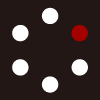
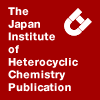
HETEROCYCLES
An International Journal for Reviews and Communications in Heterocyclic ChemistryWeb Edition ISSN: 1881-0942
Published online by The Japan Institute of Heterocyclic Chemistry
e-Journal
Full Text HTML
Received, 12th May, 2010, Accepted, 14th June, 2010, Published online, 16th June, 2010.
DOI: 10.3987/COM-10-S(E)28
■ Dehydrogenation of 5,6-Dihydropyrido[3,2-d]pyrimidin-7(8H)-ones: A Convenient Last Step for a Synthesis of Pyrido[2,3-d]pyrimidin-7(8H)-ones
Irene Pérez-Pi, Xavier Berzosa, Iñaki Galve, Jordi Teixidó , and José I. Borrell*
Molecular Engineering Group, IQS, Ramon Llull University, Via Augusta 390, E-08017 Barcelona, Spain
Abstract
Two new protocols for the dehydrogenation of 5,6-dihydropyrido[2,3-d]pyrimidin-7(8H)-ones to pyrido[2,3-d]pyrimidin-7(8H)- -ones are described. The first one uses NaH in DMSO an affords the corresponding pyridopyrimidines when an aryl substituent is present at C6. The second one is of a more general use, allowing dehydrogenation of aryl and alkyl substituted 5,6-dihydropyridopyrimdines upon treatment with Na2SeO3 in DMSO.INTRODUCTION
Among the different kinds of structures currently being tested as potential protein kinase inhibitors, pyrido[2,3-d]pyrimidin-7(8H)-ones have arisen as very promising candidates. Thus, compounds of general structure 1 have shown IC50 in the range µM to nM in front of PDFGR, FGFR, EGFR and c-Scr.1 Usually this kind of compounds are obtained through a multistep strategy in which the pyridine ring is constructed by condensation of a nitrile 2 (bearing the desired substituent R1) onto preformed pyrimidine aldehyde 3 bearing substituent R5 and a methylthio group which can be later substituted by the NHR4 substituent using an amine 4 (Scheme 1).
In this context, our group has a broad experience in the synthesis of 5,6-dihydropyrido[2,3-d]pyrimidin-7(8H)-ones (10; R3 = NH2) and (11; R3 = OH) from α,β-unsaturated esters (5) (Scheme 2). Thus, in the so called cyclic strategy 2-methoxy-6-oxo-1,4,5,6- tetrahydropyridin-3-carbonitriles (7) are obtained by reaction of an α,β-unsaturated ester (5) and malononitrile (6, G = CN) in NaOMe/MeOH.2 Treatment of pyridones 7 with guanidine systems (9, R4 = H, alkyl, aryl, heteroaryl) affords 4-amino-pyrido[2,3-d]pyrimidines (10, R3=NH2).3 On the other hand, we described an acyclic variation of the above protocol for the synthesis of pyridopyrimidines (10, R3 = NH2) based on the isolation of the corresponding Michael adduct (8, G = CN) and later cyclization with a guanidine 9.4 This approach also allowed us to obtain 4-oxopyrido[2,3-d]pyrimidines (here depicted as the hydroxyl tautomer 11, R3 = OH) by treatment of intermediates (8, G = CO2Me), synthesized by Michael addition of α,β-unsaturated esters (5) and methyl cyanoacetate (6, G = CO2Me), with guanidines 9.5 We have also described a multicomponent microwave-assisted cyclocondensation affording systems 10 and 11 via acyclic intermediates 8.6 More recently, we have achieved the synthesis of 4-unsubstituted 5,6-dihydropyrido[2,3-d]pyrimidines (12; R3 = H) through an unusual Michael addition between 2-aryl substituted acrylates (5; R1 = aryl, R2 = H) and 3,3-dimethoxypropanenitrile (13) which leads, depending on the reaction temperature (60 ºC or -78 ºC, respectively), to a 4-methoxymethylene substituted 4-cyanobutyric ester (15) or to a 4-dimethoxymethyl 4-cyanobutyric ester (14). These compounds can be subsequently converted to the desired 4-unsubstituted 5,6-dihydropyrido[2,3-d]pyrimidines (12; R3 = H) upon treatment with a guanidine carbonate 9 under microwave irradiation.7
The main difference between systems 10, 11 and, particularly, 12 and the biologically active pyrido[2,3-d]pyrimidin-7(8H)-ones (1) is the absence of a double bond between C5 and C6. Consequently, a facile dehydrogenation protocol for the conversion of 5,6-dihydropyrido[2,3-d]pyrimidin-7(8H)-ones to pyrido[2,3-d]pyrimidin-7(8H)-ones will complete a strightforward synthesis of these later ones. However, in the literature there are only two examples of such dehydrogenation: the use of MnO2 or Br2 in AcOH,8 and a treatment with Pd in AcOH.9 The present paper deals with a re-examination of such dehydrogenation protocols.
RESULTS AND DISCUSSION
In 1989, our group described8 the use of both MnO2 (freshly prepared by the Attenburrow method10) in AcOH and the treatment with Br2 in AcOH to transform 5,6-dihydropyridopyrimidines 10 (R3 = NH2) into the aromatic ones 16 (Scheme 3). The results obtained mainly depended on the nature of substituents R1 and R2. Thus, in the case of MnO2, the dehydrogenation to 16 (R1 = Me, R2 = R4 = H) took place in 95% yield while 16 (R1 = H, R2 = Me, R4 = H) gave a 75% yield. On the other hand, the treatment of 10 (R1 = Me, R2 = R4 = H) with Br2 in AcOH afforded 16 (R1 = Me, R2 = R4 = H) in 90% yield. However, when 10 (R1 = H, R2 = Me, R4 = H) was treated in the same reaction conditions, the dehydrogenation product 17 (R1 = H, R2 = Me, R4 = H) included a bromine atom at C6 (30% yield).
Although the results were good for 2,4-diamino substituted 5,6-dihydropyridopyrimidines 10, the fact that the MnO2 should be freshly prepared (nowadays activated MnO2 is commercially available from Aldrich ref. nº 217646, but yields were lower) and our idea of extending the dehydrogenation protocol to a wide range of substituents, prompted us to test new dehydrogenating agents.
The first one arose in front of us as an example of serendipity. As a part of the development of the synthetic protocol to obtain 4-unsubstituted 5,6-dihydropyrido[2,3-d]pyrimidines (12; R3 = H),7 we were interested in the debromination of compound 18. When we tested NaH/DMSO for such purpose, the debromination took place but also the dehydrogenation at C5-C6 affording compound 19 in 80% yield.
Such result led us to use NaH/DMSO as dehydrogenating agent, so we tested it on 5 model compounds: 10{2,1}, 10{3,1}, 10{4,1}, 10{2,2}, and 12{1,1}; selected to cover a wide range of substituent type, substitution position and nature of R3 (NH2 and H) and R4 (H and Ph). These compounds were synthesized according to Scheme 2 from the corresponding α,β-unsaturated esters 5{1-4}, malononitrile (6, G = CN) (for compounds 10) or 3,3-dimethoxypropanenitrile (13) (for compound 12{1,1}), and guanidine 9{1} or phenylguanidine 9{2}. The results of the dehydrogenation with NaH/DMSO are summarized in Scheme 5.
As it can be seen the reaction only proceeded when the substituent is present in the pyridone ring at position C6 although the yield obtained strongly depends on the nature of R1, being higher when it is a phenyl ring. This result is in agreement with our interpretation of the possible mechanism by which this oxidation can take place. The calculated pKa values (SPARC v. 4.5, http://ibmlc2.chem.uga.edu/sparc/)11 of the α- and β-carbonyl hydrogens, the lactam NH, and the NHR4 and R3 substituents are summarized on Table 1.
It is easy to see that those compounds giving the best results in this dehydrogenation (12{1,1} and 18) present an α-carbonyl hydrogen more acidic than the lactam NH, while the situation is the contrary in those compounds which have not reacted (10{3,1} and 10{4,1}). When the pKa values are similar (10{2,1} and 10{2,2}) the reaction proceed but the yield is very low. Although there are some examples of direct dehydrogenation of an heterocyclic ring by heating in DMSO,12 we postulate for this case a mechanism in which an initial loss of proton from α-carbonyl (C6) is followed by a hydride transfer from C5 to the DMSO. The possible role of air oxygen as coadjuvant in this oxidation can not be denied because when the reaction was carried out in an inert atmosphere (Ar) the conversions obtained were much lower. Such dehydrogenation is favoured by the presence of aryl substituents at C6, precisely the kind of substituents present in biologically active compounds 1.1
The second methodology tested for the dehydrogenation of 5,6-dihydropyrido[2,3-d]pyrimidin-7(8H)- ones was based on a recent paper by Lamba and Makrandi13 in which they describe the use of sodium selenite (Na2SeO3) in DMSO as a highly efficient reagent for dehydrogenation. Thus, the treatment of flavonones (22) with Na2SeO3 in DMSO affords flavones (23), both with conventional heating or under microwave irradiation, in high yields (60-85%) (Scheme 6).
Consequently, we tested such oxidizing agent on the same model compounds previously used in the dehydrogenation with NaH/DMSO. The results are summarized in Scheme 7.
The dehydrogenation was tested by varying the following parameters: (i) ratio between the corresponding 5,6-dihydropyridopyrimidine and Na2SeO3 (1:1, 1:1.2, and 1:1.5), (ii) volume of DMSO (2, 3, and 4 mL), (iii) reaction temperature (110 ºC, 140 ºC, and 160 ºC), (iv) conventional heating or microwave irradiation, and (v) air or Ar atmosphere. The best reaction conditions were 1.2 equivalents of Na2SeO3, 2 mL of DMSO (in order to favour precipitation of the final product by addition of water), conventional heating at 160 ºC for 6 h, and air atmosphere protected from moisture or Ar atmosphere depending on the nature of the substituents (alkyl substituents proceeded better with air atmosphere). Using this protocol, all the 5,6-dihydropyridopyrimidines tested (unless 10{3,1}, R1 = H, R2 = Me, R3 = NH2, R4 = H) afforded the corresponding pyrido[2,3-d]pyrimidin-7(8H)-one in high yield (90-100%). In the case of 10{2,2} (R1 = Me, R2 = H, R3 = NH2, R4 = Ph), it was necessary to increse the ratio 10{2,2}: Na2SeO3 to 1:6 in order to drive the reaction to completeness.
Although in the paper by Lamba and Makrandi13 there is not a mechanistic proposal for the dehydrogenation with Na2SeO3, it is possible to assume a mechanism similar to that proposed by Corey and Schaefer14 for the dehydrogenation of 1,4-diketones with SeO2. Thus, the formation of the pyrido[2,3-d]pyrimidin-7(8H)-one could proceed via an intermediate enol selenite ester followed by a concerted 1,4-elimination from the enol ester. However, other reaction paths can not be excluded.
In summary, we have developed two new protocols for the conversion of 5,6-dihydropyrido[2,3-d]pyrimidin-7(8H)-ones into pyrido[2,3-d]pyrimidin-7(8H)-ones by dehydrogenation. The use of NaH/DMSO is particularly interesting for those cases in which an aryl group is present at C6 (the kind of substituents present in biologically active compounds 1). On the other hand, sodium selenite is of a more general use, providing good yields unless for alkyl substituents at C5.
These protocols complete a very convenient synthesis of pyrido[2,3-d]pyrimidin-7(8H)-ones in which the pyridopyrimdine skeleton is formed in one or two steps from an α,β-unsaturated ester (5), an active methylene compound (malononitrile (6, G = CN), methyl cyanoacetate (6, G = CO2Me) or 3,3-dimethoxypropanenitrile (13)) and a guanidine (9) (Scheme 2), and subsequently converted to a pyrido[2,3-d]pyrimidin-7(8H)-one by dehydrogenation with one of the aforementioned protocols.
EXPERIMENTAL
All melting points were determined with a Büchi 530 capillary apparatus and were uncorrected. Infrared spectra were recorded in a Nicolet Magna 560 FTIR spectrophotometer. 1H and 13C NMR spectra were determined in a Varian-400MR operating in field strength of 400 and 100.5 MHz, respectively. Chemical shifts were reported in parts per million (δ) and Coupling constants (J) in Hz, using in the case of 1H NMR, tetramethylsilane (TMS) or sodium 2,2,3,3-tetradeuteriotrimethyl-silylpropionate (TSPNa) as internal standards and setting, in the case of 13C NMR, the reference at the signal of the solvent (39.5 ppm, DMSO-d6 and 163.8 ppm, CF3CO2D, TFA-d). Standard and peak multiplicities were designated as follows: s, singlet; d, doublet; dd, doublet of doublets; t, triplet; br, broad signal; m, multiplet. MS (EI, 70 EV) were registered on na Agilent Technologies 5975. HRMS were registered at the Unidade de Espectrometria de Masas (Universidade de Santiago de Compostela) using a Micromass Autospec spectrometer. All microwave irradiation experiments were carried out in a dedicated Biotage-Initiator microwave apparatus, operating at a frequency of 2.45 GHz with continuous irradiation power from 0 to 400 W with utilization of the standard absorbance level of 400 W maximum power. Reactions were carried out in 10-mL glass tubes, sealed with aluminium/Teflon crimp tops, which can be exposed up to 250 °C and 20 bar internal pressure. Temperature was measured with an IR sensor on the outer surface of the process vial. After the irradiation period, the reaction vessel was cooled rapidly (60–120 s) to ambient temperature by air jet cooling.
The reagents and solvents were purchased from Sigma-Aldrich and were used directly. Compounds 10{2,1}, 10{3,1}, 10{4,1}, and 12{1,1} were prepared according to reported procedures.2b,6,7
2,4-Diamino-6-methylpyrido[2,3-d]pyrimidin-7(8H)-one (20{2,1}):
Method a) Using NaH in DMSO: A mixture of 0.193 g (1 mmol) of 2,4-diamino-5,6-dihydro-6- methylpyrido[2,3-d]pyrimidin-7(8H)-one (10{2,1})2b,6 and 0.120 g (3 mmol) of NaH (60% dispersion in mineral oil) in 10 mL of DMSO was heated for 15 h at 120 ºC in an air atm. The resulting solution was cooled down, water (200 mL) was added and it was neutralized with AcOH. The resulting precipitate was filtered, washed with EtOH and Et2O, and dried to afford 57.4 mg (0.30 mmol, 30%) of 20{2,1}, mp >250 ºC (lit.,8 >350 ºC). IR (KBr): ν (cm-1) 3334-3163 (NH2), 2919, 2784, 1626 (C=O), 1546 (C=N), 1465, 793. 1H NMR (400 MHz, TFA-d): 8.15 (s, 1H, H-C5), 2.25 (s, 3H, Me). 13C NMR (100.5 MHz, DMSO-d6): δ (ppm) 165.2 (C8a), 162.8 (C2), 161.4 (C7), 155.7 (C4), 133.0 (C5), 120.4 (C6), 90.8 (C4a), 16.3 (Me). MS (EI): m/z: 190.09 [M]+. HRMS (EI): Calc. for C8H9N5O: 191.0807. Found: 191.0808.
Method b) Using Na2SeO3 in DMSO: A mixture of 0.193 g (1 mmol) of 10{2,1} and 0.207 g of Na2SeO3 (1.2 mmol) in 2 mL of DMSO was heated at 160 ºC for 6h protected from moisture. Upon cooling, 200 mL of water were added and the resulting precipitate was filtered, washed with EtOH and Et2O, and dried to afford 168.2 mg (0.88 mmol, 88%) of 20{2,1}.
2,4-Diamino-5-phenylpyrido[2,3-d]pyrimidin-7(8H)-one (20{4,1}):
Method b) Using Na2SeO3 in DMSO: A mixture of 0.255 g (1 mmol) of 2,4-diamino-5,6-dihydro-5- phenylpyrido[2,3-d]pyrimidin-7(8H)-one (10{4,1})2b,6 and 0.207 g of Na2SeO3 (1.2 mmol) in 2 mL of DMSO was heated at 160 ºC for 6 h in an Ar atm. Upon cooling, 200 mL of water were added and the resulting precipitate was filtered, washed with EtOH and Et2O, and dried to afford 248.2 mg (0.98 mmol, 98%) of 20{4,1}, mp >250 ºC. IR (KBr): ν (cm-1) 3477 (NH), 3309-3178 (NH2), 1636 (C=O), 1611 (C=N), 1611,1570,1525 (C=N), 1472, 1385, 701. 1H-NMR (400 MHz, DMSO-d6): δ (ppm) 11.94 (s, 1H, H-N8), 7.47 (m, 5H, H-Ph), 6.78 (br, 2H, NH2), 5.70 (s, 1H, H-C6). 13C-NMR (100.5 MHz, DMSO-d6): δ (ppm) 163.0 (C8a), 162.6 (C7), 161.3 (C2), 157.8 (C4), 151.1 (C5), 139.0 (Ph), 129.5 (Ph), 129.5 (Ph), 128.0 (Ph), 114.9 (C6), 90.0 (C4a). MS (EI): m/z: 253.1 [M]+(100), 252.10 [M-1]+ (88). HRMS (EI): Calc. for C13H11N5O: 253.0964. Found: 253.0964.
4-Amino-6-methyl-2-(phenylamino)pyrido[2,3-d]pyrimidin-7(8H)-one (20{2,2}):
A mixture of 536 mg (3 mmol) of phenyl guandine carbonate and 324 mg (6 mmol) of NaOMe in 5 mL of MeOH are heated in a sealed microwave vial with stirring under microwave irradiation for 15 min at 65 ºC. The resulting mixture is transferred by filtration to a second microwave vial and 0.1 mL (1 mmol) of methyl methacrylate and 79 mg (1.2 mmol) of malononitrile were added. The mixture was heated under microwave irradiation for 10 min at 140 ºC. The resulting precipitate was filtered off, washed with water, cold EtOH and Et2O to give 27.5 mg (0.11 mmol, 11%) of 4-amino-5,6-dihydro-6-methyl-2- (phenylamino)pyrido[2,3-d]pyrimidin-7(8H)-one 10{2,2}, mp >250 ºC (lit.,15 367 ºC). 1H-NMR (400 MHz, DMSO-d6): δ (ppm) 10.03 (s, 1H, H-N8), 8.69 (s, 1H, NHPh), 7.82 (d, J = 7.6 Hz, 2H, o-PhH), 7.18 (m, 2H, m-PhH), 6.83 (t, J = 7.3 Hz, 1H, p-PhH), 6.34 (s, 2H, NH2), 2.79 (dd, J = 15.6 Hz, J = 6.8 Hz, 1H, H-C6), 2.56 (m, 1H, H-C5), 2.24 (dd, J = 15.6 Hz, J = 11.3 Hz, 1H, H-C5). 13C NMR (100.5 MHz, TFA-d): δ (ppm) 179.2 (C8), 156.6 (C2), 155.3 (C4), 152.8 (C8a), 133.4 (Ph), 132.5 (Ph), 131.9 (Ph), 127.4 (Ph), 88.0 (C4a), 36.2 (C5), 25.4 (C6), 15.7 (Me).
Method a) Using NaH in DMSO: A mixture of 0.269 g (1 mmol) of 10{2,2} and 0.120 g (3 mmol) of NaH (60% dispersion in mineral oil) in 10 mL of DMSO was heated for 15 h at 120 ºC in an air atm. The resulting solution was cooled down, water (200 mL) was added and it was neutralized with AcOH. The resulting precipitate was filtered, washed with EtOH and Et2O, and dried to afford 80.1 mg (0.30 mmol, 30%) of 20{2,2}.
Method b) Using Na2SeO3 in DMSO: A mixture of 0.269 g (1 mmol) of 10{2,2} and 1.038 g of Na2SeO3 (6 mmol) in 4 mL of DMSO was heated at 160 ºC for 6 h protected from moisture. Upon cooling, 200 mL of water were added and the resulting precipitate was filtered, washed with EtOH and Et2O, and dried to afford 247.3 mg (0.90 mmol, 90%) of 20{2,2}, mp >250 ºC. IR (KBr): ν (cm-1) 3461 (NH), 3314 (NH2), 3171, 3074, 2961, 1643 (C=O), 1592, 1570, 1532 (C=N), 1500, 1439, 1292, 905, 794. 1H-NMR (400 MHz, DMSO-d6): δ (ppm) 11.75 (s, 1H, H-N8), 9.23 (s, 1H, NHPh), 7.90 (s, 1H, C5), 7.89 (d, J = 4Hz, 2H, H-Ph), 7.25 (br, 2H, NH2), 7.22 (d, J = 4Hz, 2H, H-Ph), 6.91 (t, J = 7.2 Hz, 1H, H-Ph), 2.00 (s, 3H, Me). 13C NMR (100.5 MHz, DMSO-d6): δ (ppm) 164.6 (C8a), 160.9 (C2), 159.1 (C7), 155.3 (C4), 141.3 (C5), 132.1 (C6), 128.7 (Ph), 122.4 (Ph), 121.5 (Ph), 119.6 (Ph), 91.65 (C4a), 16.64 (Me). MS (EI): m/z: 267.1 [M]+(75), 266.1 [M-H]+ (100). HRMS (EI): Calc. for C14H13N5O: 267.1120. Found: 267.1120.
2-Amino-6-(2,6-dichlorophenyl)pyrido[2,3-d]pyrimidin-7(8H)-one (21{1,1}):
Method a) Using NaH in DMSO: A mixture of 0.150 g (0.48 mmol) of 2-amino-6-(2,6-dichlorophenyl)- 5,6-dihydropyrido[2,3-d]pyrimidin-7(8H)-one (12{1,1})7 and 0.058 g (1.44 mmol) of NaH (60% dispersion in mineral oil) in 10 mL of DMSO was heated for 2 h at 100 ºC in an air atm. The resulting solution was cooled down, water (200 mL) was added and it was neutralized with AcOH. The resulting precipitate was filtered, washed with EtOH and Et2O, and dried to afford 0.12 g (0.39 mmol, 81%) of 21{1,1} as a yellowish solid, mp >250 ºC (lit.,1b 410 ºC). IR (KBr): ν (cm-1) 3595 (NH), 3314-3174 (NH2), 1624 (C=O), 1548 (N=C), 1512 (N=C), 1429, 1400, 784. 1H NMR (400 MHz, DMSO-d6): δ (ppm) 11.94 (s, 1H, H-N8), 8.61 (s, 1H, H-C4), 7.71 (s, 1H, H-C5), 7.53 (d, J = 7.81 Hz , 2H, H-Ph), 7.41 (m, J = 7.44 Hz, J = 8.73 Hz, 1H, H-Ph), 7.24 (s, 2H, NH2). 13C NMR (100.5 MHz, DMSO-d6): δ (ppm) 163.6 (C8a), 161.8 (C2), 159.7 (C7), 156.4 (C4), 138.6 (C6), 135.5 (Ph), 134.7 (C5), 130.9 (Ph), 128.5 (Ph), 124.3 (Ph), 104.5 (C4a). MS (EI): m/z: 306.0 (2) [M]+, 271.0 (100) [M-Cl]+. HRMS (EI): Calc. for C13H8Cl2N4O: 306.0075. Found: 306.0071.
Method b) Using Na2SeO3 in DMSO: A mixture of 0.309 g (1 mmol) of 12{1,1} and 0.207 g of Na2SeO3 (1.2 mmol) in 2 mL of DMSO was heated for 6 h at 160 ºC in Ar atm. The resulting solution was cooled down and water (200 mL) was added. The resulting precipitate was filtered, washed with EtOH and Et2O, and dried to afford 0.30 g (0.98 mmol, 98%) of 21{1,1}.
2-Amino-6-phenylpyrido[2,3-d]pyrimidin-7(8H)-one (19):
Using NaH in DMSO: A mixture of 0.100 g (0.31 mmol) of 2-amino-6-(2-bromophenyl)-5,6-dihydro- pyrido[2,3-d]pyrimidin-7(8H)-one (18)7 and 40 mg (1.00 mmol) of NaH (60% dispersion in mineral oil) in 10 mL of DMSO was heated for 15 h at 80 ºC in an Ar atm. The resulting solution was cooled down, water (200 mL) was added and it was neutralized with AcOH. The resulting precipitate was filtered, washed with EtOH and Et2O, and dried to afford 60 mg (0.25 mmol, 80%) of 19, mp >250 ºC (lit.,16 390 ºC). IR (KBr): ν (cm-1) 3595 (NH), 3306-3177 (NH2), 1641 (C=O), 1548 (N=C), 1513 (N=C), 1402, 1270, 1225. 1H NMR (400 MHz, DMSO-d6): δ (ppm) 12.07 (s, 1H, H-N8), 8.58 (s, 1H, H-C4), 7.91 (s, 1H, H-C5), 7.67 (d, 2H, H-Ph), 7.42 (m, 1H, H-Ph), 7.35 (m, 1H, H-Ph), 7.15 (s, 2H, NH2). 13C NMR (100.5 MHz, DMSO-d6): δ (ppm) 162.9 (C8a), 162.7 (C2), 159.0 (C7), 155.3 (C4), 136.1 (C6), 135.4 (C5), 128.4 (Ph), 127.9 (Ph), 127.3 (Ph), 126.6 (Ph), 104.8 (C4a). MS (EI): m/z: 306.0 (2) [M]+, 271.0 (100) [M-Cl]+. HRMS (EI): Calc. for C13H11N4O: 239.0933 [M++1]. Found: 239.0929.
ACKNOWLEDGEMENTS
X. Berzosa is grateful for a fellowship from Generalitat de Catalunya (2006-2009, grant 2006FI 00058). I. Galve wants to thank IQS for a PhD grant.
References
1. a) J. M. Hamby, C. J. C. Connolly, M. C. Schroeder, R. T. Winters; H. D. H. Showalter, R. L. Panek, T. C. Major, B. Olsewski, M. J. Ryan, T. Dahring, G. H. Lu, J. Keiser, A. Amar, C. Shen, A. J. Kraker, V. Slintak, J. M. Nelson, D. W. Fry, L. Bradford, H. Hallak, and A. M. Doherty, J. Med. Chem., 1997, 40, 2296; CrossRef b) S. R.Klutchko, J. M. Hamby, D. H. Boschelli, Z. Wu, A. J. Kraker, A. M. Amar, B. G. Hartl, C. Shen, W. D. Klohs, R. W. Steinkampf, D. L. Driscoll, J. M. Nelson, W. L. Elliott, B. J. Roberts, C. L. Stoner, P. W. Vincent, D. J. Dykes, R. L. Panek, G. H. Lu, T. C. Major, T. K. Dahring, H. Hallak, L. A. Bradford, H. D. H. Showalter, and A. M. Doherty, J. Med. Chem., 1998, 41, 3276; CrossRef c) D. H. Boschelli, Z. Wu, S. R. Klutchko, H. D. H. Showalter, J. M. Hamby, G. H. Lu, T. C. Major, T. K. Dahring, B. Batley, R. L. Panek, J. Keiser, B. G. Hartl, A. J. Kraker, W. D. Klohs, B. J. Roberts, S. Patmore, W. L. Elliott, R. Steinkampf, L. A. Bradford, H. Hallak, and A. M. Doherty, J. Med. Chem., 1998, 41, 4365; CrossRef d) J. F. Dorsey, R. Jove, A. J. Kraker, and J. Wu, Cancer Res., 2000, 60, 3127; e) D. Wisniewski, C. L. Lambek, C. Liu, A. Strife, D. R. Veach, B. Nagar, M. A. Young, T. Schindler, W. G. Bornmann, J. R. Bertino, J. Kuriyan, and B. Clarkson, Cancer Res., 2002, 62, 4244; f) M. Huang, J. F. Dorsey; P. K. Epling-Burnette, R. Nimmanapalli, T. H. Landowski, L. B. Mora, G. Niu, D. Sinibaldi, F. Bai, A. Kraker, H. Yu, L. Moscinski, S. Wei, J. Djeu, W. S. Dalton, K. Bhalla, T. P. Loughran, J. Wu, and R. Jove, Oncogene 2002, 21, 8804; CrossRef g) D. R. Huron, M. E. Gorre, A. J. Kraker, C. L. Sawyers, N. Rosen, and M. M. Moasser, Clin. Cancer Res., 2003, 9, 1267; h) N. C. Wolff, D. R. Veach, W. P. Tong, W. G. Bornmann, B. Clarkson, and R. L. Ilaria Jr., Blood, 2005, 105, 3995. CrossRef
2. (a) P. Victory and J.Diago, Afinidad, 1978, 35, 154; (b) P. Victory and J. Diago, Afinidad, 1978, 35, 161; (c) P. Victory, J. M. Jover, and J. Sempere, Afinidad 1981, 38, 49; (d) P. Victory and J. I. Borrell, ‘Trends in Heterocyclic Chemistry: 6-Alkoxy-5-cyano-3,4-dihydro-2-pyridones as starting materials for the synthesis of heterocycles’, Vol. 3, ed. by J. Menon, Council of Scientific Research Integration, Trivandrum, India, 1993, pp 235-247 and references therein.
3. (a) P. Victory, J. M. Jover, and R. Nomen, Afinidad, 1981, 38, 497; (b) P. Victory, R. Nomen, O. Colomina, M. Garriga, and A. Crespo, Heterocycles, 1985, 23, 1135. CrossRef
4. J. I. Borrell, J. Teixidó, J. L. Matallana, B. Martínez-Teipel, C. Colominas, M. Costa, M. Balcells, E. Schuler, and M. J. Castillo, J. Med. Chem., 2001, 44, 2366. CrossRef
5. J. I. Borrell, J. Teixidó, B. Martínez-Teipel, B. Serra, J. L. Matallana, M. Costa, and X. Batllori, Collect. Czech. Chem. Commun., 1996, 61, 901. CrossRef
6. (a) N. Mont, J. Teixidó, J. I. Borrell, and C. O. Kappe, Tetrahedron Lett., 2003, 44, 5385; CrossRef (b) N. Mont, J. Teixidó, C. O. Kappe, and J. I. Borrell, Mol. Diversity, 2003, 7, 153. CrossRef
7. (a) X. Berzosa, X. Bellatriu, J. Teixidó, and J. I. Borrell, J. Org. Chem., 2010, 75, 487; CrossRef (b) X. Berzosa and J. I. Borrell, ‘Síntesis y usos de 4-cianopentanoatos y 4-cianopentenoatos sustituidos’, ES200901191, April 29, 2009.
8. P. Victory, A. Crespo, R. Nomen, and J. I. Borrell, Afinidad, 1989, 46, 107.
9. G. Jin, Ch. C. N. Wu, D. A. Carson, and H. B. Cottam, Nucleos. Nucleot. Nucl., 2006, 25, 1391. CrossRef
10. J. Attenburrow, A. F. B. Cameron, J. H. Chapman, R. M. Evans, B. A. Hems, A. B. A. Jansen, and T. Walker, J. Chem. Soc., 1952, 1094. CrossRef
11. S. H. Hilal, S. W. Karickhoff, and L. A. Carreira, Quant. Struc. Act. Rel., 1995, 14, 348. CrossRef
12. M. Anastassiadou, G. Baziard-Mouysset, and M. Payard, Synthesis, 2000, 1814. CrossRef
13. M. Lamba and J. K. Makrandi, J. Chem. Research., 2008, 225.
14. E. J. Corey and J. P. Schaefer, J. Am. Chem. Soc., 1960, 82, 917. CrossRef
15. P. Victory, A. Crespo, M. Garriga, and R. Nomen, J. Heterocycl. Chem., 1988, 25, 245. CrossRef
16. C. J. Blankley, A. M. Doherty, J. M. Hamby, R. L. Panek, M. C. Schroeder, H. D. H. Showalter, and C. Connolly, US Patent 5733913 A, 19980331.