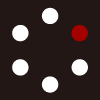
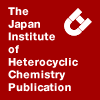
HETEROCYCLES
An International Journal for Reviews and Communications in Heterocyclic ChemistryWeb Edition ISSN: 1881-0942
Published online by The Japan Institute of Heterocyclic Chemistry
e-Journal
Full Text HTML
Received, 11th June, 2010, Accepted, 21st July, 2010, Published online, 22nd July, 2010.
DOI: 10.3987/COM-10-S(E)52
■ Crystal-State Structure Analysis of β-Hydroxy-γ-lactam Constrained Ser/Thr Peptidomimetics
Daniel J. St-Cyr, Thierry Maris, and William D. Lubell*
Department of Chemistry, University of Montreal, CP 6128, Succursale-Centre-Ville
Montreal, Quebec, H3C 3J7, Canada
Abstract
Crystallographic evidence has been obtained in support of the ability of β-hydroxy-α-amino-γ-lactams to induce β-turn conformations within peptides. Two dipeptide model systems of these β-hydroxylated variants of the so-called Freidinger-Veber lactams were prepared from the reaction of N-(Fmoc)oxiranylglycine 2 with methyl m-amino benzoate and methyl lysinate, respectively, and crystallized from benzene or ether. In the solid-state, the lactam moiety was found to adopt dihedral angle geometry similar to extended as well as type II β-turn conformations contingent on the C-terminal amino ester moiety. The β-hydroxyl group of the trans-isomer was shown to point away from the turn suggesting its potential for interaction with receptors in conformationally rigid mimics of serine and threonine-containing peptides.INTRODUCTION†
α-Amino-γ-lactam (Agl), so-called Freidinger-Veber lactam, has served a prominent role in peptide mimicry, because of its ability to locally constrain consecutive ψ- and ω-backbone dihedral angles (Scheme 1).1 Application of Agl residues in constrained mimics has led to a better understanding of the active conformation of biologically relevant peptides, including luteinizing hormone releasing hormone (LH-RH),1,2 angiotensin I and II,2 neurokinin A,2 and cyclodepsipeptide PF1022A.2 Moreover, sequential scanning of peptide sequences has recently been accomplished by employing six-member cyclic sulfamidate 1 as a bis-electrophile to alkylate and subsequently acylate peptide chains on resin (Scheme 1). Employing this Agl scanning technique on growth hormone releasing peptide-6 (GHRP-6) and the interleukin-1 receptor modulator APG101.10 has led to an improved understanding of the active conformer of these peptides as well as to analogs with improved affinity and activity relative to the parent peptides.3,4
The introduction of conformational constraint into a peptide by Agl has the consequence of losing side chain functionality. Considering the importance of serine and threonine side-chain hydroxyl groups (a) in recognition events, (b) in post-translational events such as phosphorylation and glycosylation,5 (c) in stabilization of peptide secondary structures, such as β-turns,6 as well as (d) in the synthesis of other side-chain functionality,7,8 we pursued an approach for the installation of β-hydroxy Agl residues into peptides. In particular, we and others have recently reported that oxiranylglycine is an efficient reagent for the direct installation of β-hydroxy-α-amino-γ-lactams into peptides (Scheme 1).9,10 Employing N-(Fmoc)oxiranylglycine methyl ester 2 in a modified solid-phase peptide synthesis protocol, analogs of APG101.10 were synthesized9 in order to study the consequence of the side-chain geometry of the threonine residue on biological activity, because the resulting β-hydroxy lactam provides additional constraint upon the χ dihedral angle.
Although there have been a number of X-ray structures obtained of compounds bearing β-hydroxy Agl residues,16,17,18,19,20 to the best of our knowledge, these examples feature the lactam within additional ring systems,16,17 or possessing extra substituents at the ring positions,18,19,20 such that difficulty exists in discerning the exact consequence of the hydroxyl group on the conformation of the amino lactam residue. The synthesis, crystallization, and X-ray analysis of two dipeptide models 4a,b (Figure 2), which possess N-terminal β-hydroxy Agl residues, is now reported to provide the first evidence of the influence of the β-hydroxy group on the conformation of a γ-lactam peptide in the solid state. Crystallographic analysis of the β-hydroxy-α-amino-γ-lactam peptides 4 demonstrated that a linear conformation as well as type II β-turns may be adopted contingent on the C-terminal residue and stereochemistry.
EXPERIMENTAL
Protected hydroxy-lactam dipeptides 4 were synthesized by reaction of N-(Fmoc)oxiranylglycine with methyl m-amino benzoate and methyl lysinate, respectively, as previously reported.9 The slow evaporation of solutions of 4a in benzene and 4b in diethyl ether provided, respectively, crystals suitable for X-ray analysis. Upon isolation, the crystals were collected in paratone-N oil and placed under a 150 K nitrogen stream on the goniometer head of the diffractometer. X-ray crystallographic data were collected from a single crystal sample, which was mounted on a loop fiber. Data were collected using the program APEX2 on a Bruker SMART 6000 diffractometer, equipped with a Nonius FR591 rotating anode, a Charged-Coupled Device (CCD) Area Detector and a Montel 200 optics producing CuKα radiation. The structures were solved by direct methods.21 All non-hydrogen atoms were refined anisotropically while hydrogen atoms were treated as idealized contributions (Riding model). The crystals obtained from 4a included a guest benzene molecule while for 4b, the fluorenylmethyl moiety was refined with a twofold disorder.
RESULTS AND DISCUSSION
The crystal structures of dipeptide mimics 4a and 4b and their physical properties are presented in Figure 3 and Table 1. The C2-C5 fragment of 4a had a threo configuration, supporting our previously assumed diastereoselectivity upon installation of the C4 stereocenter. Given that the C3 stereocenter derives from D-Met, the absolute stereochemistry of 4a is illustrated as (3R,4S). A reasonably low Flack parameter22 of 0.2(2) (Table 1) supports this assignment and suggests that C3 stereochemical integrity is maintained. A linear conformation was observed in the solid-state structure of 4a.
In the crystal structure of hydroxy lactam dipeptide 4b, a turn geometry may be inferred from the 5.3 Å heavy atom distance between the Fmoc oxygen and the methyl carbon of the ester (Figure 4). This distance compares well to the Cαi-Cαi+3 distance of less than 7 Å, which is a requirement of β-turn conformations. Similar to (R)-Agl, which has been shown to adopt a type II β-turn in peptides,14,15 (3R,4S)-4-hydroxy-γ-lactam 4b exhibited dihedral angle parameters within (± 30°) the range of an ideal type II β-turn (Table 2).6 The ψ i+2 dihedral angle of 4b deviated significantly from that found in an ideal β-turn, likely because the ester moiety cannot participate in the conventional i ← i + 3 hydrogen bond. Instead, an n→π* Coulomb interaction between the Fmoc-carbonyl oxygen and ester carbonyl carbon may operate to rotate the ester moiety over 90° such that a Burgi-Dunitz trajectory of attack (104.6° vs ideal 107°) is adopted.23 A similar interaction has also been noted in the crystal-state structure of a 4-fluoroproline derivative.24 The structural similarity between the conformations adopted by D-Agl and its β-hydroxy-γ-lactam counterpart demonstrates that the (S)-hydroxyl group does not perturb the normal preferences of the amino lactam moiety.
The pyrrolidine ring in Agl is sufficiently flexible such that it may access several different puckering conformations. With the added 4-hydroxyl group, these conformations gain importance as they relate to the χ dihedral angle of the side chain. In the cyclopentane model, two stable ring puckers are respectively defined as the “twist” conformation, in which two adjacent atoms lie above and below the plane containing the remaining three ring atoms, and the “envelope” conformation, in which one ring atom lies above the plane containing the remaining four atoms.25 In these ideal conformations, 1-2 intracylic dihedral angles fall between 40-42°.25 In the solid state, the pyrrolidine ring of the Agl residue has displayed envelope, twist, and intermediate conformations, which were significantly flattened relative to a cyclopentane ring, with the largest dihedral angles falling in the 8-27° range,12-15 due to the planar amide bond. Likewise, 4-hydroxy-γ-lactams 4a,b displayed, respectively, a flattened envelope and twist conformation (largest dihedral angles: 25.9° and 28.6°). The β carbon of Agl and 4-hydroxy-γ-lactam is puckered in all cases, with additional puckering of the α or γ carbons for twist-like conformations.12-15 The envelope conformation in the case of 4a might be enforced due to donation of the nitrogen lone pair into the periplanar aromatic ring. The lengthened N-C(2) bond and shortened C(2)=O bond in 4a relative to 4b support such an interaction, resulting in loss of pyramidalization at nitrogen for 4a (the sum of angles about N in 4a was slightly higher than that of 4b: 360° vs. 357.9°). Overall, the introduction of a hydroxyl group does not appear to have any influence on the conformational preferences of the pyrrolidinone ring in Agl. Similarly, more highly substituted and ring fused derivatives of hydroxy lactams have also shown the same types of puckering.16-20
As puckering occurs at the β carbon, the hydroxyl group of α-amino-β-hydroxy-γ-lactams can be oriented equatorially or axially depending on the direction of pucker. The direction of pucker may be described as Cβ-endo vs. Cβ-exo relative to the α-amino group, both of which are represented by 4a and 4b, respectively (Figure 5).26 In the context of the β-turn like conformation of the latter, the Cβ-exo pucker causes an axial orientation of the hydroxyl group such that it points perpendicularly outward from the plane of the β-turn. As both dipeptides display D-threo stereochemistry, the observed difference in the N2-C3-C4-O2 torsion angles (i.e. χ dihedral angles)27 of 89.5° and 142.4° for 4a and 4b respectively are primarily a consequence of the Cβ-endo vs. Cβ-exo ring puckering of the pyrrolidine core.
In conclusion, the first crystallographic evidence has demonstrated that β-hydroxy Agl may induce a β-turn conformation in the solid state; however, a linear conformation was also identified. The accessible conformations of β-hydroxy lactams appear to be analogous to those of traditional Freidinger-Veber lactams.
ACKNOWLEDGEMENTS
Financial support is gratefully acknowledged from the Natural Sciences and Engineering Research Council of Canada, le Fond Québécois de la Recherche sur la Nature et les Technologies, and the Canadian Institutes of Health Research Team Grant Program (funding no. CTP79848) in G-Protein Coupled Receptor Allosteric Regulation.
SUPPORTING INFORMATION AVAILABLE
CCDC 779469 and 779470 contain the supplementary crystallographic data for this paper. These data can be obtained free of charge from The Cambridge Crystallographic Data Centre via www.ccdc.cam.ac.uk/data_request/cif. Copies of the data can be obtained free of charge on application to the Director, CCDC, 12 Union Road, HETEROCYCLES, Vol. 82, 2010, XXX Cambridge CB2 1EZ, UK (Fax: +44 1223 336 033; e-mail:deposit@ccdc.cam.ac.uk).
References
1. R. M. Freidinger, J. Med. Chem., 2003, 46, 5553. CrossRef
2. A. Perdih and D. Kikelj, Curr. Med. Chem., 2006, 13, 1525. CrossRef
3. A. G. Jamieson, N. Boutard, K. Beauregard, M. S. Bodas, H. Ong, C. Quiniou, S. Chemtob, and W. D. Lubell, J. Am. Chem. Soc., 2009, 131, 7917. CrossRef
4. N. Boutard, A. G. Jamieson, H. Ong, and W. D. Lubell, Chem. Biol. Drug Des., 2010, 75, 40. CrossRef
5. (a) Glycosylation review: P. V. D. Steen, P. M. Rudd, R. A. Dwek, and G. Opdenakker, Crit. Rev. Biochem. Mol. Biol., 1998, 33, 151; CrossRef (b) Phosphorylation review: L. A. Pinna and M. Ruzzene, Biochim. Biophys. Acta, 1996, 1314, 191.
6. E. G. Hutchinson and J. M. Thornton, Protein Sci., 1994, 3, 2207. CrossRef
7. R. F. W. Jackson, ‘ACS Symposium Series: Asymmetric Synthesis and Application of α-Amino-Acids,’ Vol. 1009, ed. by V. A. Soloshonok, and K. Lzawa, American Chemical Society, Washington, DC, 2009, pp. 342-6.
8. T. Ohishi, M. Yamada, and M. Sugawara, ‘ACS Symposium Series: Asymmetric Synthesis and Application of α-Amino-Acids,’ Vol. 1009, ed. by V. A. Soloshonok and K. Lzawa, American Chemical Society, Washington, DC, 2009, pp. 2-4.
9. D. J. St-Cyr, A. G. Jamieson, and W. D. Lubell, Org. Lett., 2010, 12, 1652. CrossRef
10. F. Sicherl, T. Cupido, and F. Albericio, Chem. Commun., 2010, 46, 1266. CrossRef
11. P. K. C. Paul, P. A. Burney, M. M. Campbell, and D. J. Osguthorpe, J. Comput.-Aided Mol. Des., 1990, 4, 239. CrossRef
12. G. Valle, M. Crisma, C. Toniolo, K.-L. Yu, and R. L. Johnson, Int. J. Pept. Protein Res., 1989, 33, 181. CrossRef
13. G. Valle, M. Crisma, C. Toniolo, K.-L. Yu, and R. L. Johnson, J. Chem. Soc., Perkin Trans. 2, 1989, 83. CrossRef
14. M. C. Evans, A. Pradhan, S. Venkatraman, W. H. Ojala, W. B. Gleason, R. K. Mishra, R. L. Johnson J. Med. Chem,. 1999, 42, 1441. CrossRef
15. P. W. Baures, W. H. Ojala, W. B. Gleason, R. K. Mishra, and R. L. Johnson, J. Med. Chem., 1994, 37, 3677. CrossRef
16. J. H. Bieri, H. Heimgartner, G. Germain, and J. P. Declercq, Acta Crystallogr., Sect. C: Cryst. Struct. Commun., 1983, 39, 1064. CrossRef
17. A. Ilyin, V. Kysil, M. Krasavin, I. Kurashvili, and A. V. Ivachtchenk, J. Org. Chem., 2006, 71, 9544. CrossRef
18. C. Alvarez-Ibarra, A. G. Csaky, E. Martinez-Santos, M. L. Quiroga, and J. L.Tejedor, Tetrahedron, 1999, 55, 3041. CrossRef
19. S. Sauer, A. Schumacher, F. Barbosa, and B. Giese, Tetrahedron Lett., 1998, 39, 3685. CrossRef
20. J. J. Leban and K. L. Colson, J. Org. Chem., 1996, 61, 228. CrossRef
21. G. M. Sheldrick, Acta Cryst, 2008, A64, 112.
22. H. D. Flack and G. Bernardinelli, J. Applied Cryst., 2000, 33, 1143. CrossRef
23. H. B. Bürgi, J. D. Dunitz, J. M. Lehn, and G. Wipff, Tetrahedron, 1974, 30, 1563. CrossRef
24. M. L. DeRider, S. J. Wilkens, M. J. Waddell, L. E. Bretscher, F. Weinhold, R. T. Raines, and J. L. Markley, J. Am. Chem. Soc., 2002, 124, 2497. CrossRef
25. H. J. Geise, C. Altona, and C. Romers, Tetrahedron Lett., 1967, 8, 1383. CrossRef
26. This ring puckering nomenclature was adapted for the 3-amino-pyrrolidine ring in the context of this manuscript from that used for proline derivatives.24.
27. V. J. Hruby, G. Li, C. Haskell-Luevano, and M. Shenderovich, Biopolymers, 1997, 43, 219 CrossRef