine bond is completely intact, is a highly efficient synthetic pathway for well-defined oligothiophenes and indeed has prepared several oligomers bearing 2-8 thiophene units.5 Although the method is effective for the head-to-head (HH) or tail-to-tail (TT) type oligomers, synthetic design of head-to-tail (HT) oligothiophene that generally shows a higher performance than other regioisomers has been difficult.
Accordingly, transition metal-catalyzed CH functionalization reactions are of great interest in organic synthesis since the reaction shows advantages in atom efficiency compared with related cross coupling with organometallic compounds.6 The reaction of heteroaromatic compounds is particularly important because of their wide utilities in the synthesis of biologically active molecules and advanced organic materials.7 We have recently shown that the catalytic carbon−carbon bond-forming reaction via CH functionalization has been achieved by the reaction of 2-bromothiophene with aryl halides as shown in Scheme 1, 8,9 in which carbon−bromine bond is also intact during the palladium-catalyzed reaction.
Thereby, a new synthetic strategy for HT oligothiophenes based on the CH coupling, which improves synthetic diversity efficiently, is intriguing.10 In addition, recent progress of the HT-type oligothiophenes as materials for organic dye-sensitized11,12 and thin-film13 photovoltaic batteries as well as organic TFTs1c prompted us to develop a facile synthetic pathway for such materials. We herein describe stepwise synthesis of HT regioregular oligothiophenes via iterative palladium-catalyzed CH arylation and halogen exchange reactions leading to the oligomers bearing 2-4 thiophene units.14
RESULTS AND DISCUSSION
Synthetic strategy of the HT-type oligothiophene based on the CH arylation of a 2-bromothiophene derivative at the CH bond is illustrated in Scheme 2. Since palladium-catalyzed CH arylation of heteroaromatic compounds we have shown selectively takes place with an aryl iodide, cross coupling of thiophene and thiophene forms HT bithiophene 3 bearing C–Br bond when the reaction of bromothiophene 1 and iodothiophene 2 is employed. Accordingly, halogen exchange of 3 into iodide 2' and following coupling with 1 bring about additional extension of a thiophene unit and the iterative reactions would lead to HT oligothiophenes.
We first studied the halogen exchange reaction of 2-bromo-3-hexylthiophene 1 leading to 3-hexyl-2-iodothiophene (4). Buchwald has shown that halogen exchange of aryl bromide takes place with sodium iodide in the presence of a catalytic amount of CuI, where sevral diamine ligands are effective to undergo the reaction, to afford the corresponding iodide.15 Thus, we employed such reaction conditions toward the transformation of 1 into 4. When the reaction of 1 was carried out with 2 equiv. of NaI in the presence of a catalytic amount of CuI with N,N'-dimethylethylenediamine under similar conditions shown in the literature, the reaction occurred to afford 4 in 78% yield. The reaction was also performed with several diamine derivatives. The results are summarized in Table 1.
Although the exchange reaction proceeded in moderate to good yields, we found that it is difficult to achieve quantitative conversion of the bromide into the corresponding iodide. Since separation of bromide 1 and iodide 4 by silica gel column chromatography is difficult, the result causes problems to perform further extension of the thiophene ring leading to oligothiophenes. Alternatively, it was found that complete conversion was successful when the transformation reaction was carried out with excess amounts of copper(I) iodide under heterogeneous conditons, which was employed by Yamashita to achieve transformation of an aromatic bromide into iodide.16 After optimization of the conditions as shown in Table 1, almost complete conversion of 1 was achieved with 10 equiv. of CuI and 10 equiv. of LiI in DMSO at 150 ºC to isolate 4 in 97% yield.
Synthesis of HT-type oligothiophene bearing an ester group was carried out with palladium-catalyzed CH arylation and copper-mediated halogen exchange protocols. Scheme 3 shows the synthetic pathway of the head-to-tail oligothiophene bearing four thiophene units and 4-(ethoxycarbonyl)phenyl group at the end group. The reaction of ethyl 4-iodobenzoate 5 with bromothiophene 1 in the presence of PdCl2(PPh3)2 (5 mol%) and AgNO3/KF proceeded smoothly to afford the bromothiophene bearing the aryl group 6 in an excellent yield. The obtained bromothiophene derivative 6 was subjected to the halogen exchange reaction, whose conditions were optimized in the model study, to afford iodothiophene 7 in 91% yield. The CH arylation reaction of 7 with 1 was performed in a similar manner to the reaction of 5 to afford the corresponding HT bithiophene 8. Since CH arylation with aryl iodide bearing an electron-donating substituent has been shown to proceed with inferior yield to that with electron-deficient arenes, thienyl iodide that is recognized as an electron-enriched substrate would result in a lower yield.9b Indeed, the arylation reaction of 7 under similar conditions to that for 5 resulted in a low yield (26%). However, a slight modification of the conditions by a reduced amount of the solvent and repeating addition of AgNO3/KF improved the yield of 8 to 70%. By the iterative halogen-exchange reaction and palladium-catalyzed CH arylation in a similar manner, synthesis of HT-type oligothiophenes up to the tetramer 10 was successfully achieved.
We then examined the synthesis of oligothiophene bearing a carbazole moiety. MK-2 bearing carbazole as a donor and cyanoacrylic acid as an acceptor acts as a sensitizer for dye-sensitized solar cells (DSSCs), that we have previously reported.11a Introduction of the carbazole group was performed by the palladium-catalyzed CH arylation of 2-bromo-3-hexylthiophene 1 with N-ethyl-3-iodocarbazole 11 in the presence of silver(I) nitrate/KF to obtain the corresponding coupling product 12 in 75% yield. Although the halogen exchange reaction with excess amounts of copper salt was employed for the reaction of 12, we only obtained the corresponding iodide 13 in only 21% yield, which would be due to the complexation of the copper reagent to the amino group of carbazole. However, transformation of the bromide into iodide was carried out with bromine–lithium exchange with n-butyllithium and following treatment of iodine at -78 ºC. The reaction proceeded smoothly to afford 13 in 90% yield. The CH coupling reaction of thus obtained 13 with bromothiophene 1 was carried out in the presence of the palladium catalyst and AgNO3/KF to obtain bithiophene 14 (66% yield). Transformation into iodide 15 similarly proceeded (91%). As shown in Scheme 4, further extension of the thiophene unit was similarly successful to afford up to the corresponding trimer 16, which was subjected to protonolysis and CH arylation with thienyl aldehyde 18 to give the tetramer 19. The obtained 19 was identical with the corresponding precursor of the dye molecule MK-2. Transformation of 19 was shown to be performed by Knoevenagel reaction of the formyl group with cyanoacetic acid leading to MK-2 as reported.11b
Effect of the substituent at the 3-position of the thiophene ring plays key role in the design of HT-type oligothiophene to achieve high performance as materials, namely, to improve the planarity between the thiophene rings for the extension of π-conjugation, solubility of oligothiophene in organic solvents by suppressing intermolecular interaction of oligothiophene molecules. It is thereby intriguing that modification of the structure of the substituent of thiophene. We envisaged that introduction of poly-fluorinated alkyl group into the thiophene ring show remarkable characteristics and designed –(CH2)3–(CF2)3–CF3 group, which may improve intermolecular repulsion by the effect of the fluorine atom.17 Nevertheless, electron-negative effect of fluorine would not influence the electronic characteristics to oligothiophene by the introduction of alkyl spacer group, –(CH2)3–, at the thiophene ring. Synthesis of such thiophene derivative 25 was carried out with Wittig reaction of the phosphonium salt 22 with 3-formylthiophene (23) and following catalytic hydrogenation of the carbon–carbon double bond with Pd/C.18 Further transformation reactions of 25 into 26-28 were performed as shown in Scheme 5.
Oligothiophene synthesis with thus obtained 3-substituted thiophene MK-53, MK-54 was carried out as outlined in Schme 6. The CH arylation reaction of bromothiophene derivatives bearing a fluoroalkyl substituent and the following halogen exchange with nBuLi/I2 occured similarly to the case of the hexylthiophene derivatives. The reaction of 32 and 35 with 2-formylthiophene 27 and the following reaction with cyanoacetic acid also proceeded similarly leading to MK-53, MK-54, respectively.
It was also possible to synthesize HT oligothiophene bearing partly fluoroalkylated and partly alkylated moieties as shown in Scheme 7. Synthesis of bithiophenebromide 31 was performed in a similar manner shown in Scheme 6. The obtained bithiophenebromide 31 was subjected to Suzuki-Miyaura coupling19 of a 2-boronic acid ester of 4-hexylthiophene 37 in the presence of a palladium catalyst to afford terthiophene 38, which contains two fluoroalkylated and one alkylated thiophene rings, in 85% yield. Bromination of 38 with NBS and further coupling of the boron reagent 37 afforded the corresponding tetramer composed of two fluoroalkylated and two alkylated thiophenes 40 in a quantitative yield. Introduction of the formyl group with Vilsmeier reaction and the following Knoevenagel reaction with cyanoacetic acid afforded MK-55 in 73% and 69% yields, respectively.11b
Studies on the properties of the obtained oligothiophenes MK-53, MK-54 and MK-55 were carried out. Table 2 summarizes the results. Measurements of UV-vis absorption and photoluminescent spectra indicated that there was little difference of the wavelengths from the structure of the substituent at the 3-position. This is due to the introduction of alkylene spacer connected to the thiophene ring to avoid the influence of the electronegative effect of the perfuluoroalkyl group into oligothiophene. The energy conversion efficiency of oligothiophenes (η) was also preliminary measured. Although the η value of MK-53 and MK-54 was lower than that of MK-2, it was found to slightly improve the value of MK-55 (6.5%). Further investigation of photovoltaic performances of solar cells with MK-53, MK-54, and MK-55 is to be described in due course.
EXPERIMENTAL
General: 1H NMR (400 MHz, 500 MHz) and 13C NMR (100 MHz, 125 MHz) spectra were measured on a Bruker Avance 400 or 500 spectrometer. The chemical shifts were expressed in ppm with CHCl3 (7.26 ppm for 1H) or 13CDCl3 (77.0 ppm for 13C) as internal standards. IR spectra were recorded on PERKIN ELMER FT-IR Spectrometer SPECTRUM 1000, JASCO FT/IR-660 plus Fourier Transform Infrared Spectrometer, or Bruker Alpha with an ATR attachment (Ge). High-resolution mass spectra (EI, FAB, or ESI) were measured by JEOL JMS-700 MStation at the Graduate School of Material Science, Nara Institute of Science and Technology. For thin layer chromatography (TLC) analyses throughout this work, Merck precorted TLC plates (silica gel 60 F254) were used. UV-vis spectra were measured with SHIMADZU UV-3101PC. Photoluminescent spectra were measured with HITACHI F-7000. 1,3-Diiodo-5,5-dimethylhydantoin (DIH) was kindly donated by Nippoh Chemicals Co. Ltd. THF, DMSO, 1,4-dioxane, diethyl ether, acetonitrile, toluene, and DMF (anhydrous grade) were purchased from Wako Pure Chemicals Co. Ltd, or Kanto Chemicals Co. Ltd. and stored under nitrogen atmosphere. Fabrication of solar cells and measurements of the photovoltaic performances were carried out in similar manners to those shown in our previous works.11e
2-Bromo-5-(4-ethoxycarbonylphenyl)-3-hexylthiophene (6): To a 25 mL schlenk tube equipped with a magnetic stirring bar were added PdCl2(PPh3)2 (8.8 mg, 0.01 mmol), 2-bromo-3-hexylthiophene 1 (0.07 mL, 0.3 mmol), ethyl 4-iodobenzoate (5, 69 mg, 0.25 mmol), potassium fluoride (18.2 mg, 0.31 mmol), and anhydrous DMSO (3 mL) under a nitrogen atmosphere. The reaction mixture was stirred at 100 °C for 5 h. Silver nitrate (10.6 mg, 0.31 mmol) was then added in five portions with 1 h interval. After cooling to room temperature, the mixture was passed through a Celite pad, which was washed repeatedly with CHCl3. The filtrate was washed with water twice (50mL x 2) and brine. Then the organic layer was dried over anhydrous sodium sulfate and concentrated under reduced pressure to leave a crude oil, which was purified by chromatography on silica gel using hexane:AcOEt (20:1) as an eluent to afford 85 mg of 15 as a yellow solid (86% yield). 1H NMR (500 MHz, CDCl3) δ 0.90 (3H, t, J = 7.2 Hz), 1.33 (6H, m), 1.40 (3H, t, J = 7.1 Hz), 1.61 (2H, t, J = 7.5 Hz), 2.57 (2H, t, J = 7.5 Hz), 4.38 (2H, q, J = 7.3 Hz), 7.10 (1H, s), 7.55 (2H, d, J = 8.5 Hz), 8.02 (2H, d, J = 8.5 Hz); 13C NMR (125 MHz, CDCl3) δ 14.2, 14.5, 22.7, 29.1, 29.8, 31.8, 61.2, 110.1, 125.1, 125.4, 129.5, 130.4, 138.1, 142.5, 143.7, 166.3; IR (neat) 1108, 1185, 1276, 1442, 1606, 1715, 2856, 2925 cm-1; HRMS (EI+) Calcd for C19H23BrO2S : 394.0602 ; found: m/z 394.0602.
2-Iodo-5-(4-ethoxycarbonylphenyl)-3-hexylthiophene (7): To a 100 mL of schlenk tube equipped with a magnetic stirring bar were added 6 (0.874 g, 2.21 mmol) and 25 mL of anhydrous DMSO under a nitrogen atmosphere. To the solution were added copper(I) iodide (4.2 g, 22.1 mmol) and lithium iodide (2.96 g, 22.1 mmol) and the mixture was stirred at 150 °C for 25 h. After cooling to room temperature, the mixture was poured into water. The mixture was passed through a Celite pad and the cake was washed with CHCl3 repeatedly. The filtrate was washed with aqueous Na2S2O3 and water (100mL x 3). The organic layer was dried over anhydrous sodium sulfate. Removal of the dried solvent left a crude solid, which was purified by chromatography on silica gel using hexane:AcOEt (20:1) as an eluent to afford 0.941 g of 7 as an orange solid (96% yield). 1H NMR (500 MHz, CDCl3) δ 0.90 (3H, t, J = 6.7 Hz), 1.25-1.45 (9H, m), 1.61 (2H, t, J = 7.5 Hz), 2.55 (2H, t, J = 7.6 Hz), 4.38 (2H, q, J = 7.3 Hz), 7.05 (1H, s), 7.56 (2H, d, J = 8.2 Hz), 8.03 (2H, d, J = 8.2 Hz); 13C NMR (125 MHz, CDCl3) δ 14.2, 14.5, 22.7, 29.1, 30.1, 31.8, 32.6, 61.2, 75.5, 125.1, 125.2, 129.6, 130.4, 138.1, 147.6, 148.8, 166.3; IR (neat) 1108, 1185, 1277, 1607, 1711, 2931 cm-1; HRMS (EI+) Calcd for C19H23IO2S : 442.0463; found: m/z 442.0459.
Ethyl 4-(5’-bromo-3,4’-dihexyl-2,2’-bithiophen-5-yl)benzoate (8): The reaction carried out in a similar manner to the synthesis of 6 (70% yield). 1H NMR (500 MHz, CDCl3) δ 0.90 (6H, t, d, J = 6.9, 2.5 Hz), 1.28-1.42 (15H, m), 1.57-1.69 (4H, m), 2.57 (2H, t, J = 7.6 Hz), 2.72 (2H, t, J = 7.9 Hz), 4.39 (2H, q, J = 7.3 Hz), 6.85 (1H, s), 7.23 (1H, s), 7.62 (2H, d, J = 8.2 Hz), 8.03 (2H, d, J = 8.2 Hz); 13C NMR (125 MHz, CDCl3) 14.2, 14.5, 22.8, 29.1, 29.4, 29.6, 29.7, 29.8, 30.7, 31.8, 31.8, 61.1, 109.1, 125.2, 127.0, 127.5, 129.3, 130.4, 131.3, 135.4, 138.2, 140.8, 141.2, 142.7, 166.4; IR (neat) 1281, 1368, 1605, 1706, 2852, 2920, 2951 cm-1; HRMS (EI+) Calcd for C29H37BrO2S2: 560.1418; found: m/z 560.1408.
Ethyl 4-(3,4’,4’’-trihexyl-5’’-iodo[2,2’,5’,2’’]terthiophen-5-yl)benzoate (9): The reaction was carried out by sequential halogen exchange of 7, CH arylation with 1, and further halogen exchange. (overall yield: 58%) 1H NMR (500 MHz, CDCl3) δ 0.87-0.93 (9H, m), 1.24-1.44 (21H, m), 1.57-1.72 (6H, m), 2.55 (2H, t, J = 7.7 Hz), 2.72 (2H, t, J = 7.9 Hz), 2.79 (2H, t, J = 7.9 Hz), 4.39 (2H, q, J = 7.1 Hz), 6.79 (1H, s), 6.98 (1H, s), 7.26 (1H, s), 7.64 (2H, d, J = 8.5 Hz), 8.04 (2H, d, J = 8.5 Hz); 13C NMR (125 MHz, CDCl3) 14.2, 14.4, 22.7, 22.7, 22.7, 29.0, 29.3, 29.3, 29.4, 29.7, 30.0, 30.6, 30.6, 31.7, 31.7, 32.4, 41.1, 61.1, 70.7, 74.2, 125.1, 126.5, 127.6, 128.7, 128.8, 129.1, 130.3, 130.4, 131.7, 134.0, 138.2, 140.3, 140.4, 140.4, 140.9, 147.7, 166.3; IR (neat) 1112, 1268, 1604, 1707, 2855, 2925, 2952; HRMS (EI+) Calcd for C39H51IO2S3: 774.2096; found: m/z 774.2089.
Ethyl 4-(3,4’,4’’,4’’’-tetrahexyl-5’’’-iodo[2,2’,5’,2’’,5’’,2’’’]quaterthiophen-5-yl)benzoate (10): The reaction was carried out by CH arylation of 1 and further halogen exchange.(overall yield: 58%) 1H NMR (500 MHz, CDCl3) δ 0.91-0.98 (12H, m), 1.30-1.50 (27H, m), 1.60-1.77 (8H, m), 2.57 (2H, t, J = 7.6 Hz), 2.74 (2H, t, J = 7.9 Hz), 2.76-2.84 (4H, m), 4.41 (2H, q, J = 7.1 Hz), 6.82 (1H, s), 6.98 (1H, s), 7.02 (1H, s), 7.26 (1H, s), 7.64 (2H, d, J = 8.2 Hz), 8.06 (2H, d, J = 8.2 Hz); 13C NMR (125 MHz, CDCl3) δ14.22, 14.24, 14.5, 22.75, 22.78, 29.1, 29.2, 29.35, 29.40, 29.42, 29.6, 29.7, 29.8, 30.1, 30.5, 30.59, 30.62, 30.65, 30.67, 31.79, 31.82, 32.5, 61.0, 74.1, 108.8, 125.0, 126.4, 126.7, 127.2, 127.6, 128.5, 128.6, 128.9, 129.1, 130.2, 130.28, 130.31, 130.7, 131.9, 133.9, 134.1, 135.5, 135.6, 138.2, 138.3, 140.0, 140.2, 140.25, 140.27, 140.6, 140.8, 142.6, 147.7, 166.3; IR (neat) 1112, 1188, 1267, 1467, 1604, 1717, 2855, 2924 cm-1; HRMS (FAB+) Calcd for C49H65IO2S4: 940.2912; found: m/z 940.2920.
CH functionalization of 2-bromo-3-hexylthiophene (1) with N-ethyl-3-iodo-carbazole (11) leading to 12: To a 25 mL schlenk tube equipped with a magnetic stirring bar were added PdCl2(PPh3)2 (88.8 mg, 0.13 mmol), 2-bromo-3-hexylthiophene 1 (0.65 mL, 3.04 mmol), N-ethyl-3-iodo-carbazole (5) (814 mg, 2.53 mmol), potassium fluoride (368 mg, 6.33 mmol), and anhydrous DMSO (10 mL) under a nitrogen atmosphere. The reaction was stirred at 100 °C for 5 h. Silver nitrate (215 mg, 1.27 mmol) was then added in five portions with an hour interval. After cooling to room temperature, the mixture was passed through a Celite pad, which was washed repeatedly with CHCl3. The filtrate was washed with water twice (50mL x 2) and brine. The organic layer was dried over anhydrous sodium sulfate and concentrated under reduced pressure to leave a crude oil, which was purified by chromatography on silica gel using hexane:AcOEt (50:1) as an eluent to afford 839 mg of 12 as a yellow solid (75% yield).
9-Ethyl-3-(5-iodo-3-n-hexylthiophene2-yl)-9-H-carbazole (13): To a 25 mL schlenk tube equipped with a magnetic stirring bar were added 6 (570 mg, 1.3 mmol) and anhydrous THF (10 mL). Then, a hexane solution of n-BuLi (1.57 M, 1 mL) was added dropwise at -78 ºC under a nitrogen atmosphere. After stirring for 30 min, iodine (660 mg, 2.6 mmol) was added to the resulting solution and stirring was continued for further 30 min at room temperature. The mixture was poured into 5 mL of water and the organic phase was separated. Aqueous was extracted with diethyl ether (30 mL x 2). The combined organic layer was dried over anhydrous sodium sulfate and concentrated to leave a crude oil. Purification by column chromatography on silica gel using hexane:AcOEt (50:1) as an eluent afforded 570 mg of 7 as a colorless solid (90% yield). 1H NMR (500 MHz, CDCl3) δ 0.93 (3H, t, J = 6.8 Hz), 1.30-1.43, (6H, m), .45 (3H, t, J = 7.4 Hz), 1.62-1.67 (2H, m), .59 (2H, br t, J = 7.7 Hz), .37 (2H, q, J = 7.2 Hz), .01 (1H, s), .26 (1H, dd, J = 14.9, 0.8 Hz), .38 (1H, br d, J = 8.2 Hz), .42 (1H, br d, J = 8.2 Hz), .50 (1H, ddd, J = 8.2, 7.1, 0.9 Hz), 7.64 (1H, dd, J = 8.6, 1.7 Hz), 8.13 (1H, br d, J = 8.2 Hz), 8.24 (1H, d, J = 1.7 Hz); 13C NMR (125 MHz, CDCl3) δ 14.0, 14.3, 22.8, 29.1, 30.2, 31.8, 32.7, 37.8, 71.7, 108.8, 108.9, 117.7, 119.3, 120.7, 122.9, 123.0, 123.5, 124.0, 125.4, 126.2, 139.8, 140.6, 148.4, 150.3; IR (neat) 1151, 1236, 1330, 1344, 1444, 1470, 1490, 1598, 2852, 2924, 2949, 3043 cm-1; HRMS (EI+) Calcd for C24H26INS: 487.0831; found: m/z 487.0824.
CH arylation of 1 with 13 leading to 14: The reaction was performed in a similar manner to the synthesis of 5 (66% yield).11b
9-Ethyl-3-(5’-iodo-3’,4-di-n-hexyl[2,2’]bithiophen-5-yl)-9-H-carbazole (15): The reaction was performed in a similar manner to the synthesis of 13 (91% yield). 1H NMR (500 MHz, CDCl3) δ 0.85-0.94 (6H, m), 1.23-1.43 (12H, m), 1.45 (3H, t, J = 7.1 Hz), 1.60-1.74 (4H, m), 2.56 (2H, br t, J = 7.8 Hz), 2.75 (2H, br t, J = 7.9 Hz), 4.38 (2H, q, J = 7.2 Hz), 6.82 (1H, s), 7.17 (1H, s), 7.25-7.27 (1H, m), 7.40 (1H, br d, J = 8.6 Hz), 7.42 (1H, br d, J = 8.4 Hz), 7.49 (1H, ddd, J = 8.4, 7.0, 0.9 Hz), 7.70 (1H, dd, J = 8.6, 1.5 Hz), 8.14 (1H, br d, J = 7.6 Hz), 8.29 (1H, d, J = 1.5 Hz); 13C NMR (125 MHz, CDCl3) δ 13.9, 14.3, 22.7, 22.8, 29.1, 29.4, 29.7, 30.1, 30.8, 31.8, 31.9, 32.5, 37.7, 73.5, 108.7, 108.8, 117.6, 119.2, 120.7, 123.0, 123.5, 124.0, 124.9, 125.3, 126.0, 126.1, 128.5, 139.7, 140.5, 141.0, 141.4, 143.8, 147.6; IR (neat) 1152, 1235, 1344, 1454, 1491, 1597, 2852, 2923, 2950, 3044 cm-1; HRMS (EI+) Calcd for C34H40INS2: 653.1647; found: m/z 653.1649.
Debromination of 16 leading to 17: To a 25 mL schlenk tube equipped with a magnetic stirring bar were added 16 (67.7 mg, 0.088 mmol) and anhydrous Et2O (2 mL). Then, a hexane solution of nBuLi (1.57 M, 1 mL) was added dropwise at -78 ºC under a nitrogen atmosphere. After stirring for 30 min, MeOH (1 mL) was added to the resulting solution and stirring was continued for further 30 min. The mixture was poured into 5 mL of water and the organic phase was separated. Aqueous was extracted with diethyl ether (30 mL x 2). The combined organic layer was dried over anhydrous sodium sulfate and concentrated to leave a crude oil. Purification by column chromatography on silica gel using hexane:AcOEt (50:1) as an eluent afforded 41.1 mg of 17 as a colorless solid (68% yield).
3-Hexyl-5-iodo-2-thiophenecarbaldehyde (18): To a 25 mL of schlenk tube were added 3-hexyl-2-thiophenecarboaldehyde20 (98.2 mg, 0.5 mmol), acetic acid (1.5 mL), and CHCl3 (5 mL). To the solution was added NIS (135 mg, 0.6 mmol) and stirring was continued at 0 ºC for 18 h. The mixture was poured into 10 mL of water and the organic phase was separated. Aqueous was extracted with hexane (20 mL x 2). The combined organic layer was dried over anhydrous sodium sulfate and concentrated to leave a crude oil. Purification by column chromatography on silica gel using hexane:AcOEt (5:1) as an eluent afforded 147 mg of 11 as a pale yellow solid (65% yield). 1H NMR (500 MHz, CDCl3) δ 0.88 (3H, t, J = 6.8 Hz), 1.27-1.37 (6H, m), 1.64 (2H, t, J = 7.7 Hz), 2.90 (2H, t, J = 7.7 Hz), 7.18 (1H, s), 9.87 (1H, s); 13C NMR (125 MHz, CDCl3) δ 14.2, 22.6, 28.2, 29.0, 31.4, 31.6, 87.3, 140.7, 143.8, 153.9, 180.6; IR (ATR) 838, 990, 1217, 1250, 1369, 1391, 1417, 1463, 1528, 1656, 2856, 2926, 2953 cm-1; Calcd for C11H15IOS: 321.9888; found: m/z 321.9890.
3-(4,4,5,5,6,6,7,7,7-Nonafluoroheptyl)thiophene (25): Ph3P 8.73 g, 33 mmol, ICH2CH2C4F9 (11.19 g, 30 mmol) was dissolved in 16 mL of DMF and the mixture was stirred vigorously for 24 h at 105 °C. DMF was removed under reduced pressure. The waxy solid was triturated with diethyl ether, collected by filtration, washed with diethyl ether, and dried under reduced pressure to give 17.9 g of 21 as a white solid, which was used for the following reaction without further purification. Crude 21, 3-formylthiophene (22: 2.47 mL, 28.14 mmol), and K2CO3 (5.06 g, 36.58 mmol), was dissolved in 1,4-dioxane (150 mL) and H2O (6 mL). The resulting mixture was stirred at 95 °C for 20 h. The solvents were removed under reduced pressure, and CH2Cl2 (50 mL) and H2O (50 mL) were added to the orange residue. The aqueous layer was extracted with CH2Cl2 (50 mL) and the organic layers were combined and dried over anhydrous sodium sulfate. Removal of the solvent by rotary evaporator under reduced pressure left an oily solid, which was purified by short-path silica gel chromatography with hexanes to give 7.45 g 23 as a yellow oil. To a Schlenk flask with a magnetic stirring bar were added with 23, 10% Pd/C (0.676 g, 0.63 mmol), and MeOH (80 mL). The resulting mixture was stirred under H2 atmosphere for 24 h at room temperature. After the reaction was complete, the mixture was filtered through Celite pad. The filtrate was concentrated under reduced pressure to leave a crude oil, which was purified by chromatography on silica gel using hexane as an eluent to give 6.63 g of 24 as a colorless oil (overall yield: 65%). 1H NMR (500 MHz, CDCl3) δ 1.89-2.00 (2H, m), 2.02-2.15 (2H, m), 2.74 (2H, t, J = 8.3 Hz), 6.94 (1H, dd, J = 5.0, 1.2 Hz), 6.96-6.99 (1H, m), 7.29 (1H, dd, J = 5.0, 3.0 Hz); 13C NMR (125 MHz, CDCl3) (partial) δ 21.3, 29.6, 30.2, 30.4, 30.6, 120.8, 126.0, 127.9, 141.1; IR (ATR) 718, 768, 880, 1010, 1132, 1220 cm-1; HRMS (EI+) Calcd for C11H9F9S: 344.0281; found: m/z 344.0277.
2-Bromo-3-(4,4,5,5,6,6,7,7,7-nonafluoroheptyl)thiophene (26): To a 500 mL of Schlenk tube equipped with a magnetic stirring bar were added 3c (6.63 g, 19.3 mmol) and 100 mL of THF. The resulting colorless solution was cooled in an ice bath and NBS (3.56 g, 20 mmol) was added in five portions with 1 h interval. Stirring was continued at 0 °C for 5 h and the mixture was poured into water. Aqueous layer was extracted with hexane, the combined organic layer was dried over anhydrous sodium sulfate, and then concentrated under vacuum. An oily residue was purified by chromatography on silica gel using hexanes and following distillation under reduced pressure to afford 7.71g of 26 as a colorless oil (95% yield). 1H NMR (500 MHz, CDCl3) δ 0.87-2.00 (2H, m), 2.02-2.16 (2H, m), 2.69 (2H, t, J = 7.7 Hz), 6.81 (1H, d, J = 5.5 Hz), 7.24 (1H, d, J = 5.5 Hz); 13C NMR (125 MHz, CDCl3) (partial) δ 20.6, 28.7, 29.6, 30.2, 30.3, 30.5, 110.0, 126.1, 127.9, 140.0, 142.2, 145.5; IR (ATR) 720, 878, 1008, 1132, 1218 cm-1; HRMS (EI+) Calcd for C11H8BrF9S: 421.9386; found: m/z 421.9388.
3-(4,4,5,5,6,6,7,7,7-Nonafluoroheptyl)-2-thiophenecarbaldehyde (27): To a 50 mL schlenk tube equipped with a magnetic stirring bar were added 26 (2.12 g, 5.0 mmol) and anhydrous THF (15 mL). A THF solution of iPrMgBr (0.78 M, 8.3 mL) was then added dropwise at -40 ºC under a nitrogen atmosphere. After stirring for 30 min, DMF (5 mL) was added to the resulting solution and stirring was continued for further 30 min. The mixture was poured into 5 mL of water and the organic phase was separated. Aqueous was extracted with diethyl ether (50 mL x 2). The combined organic layer was dried over anhydrous sodium sulfate and concentrated to leave a crude oil. Purification by column chromatography on silica gel using hexane:AcOEt (5:1) as an eluent afforded 1.13 g of 27 as a colorless oil (61% yield). 1H NMR (500 MHz, CDCl3) δ 1.96-2.06 (2H, m), 2.07-2.21 (2H, m), 3.10 (2H, t, J = 7.8 Hz), 7.05 (1H, d, J = 5.0 Hz), 7.71 (1H, d, J = 5.0 Hz), 10.02 (1H, s); 13C NMR (125 MHz, CDCl3) (partial) δ 14.1, 14.2, 21.0, 21.8, 22.7, 27.8, 30.1, 30.3, 30.5, 31.7, 60.5, 130.6, 135.0, 138.2, 149.8, 182.0; IR (ATR) 880, 1008, 1132, 1221, 1424, 1663 cm-1; HRMS (EI+) Calcd for C12H9F9OS: 372.0230; found: m/z 372.0230.
5-Iodo-3-(4,4,5,5,6,6,7,7,7-nonafluoroheptyl)-2-thiophenecarbaldehyde (28): To a 25 mL of schlenk tube were added 27 (395 mg, 1.1 mmol), 0.5 mL of acetic acid, and 0.5 mL of CHCl3. To the solution was added DIH (222 mg, 0.58 mmol) and stirring was continued at room temperature for 24 h. The mixture was poured into 5 mL of water and the organic phase was separated. Aqueous was extracted with diethyl ether (30 mL x 2). The combined organic layer was dried over anhydrous sodium sulfate and concentrated to leave a crude oil. Purification by column chromatography on silica gel using hexane:AcOEt (5:1) as an eluent afforded 443 mg of 28 as a pale yellow solid (84% yield). 1H NMR (500 MHz, CDCl3) δ 1.94-2.03 (2H, m), 2.07-2.22 (2H, m), 3.04 (2H, t, J = 7.9 Hz), 7.21 (1H, s), 9.86 (1H, s); 13C NMR (125 MHz, CDCl3) (partial) δ 21.9, 27.4, 30.1, 30.3, 30.5, 87.6, 140.3, 144.1, 150.9, 180.1; IR (ATR) 880, 1132, 1220, 1419, 1658 cm-1; HRMS (EI+) Calcd for C12H8F9IOS: 497.9197; found: m/z 497.9197.
9-Ethyl-3-(5-bromo-4-(4,4,5,5,6,6,7,7,7-nonafluoroheptyl)thiophene-2-yl)-9-H-carbazole (29): The reaction was performed in a similar manner to the synthesis of 12 (75% yield). 1H NMR (500 MHz, CDCl3) δ 1.45 (3H, t, J = 7.6 Hz), 1.95-2.05 (2H, m), 2.11-2.23 (2H, m), 2.72 (2H, t, J = 7.6 Hz), 4.38 (2H, q, J = 7.3 Hz), 7.03 (1H, s), 7.24-7.28 (1H, m), 7.40 (1H, br d, J = 8.5 Hz), 7.43 (1H, br d, J = 8.1 Hz), 7.50 (1H, ddd, J = 8.1, 7.3, 0.7 Hz), 7.62 (1H, dd, J = 8.5, 1.7 Hz), 8.13 (1H, br d, J = 7.8 Hz), 8.22 (1H, d, J = 1.7 Hz); 13C NMR (125 MHz, CDCl3) (partial) δ 13.9, 20.6, 29.1, 30.3, 30.5, 30.6, 37.8, 107.5, 108.9, 109.0, 117.7, 119.4, 120.7, 122.4, 122.9, 123.6, 123.9, 124.9, 126.3, 139.8, 140.6, 140.9, 146.1; IR (ATR) 880, 1007, 1132, 1231 cm-1; HRMS (EI+) Calcd for C25H19BrF9NS: 615.0278; found: m/z 615.0278.
9-Ethyl-3-(5-iode-4-(4,4,5,5,6,6,7,7,7-nonafluoroheptyl)thiophene2-yl)-9-H-carbazole (30): The reaction was performed in a similar manner to the synthesis of 13 (91% yield). 1H NMR (500 MHz, CDCl3) δ 1.45 (3H, t, J = 7.6 Hz), 1.95-2.05 (2H, m), 2.11-2.23 (2H, m), 2.72 (2H, t, J = 7.6 Hz), 4.38 (2H, q, J = 7.3 Hz), 7.03 (1H, s), 7.24-7.28 (1H, m), 7.40 (1H, br d, J = 8.5 Hz), 7.43 (1H, br d, J = 8.1 Hz), 7.50 (1H, ddd, J = 8.1, 7.3, 0.7 Hz), 7.62 (1H, dd, J = 8.5, 1.7 Hz), 8.13 (1H, br d, J = 7.8 Hz), 8.22 (1H, d, J = 1.7 Hz); 13C NMR (125 MHz, CDCl3) (partial) δ 13.9, 20.9, 30.3, 30.5, 30.6, 31.9, 37.8, 72.4, 108.9, 108.9, 117.8, 119.4, 120.7, 122.4, 122.9, 123.5, 124.0, 125.1, 126.3, 139.9, 140.6, 146.1, 151.2; IR (ATR) 802, 847, 884, 1009, 1088, 1128, 1205, 1230, 1273, 1340, 1436, 1470, 1490 cm-1; HRMS (FAB+) Calcd for C25H19F9INS: 663.0139; found: m/z 663.0137.
9-Ethyl-3-(5’-bromo-3,4’-di-(4,4,5,5,6,6,7,7,7-nonafluoroheptyl)-[2,2’]bithiophen-5-yl)-9-H-carbazole (31): The reaction was performed in a similar manner to the synthesis of 12 (74% NMR (500 MHz, CDCl3) δ 1.46 (3H, t, J = 7.0 Hz), 1.93-2.07 (4H, m), 2.09-2.22 (4H, m), 2.71 (2H, t, J = 7.5 Hz), 2.86 (2H, t, J = 7.9 Hz), 4.39 (2H, q, J = 7.3 Hz), 6.85 (1H, s), 7.18 (1H, s),7.24-7.28 (1H, m), 7.42 (1H, br d, J = 8.3 Hz), 7.43 (1H, br d, J = 8.3 Hz), 7.50 (1H, ddd, J = 8.3, 7.1, 1.2 Hz), 7.70 (1H, dd, J = 8.3, 1.8 Hz), 8.14 (1H, br d, J = 7.8 Hz), 8.29 (1H, d, J = 1.8 Hz); 13C NMR (125 MHz, CDCl3) (partial) δ 13.9, 20.5 , 21.4, 28.8, 28.9, 30.2, 30.4, 30.6, 37.8, 108.9, 109.0, 109.5, 117.8, 119.4, 120.7, 123.0, 123.6, 124.0, 124.2, 124.9, 126.3, 126.3, 128.7, 136.4, 139.0, 139.9, 140.6, 140.6, 145.1; IR (ATR) 847, 875, 1009, 1132, 1215, 1271, 1348, 1454 cm-1; HRMS (FAB+) Calcd for C36H26BrF18NS2: 957.0403; found: m/z 957.0403.
9-Ethyl-3-(5’-iode-3,4’-di-(4,4,5,5,6,6,7,7,7-nonafluoroheptyl)-[2,2’]bithiophen-5-yl)-9-H-carbazole (32): The reaction was performed in a similar manner to the synthesis of 13 (91% yield). 1H NMR (500 MHz, CDCl3) δ 1.46 (3H, t, J = 7.3 Hz), 1.92-2.07 (4H, m), 2.10-2.24 (4H, m), 2.69 (2H, t, J = 7.9 Hz), 2.87 (2H, t, J = 7.9 Hz), 4.39 (2H, q, J = 7.2 Hz), 6.81 (1H, s), 7.18 (1H, s), 7.24-7.29 (1H, m), 7.42 (1H, br d, J = 8.1 Hz), 7.43 (1H, br d, J = 8.3 Hz), 7.50 (1H, ddd, J = 8.3, 7.1, 1.2 Hz), 7.70 (1H, dd, J = 8.1, 1.3 Hz), 8.14 (1H, br d, J = 7.8 Hz), 8.29 (1H, br s); 13C NMR (125 MHz, CDCl3) (partial) δ 14.0, 20.8, 21.3, 28.8, 30.4, 30.5, 30.6, 30.6, 30.8, 31.7, 31.7, 37.8, 74.7, 108.9, 109.0, 117.8, 119.4, 120.7, 123.0, 123.6, 124.0, 124.2, 124.9, 126.1, 126.3, 128.8, 139.0, 139.9, 140.6, 141.5, 145.1, 145.7; IR (ATR) 877, 1006, 1130, 1218, 1341, 1453 cm-1; HRMS (FAB+) Calcd for C36H26F18INS2: 1005.0264; found: m/z 1005.0258.
5’’-(9-Ethyl-9H-carbazol-3-yl)-3’,3’’,4-tri-(4,4,5,5,6,6,7,7,7-nonafluoroheptyl)-[2,2’,5’,2’’]terthiophene-5-carbaldehyde (33): To a 25 mL of Schlenk tube equipped with a magnetic stirring bar were added PdCl2(PPh3)2 (5.3 mg, 0.0075 mmol), 27 (112 mg, 0.30 mmol), 32 (152 mg, 0.15 mmol), potassium fluoride (22 mg, 0.375 mmol), and anhydrous DMSO (1 mL) under a nitrogen atmosphere. The reaction mixture was stirred at 100 °C for 5 h. Silver nitrate (64 mg, 0.375 mmol) was then added in five portions with 1 hour interval. After cooling to room temperature, the mixture was passed through a Celite pad and the cake was washed repeatedly with CHCl3. The filtrate was washed with water twice (50mL x 2) and brine. The combined organic layer was dried over anhydrous sodium sulfate and concentrated under reduced pressure to leave a crude oil, which was purified by chromatography on silica gel using hexane:AcOEt (5:1) as an eluent to afford 83 mg of 33 as a orange solid (44% yield). 1H NMR (500 MHz, CDCl3) δ 1.46 (3H, t, J = 7.2 Hz), 2.00-2.29 (12H, m), 2.95 (4H, m), 3.08 (2H, t, J = 7.7 Hz), 4.39 (2H, q, J = 7.2 Hz), 7.03 (1H, s), 7.07 (1H, s), 7.21 (1H, s), 7.24-7.31 (1H, m), 7.39-7.46 (2H, m), 7.51 (1H, ddd, J = 8.2, 7.2, 1.0 Hz), 7.72 (1H, dd, J = 8.5, 1.6 Hz), 8.15 (1H, d, J = 7.8 Hz), 8.31 (1H, d, J = 1.6 Hz) 10.00 (1H, s); 13C NMR (125 MHz, CDCl3) (partial) δ 14.0, 21.0, 21.3, 21.9, 27.9, 29.0, 29.1, 30.3, 30.4, 30.6, 37.9, 108.9, 109.0, 117.9, 119.4, 120.7, 123.0, 123.7, 124.0, 124.6, 124.8, 126.4, 128.3, 128.5, 129.9, 137.0, 137.1, 139.4, 140.0, 140.4, 140.6, 144.9, 145.5, 150.4, 181.3; IR (ATR) 1132, 1221, 1435, 1639 cm-1; HRMS (FAB+) Calcd for C48H34F27NOS3: 1249.1371; found: m/z 1249.1377.
2-Cyano-3-[5’’-(9-Ethyl-9H-carbazol-3-yl)-3’,3’’,4-tri-(4,4,5,5,6,6,7,7,7-nonafluoroheptyl)-[2,2’,5’,2’’]terthiophen-5-yl]acrylic acid (MK-53): A mixture of aldehyde 33 (81 mg, 0.065 mmol) and cyanoacetic acid (28 mg, 0.32 mmol) in MeCN (2 mL), toluene (2 mL), and piperidine (0.5 mL) was refluxed for 24 h. After cooling to room temperature, the mixture was diluted with CH2Cl2, and the organic layer was washed with aqueous HCl (1 M), H2O and brine. The combined organic layer was dried over anhydrous Na2SO4 and evaporated under reduced pressure. The crude product was purified by column chromatography (CHCl3/EtOH, gradient: 100/0 to 10/1) to obtain MK-53 (35 mg, 41%) as dark-red solid. 1H NMR (400 MHz, THF-d8) δ 1.46 (3H, t, J = 7,2 Hz), 1.95-2.13 (6H, m), 2.27-2.46 (6H, m), 2.98-3.08 (6H, m), 4.45 (2H, q, J = 7.2 Hz), 7.20 (1H, m), 7.21 (1H, s), 7.32 (1H, s), 7.41 (1H, s), 7.44 (1H, m), 7.50 (1H, br d, J = 8.2 Hz), 7.54 (1H, br d, J = 8.6 Hz), 7.74 (1H, dd, J = 8.6, 1.7 Hz), 8.14 (1H, d, J = 7.8 Hz), 8.40 (1H, d, J = 1.7 Hz), 8.43 (1H, s).
9-Ethyl-3-(5’’-bromo-3,4’,4’’-tri-(4,4,5,5,6,6,7,7,7-nonafluoroheptyl)-[2,2’,5’,2’’]terthiophen-5-yl)-9-H-carbazole (34): The reaction was performed in a similar manner to the synthesis of 12 (40% yield). 1H NMR (500 MHz, CDCl3) δ 1.46 (3H, t, J = 7.1 Hz), 1.92-2.24 (12H, m), 2.71 (2H, t, J = 7.6 Hz), 2.84 (2H, t, J = 8.0 Hz), 2.93 (2H, t, J = 7.7 Hz), 4.40 (2H, q, J = 7.1 Hz), 6.83 (1H, s), 6.98 (1H, s), 7.19 (1H, s), 7.24-7.29 (1H, m), 7.40-7.45 (2H, m), 7.50 (1H, ddd, J = 8.3, 7.1, 1.0 Hz), 7.71 (1H, dd, J = 8.5, 1.5 Hz), 8.15 (1H, br d, J = 7.8 Hz), 8.31 (1H, d, J = 1.5 Hz); 13C NMR (125 MHz, CDCl3) (partial) δ 13.9, 20.5, 21.3, 28.5, 28.9, 29.0, 30.2, 30.4, 30.5, 30.7, 30.7, 37.8, 108.9, 109.0, 110.2, 117.8, 119.4, 120.7, 123.0, 123.6, 124.0, 124.5, 124.9, 126.3, 126.8, 127.8, 129.0, 130.3, 135.5, 135.6, 138.4, 138.8, 139.9, 140.6, 140.7, 144.9; IR (ATR) 877, 1007, 1132, 1220, 1347, 1453 cm-1; HRMS (FAB+) Calcd for C47H34BrF27NS3 [M+H]+: 1300.0606; found: m/z 1300.0547.
9-Ethyl-3-(3,4’,4’’-tri-(4,4,5,5,6,6,7,7,7-nonafluoroheptyl)-[2,2’,5’,2’’]terthiophen-5-yl)-9-H-carbazole (35): The reaction was performed in a similar manner to the synthesis of 17 (98% yield). 1H NMR (500 MHz, CDCl3) δ 1.46 (3H, t, J = 7.0 Hz), 1.97-2.29 (12H, m), 2.76 (2H, t, J = 7.2 Hz), 2.90 (2H, t, J = 7.8 Hz), 2.95 (2H, t, J = 7.7 Hz), 4.38 (2H, q, J = 7.1 Hz), 6.98-7.06 (3H, m), 7.21 (1H, s), 7.25-7.32 (1H, m), 7.39-7.47 (2H, m), 7.49-7.56 (1H, m), 7.73 (1H, dd, J = 8.4, 1.2 Hz), 8.17 (1H, br d, J = 7.6 Hz), 8.33 (1H, br d, J = 1.2 Hz); 13C NMR (125 MHz, CDCl3) (partial) δ 13.8, 21.1, 21.1, 21.3, 21.3, 21.4, 21.4, 28.6, 29.0, 29.7, 30.4, 30.5, 30.6, 30.6, 30.7, 37.7, 76.9, 77.2, 77.4, 108.9, 109.0, 117.7, 119.4, 120.7, 121.4, 123.0, 123.7, 123.9, 124.4, 125.1, 126.3, 127.2, 127.9, 129.3, 131.5, 134.9, 135.8, 137.9, 138.7, 139.9, 140.7, 141.8, 144.6; IR (ATR) 880, 1010, 1132, 1220 cm-1; HRMS (ESI+) Calcd for C47H34F27NS3: 1221.1422; found: m/z 1221.1422.
5’’’-(9-Ethyl-9H-carbazol-3-yl)-3’,3’’,3’’’,4-tetra-(4,4,5,5,6,6,7,7,7-nonafluoroheptyl)-[2,2’,5’,2’’,5’’,2’’’]quaterthiophene-5-carbaldehyde (36): To a 25 mL schlenk tube equipped with a magnetic stirring bar were added PdCl2(PPh3)2 (4.4 mg, 0.0062 mmol), 28 (174 mg, 0.14 mmol), 35 (151 mg, 0.12 mmol), potassium fluoride (18 mg, 0.31 mmol), and anhydrous DMSO (2 mL) under a nitrogen atmosphere. The mixture was stirred at 100 °C for 5 h. Silver nitrate (53 mg, 0.31 mmol) was then added in five portions with 1 h interval. After cooling to room temperature, the mixture was passed through a Celite pad, which was washed repeatedly with CHCl3. The filtrate was washed with water twice (50mL x 2) and brine. The organic layer was dried over anhydrous sodium sulfate and concentrated under reduced pressure to leave a crude oil, which was purified by chromatography on silica gel using hexane:AcOEt (5:1) as an eluent to afford 86 mg of 36a as a reddish solid (44% yield). 1H NMR (500 MHz, CDCl3) δ 1.46 (3H, t, J = 7.3 Hz), 1.99-2.28 (16H, m), 2.89-2.99 (6H, m), 3.10 (2H, t, J = 7.4 Hz), 4.40 (2H, q, J = 7.2 Hz), 7.02 (1H, s), 7.02 (1H, s), 7.08 (1H, s), 7.21 (1H, s), 7.24-7.30 (1H, m), 7.43 (1H, br d, J = 8.5 Hz), 7.44 (1H, br d, J = 8.2 Hz), 7.50 (1H, ddd, J = 8.2, 7.1, 1.1 Hz), 7.72 (1H, dd, J = 8.5, 1.8 Hz), 8.15 (1H, br d, J = 7.7 Hz), 8.31 (1H, br d, J = 1.8 Hz), 10.01 (1H, s); 13C NMR (125 MHz, CDCl3) (partial) δ 14.0, 21.1, 21.3, 21.4, 21.9, 27.9, 28.8, 28.9, 29.0, 30.4, 30.6, 30.7, 37.9, 108.9, 109.0, 117.8, 119.4, 120.7, 123.0, 123.6, 124.0, 124.6, 124.9, 126.3, 128.1, 128.5, 128.7, 128.9, 130.1, 130.5, 135.8, 136.2, 137.2, 138.8, 139.0, 139.9, 140.4, 140.7, 144.6, 145.0, 150.4, 181.3; IR (ATR) 876, 1007, 1132, 1220, 1657 cm-1; HRMS (FAB+) Calcd for C59H41F36NOS4: 1591.1496; found: m/z 1591.1510.
2-Cyano-3-[5’’’-(9-Ethyl-9H-carbazol-3-yl)-3’,3’’,3’’’,4-tetra-(4,4,5,5,6,6,7,7,7-nonafluoroheptyl)-[2,2’,5’,2’’,5’’,2’’’]quaterthiophenyl-5-yl]acrylic acid (MK-54): The reaction was performed in a similar manner to the synthesis of MK-53 (41% yield). 1H NMR spectrum was measured to be very broad due to the heavy dye-aggregation, therefore we could not analyze the coupling constant of each chemical shift. 1H NMR (400 MHz, THF-d8) δ 1.41 (3H), 1.96-2.12 (8H, m), 2.30-2.45 (8H, m), 2.96-3.08 (8H, m), 4.43 (2H), 7.16-7.21 (3H, m), 7.30 (1H), 7.39-7.45 (2H, m), 7.46-7.56 (2H, m), 7.73 (1H), 8.13 (1H), 8.40 (1H), 8.43 (1H).
9-Ethyl-3-(4”-n-hexyl-3,4’-di-(4,4,5,5,6,6,7,7,7-nonafluoroheptyl)-[2,2’,5’,2’’]terthiophen-5-yl)-9-H-carbazole (38): The mixture of 31 (236 mg, 0.25 mmol), 4-hexylthiophene-2-boronic acid ester 37 (103 mg, 0.37 mmol), tetrakis(triphenylphosphine)palladium (14 mg, 0.012 mmol) and 1.5 mL of 2 M aqueous solution of Na2CO3 in dimethoxyethane (5 mL) was refluxed for 24 h. After cooling to room temperature, H2O was added and the reaction mixture was extracted with AcOEt three times. The combined organic layer was washed with H2O and brine and dried over anhydrous MgSO4. The dried solvent was concentrated under reduced pressure to leave a crude product, which was purified by column chromatography on silica gel (hexane/EtOAc = 50/1) and successive HPLC on silica gel (hexane/EtOAc = 50/1) to obtain bithiophene 2 (220 mg, 85%) as a slightly yellow oil. The product was employed for the following reaction without further purification. 1H NMR (400 MHz, CDCl3) δ 0.90 (3H, t, J = 7.0 Hz), 1.30-1.40 (6H, m), 1.46 (3H, t, J = 7.2 Hz), 1.61-1.70 (2H, m), 1.98-2.09 (4H, m), 2.10-2.23 (4H, m), 2.63 (2H, t, J = 7.7 Hz), 2.89 (2H, t, J = 7.7 Hz), 2.94 (2H, t, J = 7.7 Hz), 4.39 (2H, q, J = 7.2 Hz), 6.94-6.95 (1H, m), 6.97-6.98 (2H, m), 7.19 (1H, s), 7.27 (1H, ddd, J = 7.8, 7.0, 1.0 Hz), 7.42 (1H, br d, J = 8.5 Hz), 7.43 (1H, br d, J = 8.2 Hz), 7.50 (1H, ddd, J = 8.2, 7.0, 1.2 Hz), 7.72 (1H, dd, J = 8.5, 1.8 Hz), 8.14 (1H, br d, J = 7.8 Hz), 8.29 (1H, d, J = 1.8 Hz); 13C NMR (100 MHz, CDCl3) (partial) δ 13.7, 14.0, 21.1, 21.2, 22.6, 28.4, 28.8, 29.0, 30.37, 30.46, 30.51, 31.7, 37.6, 108.7, 108.8, 117.5, 119.2, 120.4, 120.6, 122.8, 123.4, 123.8, 124.2, 124.9, 126.1, 127.65, 127.70, 129.2, 131.8, 134.4, 134.7, 137.4, 138.3, 139.7, 140.4, 143.9, 144.3.
9-Ethyl-3-(5’’-bromo-4”-n-hexyl-3,4’-di-(4,4,5,5,6,6,7,7,7-nonafluoroheptyl)-[2,2’,5’,2’’]terthiophen-5-yl)-9-H-carbazole (39): To a solution of 38 (210 mg, 0.20 mmol) in THF (5 mL) was added N-bromosuccinimide (36 mg, 0.20 mmol). The reaction mixture was stirred at room temperature for 30 min and quenched with 10% aqueous solution of Na2CO3 and extracted with EtOAc three times. The combined organic layer was washed with H2O and brine, dried over MgSO4, and evaporated under reduced pressure. The crude product was purified by column chromatography (hexane/EtOAc = 50/1) and successive HPLC on silica gel (hexane/EtOAc = 50/1) to obtain bromide 39 (200 mg, 88%) as a slightly yellow oil. The product was employed for the following reaction. 1H NMR (400 MHz, CDCl3) δ 0.90 (3H, t, J = 7.0 Hz), 1.30-1.40 (6H, m), 1.46 (3H, t, J = 7.2 Hz), 1.58-1.66 (2H, m), 1.96-2.10 (4H, m), 2.14-2.27 (4H, m), 2.58 (2H, t, J = 7.7 Hz), 2.85 (2H, t, J = 7.7 Hz), 2.93 (2H, t, J = 7.7 Hz), 4.39 (2H, q, J = 7.2 Hz), 6.83 (1H, s), 6.97 (1H, s), 7.19 (1H, s), 7.27 (1H, ddd, J = 7.8, 7.0, 1.0 Hz), 7.42 (1H, br d, J = 8.5 Hz), 7.43 (1H, br d, J = 8.2 Hz), 7.50 (1H, ddd, J = 8.2, 7.0, 1.2 Hz), 7.72 (1H, dd, J = 8.5, 1.7 Hz), 8.14 (1H, br d, J = 7.8 Hz), 8.30 (1H, d, J = 1.7 Hz); 13C NMR (100 MHz, CDCl3) (partial) δ 13.8, 14.0, 21.1, 21.2, 22.6, 28.4, 28.8, 28.9, 29.56, 29.63, 30.2, 30.3, 30.4, 30.5, 30.6, 30.7, 31.6, 37.6, 108.7, 108.8, 109.1, 117.5, 119.2, 120.6, 122.8, 123.4, 123.8, 124.2, 124.8, 126.1, 127.2, 127.6, 129.0, 130.6, 134.6, 135.0, 138.0, 138.5, 139.7, 140.5, 142.8, 144.6.
5’’’-(9-Ethyl-9H-carbazol-3-yl)-3’,4-di-n-hexyl-3”,3’’’-di-(4,4,5,5,6,6,7,7,7-nonafluoroheptyl)-[2,2’,5’,2’’,5’’,2’’’]quaterthiophene (40): The reaction was performed in a similar manner to the synthesis of 38 (98% yield) and employed for the following reaction without further purification. 1H NMR (400 MHz, CDCl3) δ 0.925 (3H, t, J = 7.0 Hz), 0.931 (3H, t, J = 7.0 Hz), 1.31-1.44 (12H, m), 1.47 (3H, t, J = 7.2 Hz), 1.64-1.74 (4H, m), 2.01-2.13 (4H, m), 2.14-2.30 (4H, m), 2.65 (2H, t, J = 7.7 Hz), 2.79 (2H, t, J = 7.8 Hz), 2.94 (2H, t, J = 7.9 Hz), 2.96 (2H, t, J = 7.9 Hz), 4.39 (2H, q, J = 7.2 Hz), 6.94-6.95 (1H, m), 7.00 (1H, m) 7.01 (1H, s), 7.02 (1H, d, J = 1.4 Hz), 7.21 (1H, s), 7.29 (1H, ddd, J = 7.8, 7.1, 1.0 Hz), 7.42 (1H, br d, J = 8.5 Hz), 7.43 (1H, br d, J = 8.2 Hz), 7.52 (1H, ddd, J = 8.2, 7.1, 1.2 Hz), 7.73 (1H, dd, J = 8.5, 1.8 Hz), 8.17 (1H, br d, J = 7.8 Hz), 8.33 (1H, d, J = 1.8 Hz); 13C NMR (100 MHz, CDCl3) (partial) δ 13.7, 13.98, 14.02, 21.09, 21.12, 21.2, 22.6, 28.5, 28.8, 29.0, 29.27, 29.31, 30.26, 30.30, 30.40, 30.49, 30.52, 30.6, 30.70, 30.74, 31.6, 31.7, 37.6, 108.7, 108.8, 115.7, 116.0, 116.4, 117.5, 118.0, 118.27, 118.35, 118.6, 118.9, 119.2, 120.2, 120.6, 122.8, 123.4, 123.8, 124.2, 124.9, 126.1, 127.3, 127.7, 128.9, 129.2, 131.3, 131.6, 132.7, 134.4, 135.3, 137.5, 138.4, 139.7, 139.8, 140.4, 143.8, 144.4.
5’’’-(9-Ethyl-9H-carbazol-3-yl)-3’,4-di-n-hexyl-3”,3’’’-di-(4,4,5,5,6,6,7,7,7-nonafluoroheptyl)-[2,2’,5’,2’’,5’’,2’’’]quaterthiophene-5-carbaldehyde (41): To a solution of quoterthiophene 40 (190 mg, 0.16 mmol) in dry DMF (2 mL) at 0 °C was added 0.17 mL of a 2.68 M solution in DMF of Vilsmeier reagent. The mixture was stirred at 70 °C for 7 h and cooled to room temperature. The mixture was quenched with 10% aqueous solution of NaOAc (30 mL). Two layers were separated and the aqueous layer was extracted with EtOAc three times. The combined organic layer was washed with H2O and brine, dried over anhydrous MgSO4. The solvent was removed under reduced pressure. The crude product was purified by column chromatography (hexane/EtOAc = 20/1) and successive HPLC on silica gel (hexane/EtOAc = 7/1) to obtain aldehyde 41a (142 mg, 73%) as a reddish solid, which was used for further reaction. 1H NMR (400 MHz, CDCl3) δ 0.91 (3+3H, t, J = 7.0 Hz), 1.33-1.46 (12H, m), 1.46 (3H, t, J = 7.2 Hz), 1.66-1.76 (4H, m), 2.01-2.12 (4H, m), 2.15-2.29 (4H, m), 2.84 (2H, t, J = 7.7 Hz), 2.91-2.98 (6H, m), 4.39 (2H, q, J = 7.2 Hz), 7.01 (1+1H, s), 7.06 (1H, s), 7.20 (1H, s), 7.27 (1H, ddd, J = 7.8, 7.0, 1.0 Hz), 7.42 (1H, br d, J = 8.5 Hz), 7.43 (1H, br d, J = 8.2 Hz), 7.51 (1H, ddd, J = 8.2, 7.0, 1.2 Hz), 7.71 (1H, dd, J = 8.5, 1.8 Hz), 8.15 (1H, br d, J = 7.8 Hz), 8.31 (1H, d, J = 1.8 Hz), 10.03 (1H, s); 13C NMR (100 MHz, CDCl3) (partial) δ 13.7, 13.9, 21.0, 21.1, 22.5, 22.6, 28.4, 28.8, 29.0, 29.2, 29.8, 30.17, 30.25, 30.4, 30.5, 30.6, 30.7, 31.4, 31.5, 31.6, 37.6, 108.7, 108.8, 116.0, 117.5, 118.8, 119.2, 120.5, 120.6, 122.8, 123.4, 123.7, 124.2, 124.7, 126.1, 127.7, 128.2, 128.9, 129.2, 129.9, 130.5, 134.9, 135.2, 136.4, 138.2, 138.6, 139.7, 140.4, 142.5, 144.6, 144.7, 153.3, 181.5.
2-Cyano-3-[5’’’-(9-Ethyl-9H-carbazol-3-yl)-3’,4-di-n-hexyl-3”,3’’’-di-(4,4,5,5,6,6,7,7,7-nonafluoro-
heptyl)-[2,2’,5’,2’’,5’’,2’’’]quaterthiophenyl-5-yl]acrylic acid (MK-55): The reaction was performed in a similar manner to the synthesis of MK-53 (69% yield). 1H NMR spectrum was measured to be very broad as well as MK-54, therefore we could not analyze the coupling constant of each chemical shift. 1H NMR (400 MHz, THF-d8) δ 0.91 (6H, m), 1.31-1.44 (15H, m), 1.46-1.52 (2H, m), 1.64-1.70 (2H, m), 2.02-2.12 (4H, m), 2.26-2.41 (4H, m), 4.44 (2H), 7.14-7.24 (4H, m), 7.39 (1H), 7.43 (1H), 7.48-7.54 (2H, m), 7.73 (1H), 8.14 (1H), 8.39 (1H), 8.42 (1H).
ACKNOWLEDGEMENTS
This work was partially supported by Grant-in-Aids for Scientific Research (B) and on Priority Areas, "Advanced Molecular Transformation of Carbon Resources" by Ministry of Education, Culture, Sports, Science and Technology (MEXT), Japan and by Research for Promoting Technological Seeds by Japan Science and Technology Agency. The authors thank Kyoto Advanced Nanotechnology Network supported by MEXT for measurement of high-resolution mass spectra, which was performed at Graduate School of Materials Science, Nara Institute of Science and Technology.
References
1. a) C. A. Briehn, M.-S. Schiedel, E. M. Bonsen, W. Schuhmann, and P. Buerle, Angew. Chem. Int. Ed., 2001, 40, 4680; CrossRef b) J. M. Tour, Chem. Rev., 1996, 96, 537; CrossRef c) A. Facchetti, M.-H. Yoon, C. L. Stern, H. E. Katz, and T. J. Marks, Angew. Chem. Int. Ed., 2003, 42, 3900; CrossRef d) R. D. McCullough, Adv. Mater., 1998, 10, 93; CrossRef e) T. Yamamoto, Macromol. Rapid Commun., 2002, 23, 583; CrossRef f) T. Izumi, S. Kobashi, K. Takimiya, Y. Aso, and T. Otsubo, J. Am. Chem. Soc., 2003, 125, 5286; CrossRef g) T. Otsubo, Y. Aso, and K. Takimiya, Bull. Chem. Soc. Jpn., 2001, 74, 1789; CrossRef h) M. Funahashi and J.-I. Hanna, Adv. Mater., 2005, 17, 594; CrossRef i) X. Wu, T.-A. Chen, and R. D. Rieke, Macromolecules, 1996, 29, 7671; CrossRef j) J. Hassan, C. Gozzi, E. Schulz, and M. J. Lemaire, Organomet. Chem., 2003, 687, 280. CrossRef
2. a) T. Takahashi, K. Matsuoka, K. Takimiya, T. Otsubo, and Y. Aso, J. Am. Chem. Soc., 2005, 127, 8928; CrossRef b) A. R. Murphy, J. M. J. Fréchet, P. Chang, J. Lee, and V. Subramanian, J. Am. Chem. Soc., 2004, 126, 1596; CrossRef c) K. Matsumoto, T. Tanaka, S. Kugo, T. Inagaki, Y. Hirao, H. Kurata, T. Kawase and, T. Kubo, Chem. Asian J., 2008, 3, 2024; CrossRef d) R. Takita, C. Song and, T. M. Swager, Org. Lett., 2008, 10, 5003; CrossRef e) J. Lu, F. Liang, N. Drolet, J. Ding, Y. Tao, and R. Movileanu, Chem. Commun., 2008, 5315; CrossRef f) J. Casado, K. Takimiya, T. Otsubo, F. J. Ramrez, J. J. Quirante, R. P. Ortiz, S. R. Gonzaàlez, M. M. Oliva, and J. T. L. Navarrete, J. Am. Chem. Soc., 2008, 130, 14028; CrossRef g) A. Tsuge, T. Hara, T. Moriguchi, and M. Yamaji, Chem. Lett., 2008, 37, 870; CrossRef h) D.-S. Kim and K. H. Ahn, J. Org. Chem., 2008, 73, 6831; CrossRef i) Y. Umemoto, Y. Ie, A. Saeki, S. Seki, S. Tagawa, and Y. Aso, Org. Lett., 2008, 10, 1095; CrossRef j) F. Zhang, and P. Buerle, J. Am. Chem. Soc., 2007, 129, 3090; CrossRef k) K. Sakamoto, Y. Takashima, H. Yamaguchi, and A. Harada, J. Org. Chem., 2007, 72, 459. CrossRef
3. a) Y. Wei, B. Wang, and J. Tian, Tetrahedron Lett., 1995, 36, 665; CrossRef b) J. Hassan, L. Lavenot, C. Gozzi, and M. Lemaire, Tetrahedron Lett., 1999, 40, 857; CrossRef c) K. Tamao, S. Kodama, I. Nakajima, M. Kumada, A. Minato, and K. Suzuki, Tetrahedron, 1982, 38, 3347; CrossRef d) K. Yui, Y. Aso, T. Otsubo, and F. Ogura, Bull. Chem. Soc. Jpn., 1989, 62, 1539; CrossRef e) S. Gronowits, and D. Peters, Heterocycles, 1990, 30, 645; CrossRef f) J. P. Parrish, Y. C. Jung, R. J. Floyd, and K. W. Jung, Tetrahedron Lett., 2002, 43, 7899. CrossRef
4. a) J. Nakayama, T. Konishi, S. Murabayashi, and M. Hoshino, Heterocycles, 1987, 26, 1793; CrossRef b) Z. Xu, D. Fichou, G. Horowitz, and F. Garnier, J. Electronal. Chem., 1989, 267, 339; c) L. Buzhansky and B. A. Feit, J. Org. Chem., 2002, 67, 7523; CrossRef d) P. Buerle, T. Fischer, B. Bidlingmeier, A. Stable, and J. P. Rabe, Angew. Chem., Int. Ed. Engl., 1995, 34, 303. CrossRef
5. M. Takahashi, K. Masui, H. Sekiguchi, N. Kobayashi, A. Mori, M. Funahashi, and N. Tamaoki, J. Am. Chem. Soc., 2006, 128, 10930. CrossRef
6. a) F. Kakiuchi and T. Kochi, Synthesis, 2008, 3013; CrossRef b) G. Dyker, Angew. Chem. Int. Ed., 1999, 38, 1698; CrossRef c) D. Alberico, M. E. Scott, and M. Lautens, Chem. Rev., 2007, 107, 174. CrossRef
7. a) A. Mori and A. Sugie, Bull. Chem. Soc. Jpn., 2008, 81, 548; CrossRef b) L. C. Campeau, D. R. Stuart, and K. Fagnou, Aldrichimica Acta, 2007, 40, 35; c) C. A. Zificsak and D. J. Hlasta, Tetrahedron, 2004, 60, 8991; CrossRef d) T. Satoh and M. Miura, Chem. Lett., 2007, 36, 200; CrossRef e) I. V. Seregin and V. Gevorgyan, Chem. Soc. Rev., 2007, 36, 1173, and references therein. CrossRef
8. a) G. L. Turner, J. A. Morris, and M. F. Greaney, Angew. Chem. Int. Ed., 2007, 46, 7996; CrossRef b) F. Bellina, C. Calandri, S. Cauteruccio, and R. Rossi, Tetrahedron, 2007, 63, 1970; CrossRef c) D. Alagille, R. M. Baldwin, and G. D. Tamagnan, Tetrahedron Lett., 2005, 46, 1349; CrossRef d) Y. Kondo, T. Komine, and T. Sakamoto, Org. Lett., 2000, 2, 3111; CrossRef e) A. L. Gottumukkala and H. Doucet, Eur. J. Inorg. Chem., 2007, 23, 3629; CrossRef f) M. Parisien, D. Valette, and K. Fagnou, J. Org. Chem., 2005, 70, 7578; CrossRef g) S. Pivsa-Art, T. Satoh, Y. Kawamura, M. Miura, and M. Nomura, Bull. Chem. Soc. Jpn., 1998, 71, 467; CrossRef h) H.-Q. Do, R. M. Kashif Khan, and O. Daugulis, J. Am. Chem. Soc., 2008, 130, 15185. CrossRef
9. a) A. Mori, A. Sekiguchi, K. Masui, T. Shimada, M. Horie, K. Osakada, M. Kawamoto, and T. Ikeda, J. Am. Chem. Soc., 2003, 125, 1700; CrossRef b) K. Masui, H. Ikegami, and A. Mori, J. Am. Chem. Soc., 2004, 126, 5074; CrossRef c) K. Masui, A. Mori, K. Okano, K. Takamura, M. Kinoshita, and T. Ikeda, Org. Lett., 2004, 6, 2011; CrossRef d) K. Kobayashi, A. Sugie, M. Takahashi, K. Masui, and A. Mori, Org. Lett., 2005, 7, 5083; CrossRef e) A. Sugie, K. Kobayashi, Y. Suzaki, and K. Osakada, Chem. Lett., 2006, 35, 1100; CrossRef f) K. Kobayashi, M. S. Mohamed Ahmed, and A. Mori, Tetrahedron, 2006, 62, 9548; CrossRef g) N. Arai, M. Takahashi, M. Mitani, and A. Mori, Synlett, 2006, 3170; CrossRef h) J. Shikuma, A. Mori, K. Masui, R. Matsuura, A. Sekiguchi, H. Ikegami, M. Kawamoto, and T. Ikeda, Chem. Asian J., 2007, 2, 301; CrossRef i) A. Mori, J. Shikuma, M. Kinoshita, T. Ikeda, M. Misaki, Y. Ueda, M. Komura, S. Asaoka, and Y. Iyoda, Chem. Lett., 2008, 37, 272; CrossRef j) N. Arai, T. Miyaoku, S. Teruya, and A. Mori, Tetrahedron Lett., 2008, 49, 1000; CrossRef k) T. Miyaoku and A. Mori, Heterocycles, 2009, 77, 151; CrossRef l) H. Furukawa, S. Matsumura, A. Sugie, D. Monguchi, and A. Mori, Heterocycles, 2009, 79, 303. CrossRef
10. a) A. Yokoyama and T. Yokozawa, Macromolecules, 2007, 40, 4093; CrossRef b) A. Yokoyama, R. Miyakoshi, and T. Yokozawa, Macromolecules, 2004, 37, 1169; CrossRef c) E. E. Sheina, J. Liu, M. Corina lovu, D. W. Laird, and R. D. McCullough, Macromolecules, 2004, 37, 3526; CrossRef d) T. A. Chen and R. D. Rieke, J. Am. Chem. Soc., 1992, 114, 10087; CrossRef e) R. Miyakoshi, A. Yokoyama, and T. Yokozawa, J. Am. Chem. Soc., 2005, 127, 17542. CrossRef
11. a) N. Koumura, Z-S. Wang, S. Mori, M. Miyashita, E. Suzuki, and K. Hara, J. Am. Chem. Soc., 2006, 128, 14256; CrossRef b) Z-S. Wang, N. Koumura, Y. M. Takahashi, H. Sekiguchi, A. Mori, T. Kubo, A. Furube, and K. Hara, Chem. Mater., 2008, 20, 3993; CrossRef c) M. Miyashita, K. Sunahara, T. Nishikawa, Y. Uemura, N. Koumura, K. Hara, A. Mori, T. Abe, E. Suzuki, and S. Mori, J. Am. Chem. Soc., 2008, 130, 17874; CrossRef d) N. Koumura, Z-S. Wang, M. Miyashita, Y. Uemura, H. Sekiguchi, Y. Cui, A. Mori, S. Mori and K. Hara, J. Mater. Chem., 2009, 19, 4829; CrossRef e) X-H. Zhang, Z-S. Wang, Y. Cui, N. Koumura, A. Furube, and K. Hara, J. Phys. Chem. C, 2009, 113, 13409. CrossRef
12. a) K. Hara, Y. Tachibana, Y. Ohga, A. Shinpo, S. Suga, K. Sayama, H. Sugihara, and H. Arakawa, Sol. Energy Mater. Sol. Cells, 2003, 77, 89; CrossRef b) K. Hara, M. Kurashige, Y. Dan-oh, C. Kasada, A. Shinpo, S. Suga, K. Sayama, and H. Arakawa, New J. Chem., 2003, 27, 783; CrossRef c) K. Hara, T. Sato, R. Katoh, A. Furube, Y. Ohga, A. Shinpo, S. Suga, K. Sayama, H. Sugihara, and H. Arakawa, J. Phys. Chem. B, 2003, 107, 597; CrossRef d) K. Hara, Y. Dan-oh, C. Kasada, Y. Ohga, A. Shinpo, S. Suga, K. Sayama, and H. Arakawa, Langmuir, 2004, 20, 4205; CrossRef e) K. Hara, T. Sato, R. Katoh, A. Furube, T. Yoshihara, M. Murai, M. Kurashige, S. Ito, A. Shinpo, S. Suga, and H. Arakawa, Adv. Funct. Mater., 2005, 15, 246; CrossRef f) Z.-S. Wang, K. Hara, Y. Dan-oh, C. Kasada, A. Shinpo, S. Suga, H. Arakawa, and H. Sugihara, J. Phys. Chem. B, 2005, 109, 3907; CrossRef g) K. Hara, Z.-S. Wang, T. Sato, A. Furube, R. Katoh, H. Sugihara, Y. Dan-oh, C. Kasada, A. Shinpo, and S. Suga, J. Phys. Chem. B, 2005, 109, 15476; CrossRef h) K. Hara, K. Miyamoto, Y. Abe, and M. Yanagida, J. Phys. Chem. B, 2005, 109, 23776; CrossRef i) Z.-S. Wang, Y. Cui, Y. Dan-oh, C. Kasada, A. Shinpo, and K. Hara, J. Phys. Chem., 2007, 111, 7224; CrossRef j) R. Katoh, A. Huijser, K. Hara, T. J. Savenije, and L. D. A. Siebbeles, J. Phys. Chem. C, 2007, 111, 10741; CrossRef k) Z.-S. Wang, Y. Cui, Y. Dan-oh, C. Kasada, A. Shinpo, and K. Hara, Adv. Mater., 2007, 19, 1138; CrossRef l) Z.-S. Wang, Y. Cui, Y. Dan-oh, C. Kasada, A. Shinpo, and K. Hara, J. Phys. Chem. C, 2008, 112, 17011. CrossRef
13. a) T.-A. Chen, X. Wu, and R. D. Rieke, J. Am. Chem. Soc., 1995, 117, 233; CrossRef b) H. Sirringhaus, P. J. Brown, R. H. Friend, M. M. Nielsen, K. Bechgaard, B. M. W. Langeveld-Voss, A. J. H. Spiering, R. A. J. Janssen, W. W. Meijer, P. Herwig, and D. M. deLeeuw, Nature, 1999, 401, 685. CrossRef
14. Preliminary communication. N. Masuda, S. Tanba, A. Sugie, D. Monguchi, N. Koumura, K. Hara, and A. Mori, Org. Lett., 2009, 11, 2297. CrossRef
15. A. Klapars and S. L. Buchwald, J. Am. Chem. Soc., 2002, 124, 14844. CrossRef
16. a) H. Suzuki, A. Kondo, and T. Ogawa, Chem. Lett., 1985, 14, 411; CrossRef b) Y. Yamashita, K. Suzuki, and M. Tomura, Synth. Met., 2003, 133−134, 341. CrossRef
17. a) X. M. Hong and D. M. Collard, Macromolecules, 2000, 33, 6916; CrossRef b) K. Hoshino, K. Kanie, T. Ohtake, T. Mukai, M. Yoshizawa, S. Ujiie, H. Ohno, and T. Kato, Macromol. Chem. Phys., 2002, 203, 1547; CrossRef c) L. Li, K. E. Counts, S. Kurosawa, A. S. Teja, and D. M. Collard, Adv. Mater., 2004, 16, 180; CrossRef d) A. Facchetti, Y. Myung-Han, L. S. Charlotte, R. H. Geoffrey, A. R. Mark, and J. M. Tobin, J. Am. Chem. Soc., 2004, 126, 13480. CrossRef
18. C. Rocaboy, D. Rutherford, B. L. Bennett, and J. A. Gladysz. J. Phys. Org. Chem., 2000, 13, 596. CrossRef
19. N. Miyaura and A. Suzuki, Chem. Rev., 1995, 7, 2457. CrossRef
20. Naraso and F. Wudl, Macromolecules, 2008, 41, 3169 CrossRef