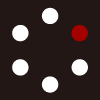
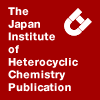
HETEROCYCLES
An International Journal for Reviews and Communications in Heterocyclic ChemistryWeb Edition ISSN: 1881-0942
Published online by The Japan Institute of Heterocyclic Chemistry
e-Journal
Full Text HTML
Received, 3rd May, 2010, Accepted, 7th June, 2010, Published online, 8th June, 2010.
DOI: 10.3987/COM-10-S(E)25
■ An Efficient Synthesis of Methyl Esters of Heterocyclic α,β-Didehydro-α-amino Acid Derivatives
Franc Požgan, Klavdija Lukman, and Marijan Kočevar*
Department of Organic Chemistry, Faculty of Chemistry and Chemical Technology, University of Ljubljana, Askerceva 5, P.O. Box 537, SI-1000 Ljubljana, Slovenia
Abstract
N-Benzoyl-protected α,β-didehydro-α-amino acid (DDAA) derivatives containing a pyrazole or an isoxazole ring at the β-position were transformed with diazomethane into the corresponding methyl esters. Applying this method, the double C=C bond of the DDAA derivatives was not affected, thus selectively giving the corresponding esters in good-to-excellent yields.INTRODUCTION
Non-proteinogenic α-amino acids can exhibit peculiar properties once incorporated into peptides, and are attracting increasing interest from researchers in the fields of organic chemistry, medicinal chemistry, and protein engineering.1 From among them, the DDAAs2 have received particular attention since they constitute many naturally occurring peptides that show important biological properties.3 For example, lantibiotic peptides, as a group of potent antibacterial agents, contain some unusual amino acids and DDAAs, such as didehydroalanine or didehydroaminobutyric acid.4 The incorporation of DDAA into peptide sequences introduces conformational constraints, making these peptides models in the study of enzyme-binding mechanisms.5 Thus, one of the major goals in modern amino acid chemistry is to develop conformationally restricted amino acid derivatives that may lead to enhanced biological activity by decreasing the degree of freedom of the peptide into which they are incorporated. Moreover, the asymmetric hydrogenation of DDAA derivatives would provide a simple and relatively efficient method for the preparation of optically active natural and unnatural amino acid derivatives6 for applications in a variety of fields.
2H-pyran-2-ones containing the 3-amino functionality (protected or not), and which are easily prepared by simple one-pot methods,7 can be regarded as the cyclic analogues of DDAA derivatives. As a part of our investigations of the transformation of the 2H-pyran-2-one derivatives with different nucleophilic reagents,2d we have developed a straightforward method for the preparation of novel types of DDAA derivatives containing a substituted pyrazole, isoxazole or pyrimidine ring at the β-position, starting from 5-acyl-3-benzoylamino-2H-pyran-2-ones and substituted hydrazines, hydroxylamines or amidines.8 These reactions led mainly to the formation of heterocyclic DDAA derivatives with an (E)-configuration around the C=C double bond; in some cases, however, mixtures of (E)- and (Z)-isomers were obtained. In this paper we present a selective transformation of pyrazole- or isoxazole-bearing DDAA derivatives with diazomethane that afforded the corresponding methyl esters as the sole products.
RESULTS AND DISCUSSION
The previously described reactions of 5-acetyl- and 5-benzoyl-3-benzoylamino-6-methyl-2H-pyran-2-one with hydroxylamine gave mixtures of the (E)- and (Z)-β-isoxazolyl-DDAA derivatives (1a) and (1b) (Scheme 1, Table 1), which were not completely separable by ordinary chromatographic techniques due to the close Rf factors.8c The structures of their minor components were proposed on the basis of a comparison of the 1H and 13C NMR chemical shifts of a series of DDAA derivatives as well as on the basis of 2D-NMR experiments performed with mixtures of (E/Z)-1a and (E/Z)-1b. We wanted to improve the possibility of separation of the above mixtures by transforming them into their appropriate derivatives. Therefore, we decided to prepare their methyl esters, for which we believed they would be more easily separable.8d We decided to use diazomethane for this purpose.9 Diazomethane is known to react very well with carboxylic acids to give their esters, but its 1,3-dipolar cycloadditions with C=C double bonds have also been documented.10 It is interesting to note that in the literature there are only very few examples of the preparation of DDAA methyl esters from the corresponding free acids by a reaction with diazomethane.11 On the other hand, it has been shown that DDAA derivatives can react with diazomethane, in addition to their esterification, also with the C=C double bond to give the corresponding pyrazolines or their decomposition products.12 On this basis, in our case we cannot exclude the formation of rather complex mixtures of products. However, the reaction of an excess of diazomethane (used as a solution in diethyl ether) with the 70/30 mixture of (E/Z)-1a gave a mixture of the corresponding methyl esters (E/Z)-(2a) in the same ratio. This allowed us to separate the (E)- and (Z)-isomers by a partial crystallization and radial chromatography, and then unequivocally to confirm the structures of the starting acids. On the other hand, we were able to isolate only the major product (Z)-(2b) in a 38% yield by radial chromatography of the reaction mixture of (E/Z)-2b esters due to the small amount of (E)-isomer in the mixture. Since the reactions in these two cases were very selective and yielded esters as the sole products, we then decided to examine the action of diazomethane on a series of DDAA derivatives containing the pyrazolyl moiety at the β-position. Thus, compounds (1c–o) were treated with diazomethane in short reaction times (0.5–2 h) and successfully transformed into the corresponding methyl esters (2c–o). After the completion of the reaction, the reaction mixture was evaporated, resulting in an oily residue, which was treated in different ways so as to isolate the product. The products (2c–g), (2i–l) and (2o) were simply precipitated by the addition of petroleum ether or diethyl ether to the evaporated reaction mixtures and isolated in 73–98% yields. The product (2h) was isolated by crystallization from ethanol in a 56% yield. The reaction of the acid (1m) containing an additional carboxylic functionality at the phenyl moiety attached to the pyrazole ring resulted in the ester (2n), with both carboxylic groups being methylated; this product was isolated in a 66% yield after purification on a chromatotron. In the 1H NMR spectra of all the isolated products (2) no additional signals were observed that could correspond to the eventually formed 1,3-cycloadducts, thus revealing that this method clearly gave only the products with the methylated carboxylic group.
The resonance signals for the 3-H protons of pairs of the methyl esters (2) and the starting acids (1) had practically the same chemical shift values (the maximum difference being 0.04 ppm) indicating the same configuration of C=C double bond of the esters and the starting acids. Additionally, as expected,8b−d the (Z)-products (Z)-(2a) and (Z)-(2b) show a downfield shift for the 3-H of about 0.5 ppm with respect to their (E)-isomers (6.43 vs. 6.94 ppm in the case of (E)- and (Z)-2a).
Interesting results were obtained in the 24-hour reaction of the compound (1p) with an excess of diazomethane. Namely, after the separation of the reaction mixture by radial chromatography, three products were isolated. One of them was identified as the expected methyl ester (2p). A careful NMR investigation of the other two revealed the regioisomeric structures (2o) and (2r), originating from additional methylation of the pyrazole nitrogen atoms.
In the 1H NMR spectrum of the compound (2p), recorded in DMSO-d6 at 29 ºC, we observed two slightly separated singlets at 2.10 and 2.15 ppm for the protons of the methyl group on the pyrazole ring, whereas for the pyrazole N-H proton, two broad singlets (12.78 and 12.94 ppm) of unequal intensities were found. We assumed that the double signals originated from the tautomerism of the pyrazole ring (Figure 1), which was supported by evidence in the literature.13 When the 1H NMR spectrum of 2p was recorded at 80 ºC the signals for the methyl protons coalesced to form a broad singlet; the same also happened with the two N-H signals. This tautomerism can also explain the formation of the two products (2o) and (2r). Namely, each of the two tautomeric forms (2p and 2p') was trapped by diazomethane, yielding the N-methylated regioisomeric products (2o) and (2r), respectively.
The structures of the compounds (2o) and (2r) were established on the basis of their NMR spectroscopic data. The resonance signals in their 1H NMR spectra differed only slightly in terms of the chemical shift values of the pyrazole moiety, thus pointing to structures with two different arrangements of methyl and phenyl groups attached to the pyrazole ring. We have previously shown that heterocyclic didehydroamino acids, regioisomeric in the pyrazole ring, can be differentiated on the basis of the 13C chemical shifts of the 3'-C and 5'-C carbon atoms of the pyrazole ring.8b−d Namely, the resonance signal for the 3'-C was always shifted downfield with regard to the signal for the 5'-C. In the 1H-13C HMBC spectrum of the compound (2o), the ortho protons of the phenyl group attached to the pyrazole ring correlated with the carbon atom at 147.0 ppm, revealing the position of this carbon to be 3'-C. Furthermore, the protons of both methyl groups on the pyrazole ring correlated with the carbon at 138.2 ppm (5'-C). Additionally, a NOE difference experiment with the compound (2o) was carried out. Saturation of the 5'-Me (2.15 ppm) signal showed a slight enhancement (3.3%) on the resonance of the 1'-Me (3.79 ppm), indicating the spatial proximity of both methyl groups attached to the pyrazole ring. On the other hand, the HMBC spectrum of the compound (2r) revealed that the pyrazole carbon atom bearing the phenyl moiety was 5'-C (141.4 ppm). The protons of the methyl group attached at position 1 (N-Me) also correlated with this carbon atom (5'-C), while the protons of the second methyl group correlated with the carbon atom at 144.8 ppm (3'-C). The structure of the compound (2o) was further confirmed by an independent synthesis of the methyl ester from the acid (1o).
To exclude any eventual isomerization during the reaction, the configuration of the C2,C3 double bond of some products was also determined on the basis of their NOESY spectra, which were taken for the compounds (E)-(1d), (E)-(2a), (Z)-(2a), (Z)-(2b), (E)-(2i), (E)-(2j), (E)-(2o), (E)-(2p) and (E)-(2r). In the case of the E-products we observed a NOE between the PhCONH and 3-H, while it was not observed in the case of the Z-products.
In conclusion, we have presented a simple and general method for the preparation of the methyl esters of α,β-didehydro-α-amino acids containing a pyrazole or isoxazole ring at the β-position. By the application of an excess of diazomethane only the carboxylic group was methylated, while the C=C double bond was not affected. After a prolonged reaction time the pyrazole NH groups can also react, yielding N-methylated products as a result of pyrazole tautomerism. In contrast to some previously described transformations of differently substituted DDAA derivatives with diazomethane,12 the formation of N-methylated products is evidence of a high resistance of the C=C double bond of our derivatives to dipolar cycloaddition; even N-methylation occurs more easily than the addition to the C=C double bond. It is not easy to explain such behavior of our DDAA derivatives. One of the reasons for the resistance of the C=C double bond might be its conjugation with the heterocyclic ring or eventual steric hindrances within the compound. The formation of DDAA esters was also confirmed as a tool for the separation of DDAA derivatives.
EXPERIMENTAL
Acids (1) were prepared by previously described methods.8a-d Diazomethane was prepared as described in the literature.9 All other reagents and solvents were used as obtained from commercial suppliers.
Melting points were determined on a Kofler micro hot stage, and are uncorrected. NMR spectra were recorded at 29 ºC with a Bruker Avance DPX 300 in DMSO-d6. 1H NMR spectra were recorded at 300 MHz using TMS as an internal standard. 13C NMR spectra were recorded at 75.5 MHz and are referenced against the central line of the residual DMSO (present in DMSO-d6) septet at 39.5 ppm. The coupling constants J are given in Hz. IR spectra were obtained with a Bio-Rad FTS 300MX. MS spectra were recorded with a VG-Analytical AutoSpec Q instrument. Elemental analyses (C, H, N) were performed with Perkin-Elmer 2400 CHN and CHNS/O Analyzers. TLC was carried out on Fluka silica gel TLC cards. Merck silica gel 60 PF254 containing gypsum was used to prepare chromatotron plates.
(E)-2-(Benzoylamino)-3-(1,3,5-trimethyl-1H-pyrazol-4-yl)propenoic acid (E-1d). A mixture of 5-acetyl-3-benzoylamino-6-methyl-2H-pyran-2-one (542 mg, 2 mmol) and methylhydrazine (102 mg, 2.2 mmol) in a mixture of absolute ethanol (8 mL) and pyridine (2 mL) was stirred at room temperature for 50 min. The solvent was removed in vacuo and water (8 mL) was added to the residue. The pH of the resulting mixture was adjusted to 2 by 9% hydrochloric acid. Upon cooling the solid material was filtered off and washed with a small amount of water to give 1d (587 mg, 98%).
White solid; mp 180–183 °C (EtOAc). IR (KBr) νmax/cm-1: 3292 br, 1659, 1528. 1H NMR (300 MHz): δ 2.03 (s, 3H, Me), 2.11 (s, 3H, Me), 3.64 (s, 3H, Me), 6.53 (s, 1H, 3-H), 7.52 (m, 3H, Ph), 7.93 (m, 2H, Ph), 10.12 (s, 1H, NH), 12.49 (br s, 1H, OH). 13C NMR (75.5 MHz): δ 10.3, 12.5, 35.7, 112.1, 118.5, 127.6, 128.2, 128.4, 131.7, 133.5, 137.3, 144.6, 165.2, 166.1. MS-EI: m/z (%) = 299 (M+, 52), 105 (100). Anal. Calcd for C16H17N3O3: C, 64.20; H, 5.72; N, 14.04. Found: C, 64.22; H, 5.81; N, 13.97.
General procedure for the preparation of the esters (2). A solution of diazomethane in diethyl ether (30 mL) was added dropwise into the stirred solution of 1 (2 mmol) in MeOH (5–15 mL). After stirring at room temperature for the time indicated in Table 1, the reaction mixture was evaporated under reduced pressure. The oily residue was further treated as follows.
Methyl (E/Z)-2-(benzoylamino)-3-(3,5-dimethylisoxazol-4-yl)propenoate (E/Z-2a). The evaporated residue was crystallized from MeOH to give, after filtration, E-2a (23%). The filtrate was separated on a chromatotron (petroleum ether–EtOAc, 3:1) to give an additional amount of E-2a (13%) and Z-2a (17%).
Data for E-2a: White solid; mp 161–164 °C (MeOH). IR (KBr) νmax/cm-1: 3250 br, 1724, 1648. 1H NMR (300 MHz): δ 2.12 (s, 3H, Me), 2.25 (s, 3H, Me), 3.64 (s, 3H, OMe), 6.43 (s, 1H, 3-H), 7.53 (m, 2H, Ph), 7.61 (m, 1H, Ph), 7.94 (m, 2H, Ph), 10.55 (s, 1H, NH). 13C NMR (75.5 MHz): δ 10.0, 11.4, 52.1, 110.1, 112.0, 127.7, 128.5, 131.9, 132.1, 132.7, 158.6, 164.4, 165.3, 165.7. MS–EI: m/z (%) = 300 (M+, 18), 105 (100). Anal. Calcd for C16H16N2O4: C, 63.99; H, 5.37; N, 9.33. Found C, 63.78; H, 5.33; N, 9.05.
Data for Z-2a: White solid; mp 153.5–156.5 °C (MeOH). IR (KBr) νmax/cm-1: 3243 br, 1721, 1643. 1H NMR (300 MHz): δ 2.12 (s, 3H, Me), 2.21 (s, 3H, Me), 3.73 (s, 3H, OMe), 6.94 (s, 1H, 3-H), 7.53 (m, 3H, Ph), 7.90 (m, 2H, Ph), 9.97 (s, 1H, NH). 13C NMR (75.5 MHz): δ 10.4, 12.0, 52.2, 110.3, 119.9, 127.6, 128.5, 129.4, 132.0, 132.9, 158.8, 164.7, 166.0, 167.1. MS-EI: m/z (%) = 300 (M+, 10), 105 (100). Anal. Calcd for C16H16N2O4: C, 63.99; H, 5.37; N, 9.33. Found C, 63.93; H, 5.49; N, 9.15.
Methyl (Z)-2-(benzoylamino)-3-(3-methyl-5-phenyl-isoxazol-4-yl)propenoate (Z-2b). The evaporated residue was purified on a chromatotron (petroleum ether–EtOAc, 3:1) to give Z-2b.
Yellowish solid; mp 135–137 °C (EtOH). IR (KBr) νmax/cm-1: 3264 br, 1732, 1670. 1H NMR (300 MHz): δ 2.16 (s, 3H, Me), 3.75 (s, 3H, OMe), 7.00 (s, 1H, 3-H), 7.40 (m, 5H, Ph), 7.52 (m, 1H, Ph), 7.64 (m, 4H, Ph), 9.87 (s, 1H, NH). 13C NMR (75.5 MHz): δ 10.4, 52.3, 109.6, 118.5, 126.6, 127.3, 127.5, 128.1, 128.9, 130.3, 131.1, 131.8, 132.8, 159.5, 164.7, 165.4, 165.6. MS–EI: m/z (%) = 362 (M+, 4), 105 (100). Anal. Calcd for C21H18N2O4: C, 69.60; H, 5.01; N, 7.37. Found C, 69.53; H, 5.01; N, 7.69.
Methyl (E)-2-(benzoylamino)-3-(3,5-dimethyl-1H-pyrazol-4-yl)propenoate (E-2c). After the addition of Et2O (8 mL) to the evaporated residue E-2c was filtered off.
White solid; mp 153–156 °C (MeOH/H2O). IR (KBr) νmax/cm-1: 3326, 3277 br, 1725, 1640, 1613, 1574, 1531. 1H NMR (300 MHz): δ 2.05 (br s, 6H, two Me), 3.62 (s, 3H, OMe), 6.52 (s, 1H, 3-H), 7.55 (m, 3H, Ph), 7.93 (m, 2H, Ph), 10.33 (s, 1H, NH), 12.28 (br s, 1H, NH). 13C NMR (75.5 MHz): δ 10.0, 12.2, 51.8, 110.8, 118.5, 126.9, 127.7, 128.4, 131.9, 133.1, 137.3, 144.5, 165.26, 165.28. MS–EI: m/z (%) = 299 (M+, 32), 105 (100). Anal. Calcd for C16H17N3O3: C, 64.20; H, 5.72; N, 14.04. Found C, 63.95; H, 5.89; N, 13.94.
Methyl (E)-2-(benzoylamino)-3-(1,3,5-trimethyl-1H-pyrazol-4-yl)propenoate (E-2d). After the addition of petroleum ether (8 mL) to the evaporated residue E-2d was filtered off.
Off-white solid; mp 58–61 °C (petroleum ether/EtOAc). IR (KBr) νmax/cm-1: 3255 br, 1721, 1661. 1H NMR (300 MHz): δ 1.99 (s, 3H, 3'-Me), 2.09 (s, 3H, 5'-Me), 3.62 (s, 3H, OMe), 3.65 (s, 3H, 1'-Me), 6.52 (s, 1H, 3-H), 7.56 (m, 3H, Ph), 7.93 (m, 2H, Ph), 10.34 (s, 1H, NH). 13C NMR (75.5 MHz): δ 10.0, 12.2, 35.7, 51.8, 111.6, 118.4, 127.1, 127.6, 128.4, 131.9, 133.0, 137.3, 144.4, 165.1, 165.2. MS–EI: m/z (%) = 313 (M+, 73), 105 (100). HRMS–EI: m/z (M+) calcd for C17H19N3O3 313.1426; found: 313.1430. Anal. Calcd for C17H19N3O3×⅓H2O: C, 63.94; H, 6.21; N, 13.16. Found C, 64.05; H, 6.62; N, 13.16.
Methyl (E)-2-(benzoylamino)-3-(1-tert-butyl-3,5-dimethyl-1H-pyrazol-4-yl)propenoate (E-2e). After the addition of Et2O (8 mL) to the evaporated residue E-2e was filtered off.
White solid; mp 116–119 °C (EtOH/H2O). IR (KBr) νmax/cm-1: 3292 br, 2982, 1727, 1643, 1520. 1H NMR (300 MHz): δ 1.55 (s, 9H, t-Bu), 1.98 (s, 3H, Me), 2.25 (s, 3H, Me), 3.60 (s, 3H, OMe), 6.52 (s, 1H, 3-H), 7.54 (m, 3H, Ph), 7.93 (m, 2H, Ph), 10.33 (s, 1H, NH). 13C NMR (75.5 MHz): δ 12.6, 13.0, 29.7, 51.7, 59.2, 113.7, 118.5, 127.6, 127.8, 128.4, 131.9, 133.1, 136.4, 142.6, 165.0, 165.2. MS–EI: m/z (%) = 355 (M+, 43), 105 (100). Anal. Calcd for C20H25N3O3: C, 67.58; H, 7.09; N, 11.82. Found C, 67.55; H, 7.30; N, 11.65.
Methyl (E)-2-(benzoylamino)-3-(1-benzyl-3,5-dimethyl-1H-pyrazol-4-yl)propenoate (E-2f). After the addition of petroleum ether (8 mL) to the solid residue E-2f was filtered off.
White solid; mp 33–36 °C (petroleum ether). IR (KBr) νmax/cm-1: 3267 br, 1726, 1659. 1H NMR (300 MHz): δ 2.04 (s, 3H, Me), 2.06 (s, 3H, Me), 3.57 (s, 3H, OMe), 5.24 (s, 2H, CH2), 6.55 (s, 1H, 3-H), 7.09 (m, 2H), 7.30 (m, 3H), 7.54 (m, 3H) and 7.93 (m, 2H) (two Ph), 10.36 (s, 1H, NH). MS–EI: m/z (%) = 389 (M+, 35), 91 (100). HRMS-EI: m/z (M+) calcd for C23H23N3O3 389.1739; found: 389.1750.
Methyl (E)-2-(benzoylamino)-3-(3,5-dimethyl-1-phenyl-1H-pyrazol-4-yl)propenoate (E-2g). After the addition of petroleum ether (8 mL) to the solid residue E-2g was filtered off.
Brownish solid; mp 138–140 °C (MeOH/H2O). IR (KBr) νmax/cm-1: 3281, 1734, 1643. 1H NMR (300 MHz): δ 2.12 (s, 3H, Me), 2.19 (s, 3H, Me), 3.67 (s, 3H, OMe), 6.60 (s, 1H, 3-H), 7.38–7.65 (m, 8H) and 7.95 (m, 2H) (two Ph), 10.43 (s, 1H, NH). 13C NMR (75.5 MHz): δ 11.8, 12.4, 51.9, 113.9, 117.2, 124.1, 127.2, 127.7, 128.4, 128.6, 129.1, 131.9, 133.0, 137.2, 139.2, 147.0, 165.0, 165.3. MS–EI: m/z (%) = 375 (M+, 65), 105 (100). Anal. Calcd for C22H21N3O3: C, 70.38; H, 5.64; N, 11.19. Found C, 70.41; H, 5.79; N, 10.94.
Methyl (E)-2-(benzoylamino)-3-[1-(imidazo[1,2-b]pyridazin-6-yl)-3,5-dimethyl-1H-pyrazol-4-yl]propenoate (E-2h). The solid residue was crystallized from EtOH to give E-2h.
White solid; mp 125–127.5 °C (EtOH). IR (KBr) νmax/cm-1: 3273 br, 1728, 1662. 1H NMR (300 MHz): δ 2.18 (s, 3H, Me), 2.51 (s, 3H, Me), 3.65 (s, 3H, OMe), 6.61 (s, 1H, 3-H), 7.51–7.65 (m, 3H, Ph), 7.73 (d, J = 9.8 Hz, 1H, 7"-H), 7.81 (d, J = 1.2 Hz, 1H, 2"-H), 7.96 (m, 2H, Ph), 8.25 (dd, 1H, J1 = 9.8 Hz, J2 = 0.8 Hz, 8"-H), 8.295 (dd, 1H, J1 = 1.2 Hz, J2 = 0.8 Hz, 3"-H), 10.52 (s, 1H, NH). 13C NMR (75.5 MHz): δ 12.4, 13.0, 52.0, 113.3, 115.5, 116.3, 117.6, 127.4, 127.7, 128.5, 130.4, 132.0, 132.9, 134.1, 137.2, 138.8, 148.6, 149.2, 164.7, 165.3. MS–EI: m/z (%) = 416 (M+, 23), 105 (100). HRMS–EI: m/z (M+) calcd for C22H20N6O3 416.1597; found: 416.1605.
Methyl (E)-2-(benzoylamino)-3-(3-methyl-1,5-diphenyl-1H-pyrazol-4-yl)propenoate (E-2i). After the addition of petroleum ether (8 mL) to the evaporated residue E-2i was filtered off.
Brownish solid; mp 143.5–148 °C (MeOH/H2O). IR (KBr) νmax/cm-1: 3264 br, 1738, 1641. 1H NMR (300 MHz): δ 2.17 (s, 3H, Me), 3.50 (s, 3H, OMe), 6.44 (s, 1H, 3-H), 7.18 (m, 4H), 7.33 (m, 6H), 7.49 (m, 2H), 7.57 (m, 1H) in 7.90 (m, 2H) (three Ph), 10.36 (s, 1H, NH). 13C NMR (75.5 MHz): δ 12.5, 51.7, 114.7, 116.1, 124.6, 127.1, 127.7, 128.40, 128.45, 128.49, 128.6, 128.8, 129.6, 129.9, 132.0, 132.8, 139.4, 140.8, 147.2, 164.6, 165.1. MS–EI: m/z (%) = 437 (M+, 17), 105 (100). Anal. Calcd for C27H23N3O3: C, 74.13; H, 5.30; N, 9.60. Found C, 74.10; H, 5.34; N, 9.75.
Methyl (E)-2-(benzoylamino)-3-[3-methyl-1-(2-methylphenyl)-5-phenyl-1H-pyrazol-4-yl]propenoate (E-2j). After the addition of petroleum ether (8 mL) to the evaporated residue E-2j was filtered off.
White solid; mp 88–90 °C (EtOH/H2O). IR (KBr) νmax/cm-1: 3566, 3331, 3248, 1723, 1655. 1H NMR (300 MHz): δ 1.89 (s, 3H, Me), 2.17 (s, 3H, Me), 3.47 (s, 3H, OMe), 6.53 (s, 1H, 3-H), 7.13 (m, 2H), 7.27 (m, 7H), 7.53 (m, 3H) in 7.92 (s, 2H) (two Ph, C6H4), 10.36 (s, 1H, NH). 13C NMR (75.5 MHz): δ 12.4, 16.9, 51.6, 112.9, 116.4, 126.4, 127.7, 128.17, 128.22, 128.3, 128.4, 128.8, 129.0, 129.3, 130.1, 130.6, 132.0, 132.9, 135.1, 138.7, 142.0, 146.8, 164.6, 165.1. MS–EI: m/z (%) = 451 (M+, 25), 105 (100). HRMS–EI: m/z (M+) calcd for C28H25N3O3 451.1896; found: 451.1905. Anal. Calcd for C28H25N3O3×¾H2O: C, 72.32; H, 5.74; N, 9.04. Found C, 72.18; H, 5.88; N, 9.01.
Methyl (E)-2-(benzoylamino)-3-[1-(4-chlorophenyl)-3-methyl-5-phenyl-1H-pyrazol-4-yl]propenoate (E-2k). After the addition of petroleum ether (8 mL) to the evaporated residue E-2k was filtered off.
White solid; mp 193–195 °C (EtOH). IR (KBr) νmax/cm-1: 3243, 1731, 1639. 1H NMR (300 MHz): δ 2.17 (s, 3H, Me), 3.50 (s, 3H, OMe), 6.43 (s, 1H, 3-H), 7.19 (m, 4H), 7.40 (m, 5H), 7.50 (m, 2H), 7.59 (m, 1H) in 7.89 (m, 2H) (two Ph, C6H4), 10.37 (s, 1H, NH). MS–EI: m/z (%) = 471 (M+, 46), 105 (100). Anal. Calcd for C27H22ClN3O3: C, 68.72; H, 4.70; N, 8.90. Found C, 68.86; H, 4.72; N, 8.82.
Methyl (E)-2-(benzoylamino)-3-{3-methyl-5-phenyl-1-[3-(trifluoromethyl)phenyl]-1H-pyrazol-4-yl}propenoate (E-2l). After the addition of Et2O (8 mL) to the evaporated residue E-2l was filtered off.
Yellowish solid; mp 211–213 °C (EtOH). IR (KBr) νmax/cm-1: 3262 br, 1726, 1640. 1H NMR (300 MHz): δ 2.20 (s, 3H, Me), 3.50 (s, 3H, OMe), 6.46 (s, 1H, 3-H), 7.22 (m, 2H), 7.39–7.67 (m, 10H) in 7.90 (m, 2H) (two Ph, C6H4), 10.39 (s, 1H, NH). MS–EI: m/z (%) = 505 (M+, 24), 105 (100). Anal. Calcd for C28H22F3N3O3: C, 66.53; H, 4.39; N, 8.31. Found C, 66.65; H, 4.41; N, 8.29.
Methyl (E)-2-(benzoylamino)-3-[1-(2-methoxycarbonylphenyl)-3-methyl-5-phenyl-1H-pyrazol-4-yl]propenoate (E-2n). The evaporated residue was purified on a chromatotron (petroleum ether–EtOAc, 5:3; then petroleum ether–EtOAc, 1:1) to give E-2n.
White solid; mp 177–180 °C (EtOH/H2O). IR (KBr) νmax/cm-1: 3190 br, 1726, 1672. 1H NMR (300 MHz): δ 2.15 (s, 3H, Me), 3.50 (s, 3H, OMe), 3.59 (s, 3H, OMe), 6.49 (s, 1H, 3-H), 7.11 (m, 2H), 7.29 (m, 4H), 7.48–7.69 (m, 5H), 7.72 (dd, 1H, J1 = 7.5 Hz, J2 = 1.5 Hz), 7.90 (m, 2H) (two Ph, C6H4), 10.34 (s, 1H, NH). 13C NMR (75.5 MHz): δ 12.4, 51.6, 52.1, 113.7, 116.3, 127.7, 128.3, 128.37, 128.40, 128.8, 129.0, 129.4, 129.8, 130.0, 131.9, 132.2, 132.9, 138.3, 141.8, 147.1, 164.6, 165.1, 165.8 (two signals are missing). MS–EI: m/z (%) = 495 (M+, 35), 374 (100), 105 (86). Anal. Calcd for C29H25N3O5: C, 70.29; H, 5.09; N, 8.48. Found C, 70.18; H, 5.08; N, 8.39.
Methyl (E)-2-(benzoylamino)-3-(1,5-dimethyl-3-phenyl-1H-pyrazol-4-yl)propenoate (E-2o). After the addition of petroleum ether (8 mL) to the evaporated residue E-2o was filtered off.
White solid; mp 158–162 °C (MeOH/H2O). IR (KBr) νmax/cm-1: 3269 br, 1732, 1645 cm-1. 1H NMR (300 MHz): δ 2.15 (s, 3H, 5′-Me), 3.45 (s, 3H, OMe), 3.79 (s, 3H, 1′-Me), 6.63 (s, 1H, 3-H), 7.26–7.44 (m, 3H, Ph), 7.48–7.64 (m, 5H, Ph), 7.95 (m, 2H, Ph), 10.38 (s, 1H, NH). 13C NMR (75.5 MHz): δ 10.0, 36.3, 51.6, 110.6, 118.0, 126.9, 127.2, 127.7, 128.36, 128.44, 129.0, 132.0, 132.9, 133.7, 138.2, 147.0, 164.6, 165.1. MS–EI: m/z (%) = 375 (M+, 25), 105 (100). Anal. Calcd for C22H21N3O3: C, 70.38; H, 5.64; N, 11.19. Found C, 70.64; H, 5.77; N, 10.91.
Methyl (E)-2-(benzoylamino)-3-(5-methyl-3-phenyl-1H-pyrazol-4-yl)propenoate (E-2p) and methyl (E)-2-(benzoylamino)-3-(1,3-dimethyl-5-phenyl-1H-pyrazol-4-yl)propenoate (E-2r). The evaporated residue was separated on a chromatotron (CH2Cl2–MeOH, 50:1; then CHCl3–MeOH, 40:1) to give E-2p (24%) and E-2r (35%).
Data for E-2p: White solid; mp 94–97 °C (MeOH/H2O). IR (KBr) νmax/cm-1: 3256 br, 1719, 1652, 1524 cm-1. 1H NMR (300 MHz): δ 2.10 and 2.15 (two s, 3H, Me), 3.42 and 3.47 (two s, 3H, OMe), 6.64 (s, 1H, 3-H), 7.27–7.67 (m, 8H), 7.94 (m, 2H) (two Ph), 10.37 (s, 1H, NH), 12.78 (br s) and 12.94 (br s) (1H, 1'-H of both tautomers). MS–EI: m/z (%) = 361 (M+, 16), 105 (100). Anal. Calcd for C21H19N3O3: C, 69.79; H, 5.30; N, 11.63. Found C, 69.63; H, 5.51; N, 11.52.
Data for E-2r: White solid; mp 70–73 °C (H2O). IR (KBr) νmax/cm-1: 3274 br, 1728, 1654 cm-1. 1H NMR (300 MHz): δ 2.07 (s, 3H, Me), 3.46 (s, 3H, OMe), 3.68 (s, 3H, 1′-Me), 6.37 (s, 1H, 3-H), 7.38–7.60 (m, 8H) and 7.89 (m, 2H) (two Ph), 10.27 (s, 1H, NH). 13C NMR (75.5 MHz): δ 12.3, 36.9, 51.6, 112.2, 117.1, 127.6, 128.4, 128.55, 128.57, 128.7, 129.4, 129.5, 131.9, 132.9, 141.4, 144.8, 164.7, 165.0. MS–EI: m/z (%) = 375 (M+, 48), 105 (100). Anal. Calcd for C22H21N3O3: C, 70.38; H, 5.64; N, 11.19. Found C, 70.23; H, 5.71; N, 11.09.
ACKNOWLEDGEMENTS
We thank the Ministry of Higher Education, Science and Technology of the Republic of Slovenia and the Slovenian Research Agency for financial support (P1-0230-0103). Dr B. Kralj and Dr D. Žigon (Center for Mass Spectroscopy, “Jožef Stefan” Institute, Ljubljana, Slovenia) are gratefully acknowledged for the mass measurements.
References
1. (a) R. M. Williams, 'Synthesis of Optically Active Amino Acids,' Pergamon Press, Oxford, 1989, Chapter 6; (b) P. Balaram, Curr. Opin. Struct. Biol., 1992, 2, 845; CrossRef (c) A. Giannis and T. Kolter, Angew. Chem., 1993, 105, 1303; CrossRef (d) J. Rizo, and L. M. Gierasch, Annu. Rev. Biochem., 1992, 61, 387; CrossRef (e) R. M. J. Liskamp, Recl. Trav. Chim. Pays-Bas, 1994, 113, 1; (f) R. S. McDowell and R. D. Artis, Annu. Rep. Med. Chem., 1995, 30, 265; CrossRef (g) S. Hanessian, G. McNaughton Smith, H. G. Lombart, and W. D. Lubell, Tetrahedron, 1997, 53, 6281; CrossRef (h) V. J. Hruby, G. Li, C. Haskell-Luevano, and M. Shenderovich, Biopolymers, 1997, 43, 219. CrossRef
2. (a) U. Schmidt, A. Lieberknecht, and J. Wild, Synthesis, 1988, 159; CrossRef (b) M. J. Smith, D. Kim, B. Horenstein, K. Nakanishi, and K. Kustin, Acc. Chem. Res., 1991, 24, 117; CrossRef (c) C. Bonauer, T. Walenzyk, and B. Koenig, Synthesis, 2006, 1; CrossRef (d) F. Požgan and M. Kočevar, Heterocycles, 2009, 77, 657; CrossRef (e) K. Kranjc and M. Kočevar, Curr. Org. Chem., 2010, 14, 1050. CrossRef
3. (a) L. M. Gross, P. M. Morell, and G. T. Craig, Proc. Natl. Acad. Sci. U.S.A., 1969, 62, 952; CrossRef (b) C. H. Stammer, 'Chemistry and Biochemistry of Amino Acids and Proteins,' Vol. 6, ed. by B. Weinstein, Marcel Dekker, New York, 1982, 33; (c) M. Aydin, N. Lucht, W. A. Koenig, R. Lupp, G. Jung, and G. Winkelmann, Liebigs Ann. Chem., 1985, 2285; CrossRef (d) G. D. Fate, C. P. Benner, S. H. Grode, and T. J. Gilbertson, J. Am. Chem. Soc., 1996, 118, 11363. CrossRef
4. (a) G. Jung, Angew. Chem., Int. Ed. Engl., 1991, 30, 1051; CrossRef (b) J. Petersen, A. Boysen, L. Fogh, K. Tabermann, T. Kofoed, A. King, P. Schrotz-King, and M. C. Hansen, Biol. Chem., 2009, 390, 437. CrossRef
5. (a) Y. Shimohigashi, C. H. Stammer, T. Costa, and P. F. von Voigtlander, Int. J. Pept. Protein Res., 1983, 22, 489; (b) S. F. Brady, C. W. Cochran, R. F. Nutt, F. W. Holly, C. D. Bennett, W. J. Paleveda, P. E. Curley, B. H. Arison, R. Saperstein, and D. Weber, Int. J. Pept. Protein Res., 1984, 23, 212; (c) S. Salvadori, M. Marastoni, G. Balboni, G. Marzola, and R. Tomatis, Int. J. Pept. Protein Res., 1986, 28, 254; (d) S. Salvadori, M. Marastoni, G. Balboni, G. Marzola, and R. Tomatis, Int. J. Pept. Protein Res., 1986, 28, 262; (e) S. Imazu, Y. Shimohigashi, H. Kodama, K. Sakaguchi, M. Waki, T. Kato, and N. Izumiya, Int. J. Pept. Protein Res., 1988, 32, 298.
6. (a) R. Noyori, 'Asymmetric Catalysis in Organic Syntheses,' Wiley-Interscience, New York, 1994, 16; (b) E. N. Jacobsen, A. Pfaltz, and H. Yamamoto, 'Comprehensive Asymmetric Catalysis,' Vol. 1, Springer-Verlag, Berlin and Heidelberg, 1999, 145.
7. (a) M. Kočevar, S. Polanc, M. Tišler, and B. Verček, Synth. Commun., 1989, 19, 1713; CrossRef (b) V. Kepe, M. Kočevar, S. Polanc, B. Verček, and M. Tišler, Tetrahedron, 1990, 46, 2081; CrossRef (c) M. Kočevar, S. Polanc, B. Verček, and M. Tišler, Liebigs Ann. Chem., 1990, 501; (d) V. Kepe, M. Kočevar, A. Petrič, S. Polanc, and B. Verček, Heterocycles, 1992, 33, 843; CrossRef (e) V. Kepe, M. Kočevar, and S. Polanc, Heterocycles, 1995, 41, 1299; CrossRef (f) V. Kepe, M. Kočevar, and S. Polanc, J. Heterocycl. Chem., 1996, 33, 1707; CrossRef (g) V. Kepe, S. Polanc, and M. Kočevar, Heterocycles, 1998, 48, 671; CrossRef (h) F. Požgan, K. Kranjc, V. Kepe, S. Polanc, and M. Kočevar, Arkivoc, 2007, viii, 97.
8. (a) P. Trebše, S. Polanc, M. Kočevar, T. Šolmajer, and S. Golič Grdadolnik, Tetrahedron, 1997, 53, 1383; CrossRef (b) L. Vraničar, S. Polanc, and M. Kočevar, Tetrahedron, 1999, 55, 271; CrossRef (c) L. Vraničar, A. Meden, S. Polanc, and M. Kočevar, J. Chem. Soc., Perkin Trans 1, 2002, 675; CrossRef (d) L. Vraničar, F. Požgan, S. Polanc, and M. Kočevar, Amino Acids, 2003, 24, 273; CrossRef (e) L. Vraničar, S. Polanc, and M. Kočevar, Heterocycles, 2003, 61, 105; CrossRef (f) J. Hren, F. Požgan, A. Bunič, V. I. Parvulescu, S. Polanc, and M. Kočevar, Tetrahedron, 2009, 65, 8216. CrossRef
9. F. Arndt, C. R. Noller, and I. Bergsteinsson, Org. Synth., Coll. Vol. II, 1948, 165.
10. R. Askani and D. F. Taber, 'Comprehensive Organic Synthesis,' Vol. 6, ed. by B. M. Trost and I. Fleming, Pergamon Press, Oxford, 1991, 103.
11. (a) S. Ghosh, D. B. Datta, and N. Sen, Synth. Commun., 1987, 17, 299; CrossRef (b) U. Nagai and V. Pavone, Heterocycles, 1989, 28, 589; CrossRef (c) G. Abbiati, F. Clerici, M. L. Gelmi, A. Gambini, and T. Pilati, J. Org. Chem., 2001, 66, 6299. CrossRef
12. (a) C. Cativiela, M. D. Díaz de Villegas, J. A. Mayoral, and E. Meléndez, J. Org. Chem., 1985, 50, 3167; CrossRef (b) T. Wakamiya, Y. Oda, H. Fujita, and T. Shiba, Tetrahedron Lett., 1986, 27, 2143. CrossRef
13. (a) J. Elguero, 'Comprehensive Heterocyclic Chemistry,' Vol. 5, ed. by A. R. Katritzky and C. W. Rees, Pergamon, Oxford, 1984, pp. 167−343; CrossRef (b) J. Elguero, 'Comprehensive Heterocyclic Chemistry II,' Vol. 3, ed. by A. R. Katritzky, C. W. Rees, and E. F. V. Scriven, Pergamon, Oxford, 1996, pp. 1−75; CrossRef (c) L. Yet, 'Comprehensive Heterocyclic Chemistry III,' Vol. 4, ed. by A. R. Katritzky, C. A. Ramsden, E. F. V. Scriven, and R. J. K. Taylor, Elsevier, Amsterdam, 2008, pp.1−141; CrossRef (d) M. Begtrup, G. Boyer, P. Cabildo, C. Cativiela, R. M. Claramunt, J. Elguero, J. I. García, C. Toiron, and P. Vedsø, Magn. Reson. Chem., 1993, 31, 107. CrossRef