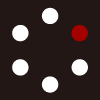
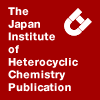
HETEROCYCLES
An International Journal for Reviews and Communications in Heterocyclic ChemistryWeb Edition ISSN: 1881-0942
Published online by The Japan Institute of Heterocyclic Chemistry
e-Journal
Full Text HTML
Received, 20th April, 2010, Accepted, 21st June, 2010, Published online, 22nd June, 2010.
DOI: 10.3987/COM-10-S(E)21
■ Relative Stabilities of 1- and 2-Substituted 1,2,3-Triazoles
Alan R. Katritzky,* Ana-Maria Buciumas, Ekaterina Todadze, Munawar Ali Munawar, and Levan Khelashvili
Center for Heterocyclic Compounds, Department of Chemistry, University of Florida, Gainesville, FL 32611-7200, U.S.A.
Abstract
N-(α-Aminoalkyl)-1,2,3-triazoles are potentially tautomeric between the 1- and 2-substituted forms. They exist in solution predominantly as 2-isomers as shown by 1H and 13C NMR; electron attracting substituents on the amino nitrogen increase the proportion of the 1-isomer in the equilibrium mixture.INTRODUCTION
The relative stabilities of 1- 1A and 2-substituted benzotriazoles 1B have been studied intensively.1-7 When the N-substituent is hydrogen (X = H in 1A, 1B) there is rapid tautomeric exchange between 1A and 1B (Figure 1),8 and this equilibrium is overwhelmingly on the side of the 1-H benzotriazole 1A in the crystalline state, but significant amounts of 2-H benzotriazole 1B are found in gas and solution phases.2,9 Rapidly equilibrating N-(dialkylaminomethyl)benzotriazoles are shown by 1H, 13C NMR and X-ray analysis to exist solely in the 1-substituted form in the crystalline phase but as mixtures of benzotriazole-1-yl and 2-yl isomers in solution and vapor phases.2 These results are explained by (i) the greater aromaticity of 1-substituted benzotriazoles and (ii) the fact that in media of low dielectric constant the much higher dipole moment of a 1-substituted benzotriazole compared to its 2-substituted isomer displaces the equilibrium towards the 2-substituted form (e.g. 1-methylbenzotriazole: 4.65D, 2-methylbenzotriazole: 0.77D).10
Far less is known about the corresponding 1- and 2-substituted monocyclic 1,2,3-triazoles (Figure 2). When the N-substituent is simply a hydrogen atom, 2H-tautomer 2B is calculated to be about 4.5 kcal mol-1 more stable than 1H tautomer 2B in the gas phase and this is supported by experiment.3,11-12 In solution, the more polar 1H isomer with the higher dipole moment (4.38 D) exists alongside the 2H tautomer.3,11 Low temperature 1H NMR studies of the parent 1,2,3-triazole by Lunazzi et al. indicated tautomeric equilibrium in CD2Cl2 and toluene: at -98 oC in CD2Cl2 the 1H form 2A is predominant, while at 27 °C the 2H form 2B is observed to the extent of 80% in CD2Cl2 and 97% in toluene.13 Similar results were obtained by Begtrup.14 Based on circumstantial arguments (basicity and partitioning) Albert and Taylor concluded that in aqueous solution the 2H form of 1,2,3-triazole is favored over the 1H tautomer by a factor of about two.15
Quantum chemical calculations indicate that the 2H form of 4-Me-1,2,3-triazole is more stable than the 1-isomer even in aqueous solution, despite the smaller dipole moment of this former.11,16 Ab initio calculations for C-substituted 1,2,3-triazoles suggest that 2H tautomers predominate in the gas phase, while both 1H and 2H tautomers are present in aqueous solution.17
The two tautomers of 1,2,3-triazole allow three different complexes connected by two hydrogen bonds, i,e, 1:1, 1:2 and 2:2 (Figure 3). According to CCSD(T)/[aug]-cc-pVDZ calculations reported by Rauhut18 the 2:2 complex is the most stable complex in the gas phase.
N-(α-Aminoalkyl)benzotriazoles and N-(α-aminoalkyl)-1,2,3-triazoles dissociate into stabilized ion pairs of benzotriazole or triazole anions and an immonium cation (Figure 4), which enables rapid isomerization between the 1-substituted 3A, 4A and the 2-substituted 3B, 4B isomers. We have now examined a series of N-substituted 1,2,3-triazoles in order to provide further information about their tautomeric equilibria.
RESULTS AND DISCUSSION
Preparation of N-(α-aminoalkyl)-1,2,3-triazoles
As an addition to existing literature procedures,19-21 novel N-substituted 1,2,3-triazoles 7-10 (Table 1) were prepared following procedures similar to our published method for the benzotriazoles.4,22 The reaction of 1,2,3-triazole, with an aldehyde and amine or sulfonamide in water or acetonitrile afforded compounds 7-10 in 54-95% yields (Scheme1).
Compound 13 was prepared by the reaction of (1,2,3-triazol-2-yl)methanol 11 with benzenesulfonamide 12 in 95% yield.
Deprotonation of carbazole with NaOH under reflux and nucleophilic substitution of 1-chloromethyl-1,2,3-triazole hydrochloride leads to compound 15 in 60% yield.
The reaction of saccharin 16 with paraformaldehyde and subsequent treatment with SOCl2 resulted in the formation of 18 which was then reacted with triazole to give 19 in 78% yield.
Characterization of compounds 7-10, 13, 15 and 19
The isomer ratios of triazoles 7-10, 13, 15, and 19 in CDCl3, DMSO-d6 or D2O as determined by 1H-NMR are shown in Table 1. The 13C spectral data for the same series of compounds in CDCl3 and DMSO-d6 are shown in Tables 2 and 3. The 13C spectral data for 7 and 8 in D2O are shown in Table 4. In deutoriochloroform, compounds 7-8 and 13 exist solely in the 2-substituted form, while compound 10 exists as a mixture of the 1- and 2-substituted derivatives as confirmed by the existence of two sets of signals; three signals were observed in the aromatic region at 123.9, 133.4 and 133.9 ppm corresponding to C4 and C5 carbons and two signals in the aliphatic region at 67.1 and 70.7 ppm corresponding to NCH2N.
The change from CDCl3 to the more polar DMSO for 7-8, 10 and 13 resulted in increased amounts of the 1-substituted isomers, but only the 2-isomer of 9 was detected in both solvents.
Incorporation of electron attracting substituents such as carbazole or saccharine on the nitrogen of the amino group shifted the equilibrium toward the 1H-isomer. According to 1H and 13C NMR compounds 15 and 19 exist solely in 1H form in both solvents CDCl3 and DMSO-d6.
CONCLUSIONS
A series of novel N-(α-aminoalkyl)-1,2,3-triazoles has been synthesized and the relative stabilities of their 1-and 2-isomers studied by 1H and 13C NMR. They exist solely as the 2-isomer in CDCl3 but as a mixture of the 1- and 2- isomers in more polar solvents in agreement with literature reports. Electron attracting groups on the exocyclic nitrogen shift the equilibrium towards the 1-isomer.
EXPERIMENTAL
Melting points were determined on a capillary point apparatus equipped with a digital thermometer and are uncorrected. The NMR spectra were recorded in CDCl3, DMSO-d6 or D2O with TMS for 1H (300 MHz) and 13C (75 MHz) as an internal reference.
General procedure for preparation of 7, 8 and 10
1,2,3-1H-Triazole (5.0 mmol, 1 equiv.) and amine (5.0 mmol, 1 equiv.) were mixed in water (10 mL) for 5 min. Paraformaldehyde 96% (5.0 mmol, 1 equiv) was added to the reaction mixture and kept under vigorous stirring for 4 h. The aqueous phase was extracted with ether and the organic layer was dried over MgSO4. The solvent was removed under reduced pressure to give the final products 7, 8 and 10.
4-((1H-1,2,3-Triazol-1-yl)methyl)morpholine (7A) and 4-((2H-1,2,3-triazol-2-yl)methyl)morpholine (7B). Colorless microcrystals (from toluene) (80%), mp 74-76 °C; 1H NMR (300 MHz, CDCl3) δ 7.65 (s, 2H), 5.25 (d, J=1.1 Hz, 2H), 3.69 (t, J=4.8 Hz, 4H), 2.64 (t, J=4.8 Hz 4H); 1H NMR (300 MHz, DMSO-d6) δ 8.13 (br s, 0.15H from 7A), 7.83 (d, J=2.0, 1.7H from 7B), 7.77 (br s, 0.15H from 7A), 5.26-5.24 (m, 2H from 7B and 7A), 3.55-3.52 (m, 4H from 7B and 7A), 2.51-2.44 (m, 4H from 7B and 7A). 1H NMR (300 MHz, D2O) δ 7.93 (br s, 0.34H from 7A), 7.71 (br s, 0.34H from 7A), 7.70 (s, 1.3H from 7B), 5.16 (s, 0.7H from 7A), 5.12 (s, 1.3H from 7B), 3.66-3.57 (m, 4H from 7B and 7A), 2.53-2.48 (m, 4H from 7B and 7A); Anal. Calcd for C7H12N4O: C, 49.99; H, 7.19; N, 33.31. Found: C, 50.35; H, 7.26; N, 33.04.
1-((1H-1,2,3-Triazol-1-yl)methyl)-4-methylpiperazine (8A) and 1-((2H-1,2,3-triazol-2-yl)methyl)-4-methylpiperazine (8B). Colorless microcrystals (from toluene) (54%), mp 78-80 °C; 1H NMR (300 MHz, CDCl3) δ 7.61 (s, 2H), 5.28 (s, 2H), 2.69 (brs, 4H), 2.43 (brs, 4H), 2.25 (s, 3H); 1H NMR (300 MHz, DMSO-d6) δ 8.10 (br s, 0.24H from 8A), 7.81 (1.5H from 8B), 7.75 (br s, 0.24H from 8A), 5.24 (s, 0.5H from 8A), 5.23 (s, 1.5H from 8B), 2.51-2.40 (m, 6H from 8B and 8A), 2.27 (br s, 4H from 8B and 8A), 2.11-2.09 (m, 4H from 8B and 8A); 1H NMR (300 MHz, D2O) δ 7.91 (br s, 0.5H from 8A), 7.68-7.66 (m, 1.5H from 8B and 8A), 5.15 (s, 1H from 8A), 5.11 (s, 1H from 8B), 2.56-2.38 (m, 4H from 8B and 8A), 2.06 (s, 3H from 8B and 8A); Anal. Calcd for C8H15N5: C, 53.02; H, 8.34; N, 38.64. Found: C, 53.37; H, 8.66; N, 38.82.
1-(Pyrrolidin-1-ylmethyl)-1H-1,2,3-triazole (10A) and 2-(pyrrolidin-1-ylmethyl)-2H-1,2,3-triazole (10B). Colorless needles (from CH2Cl2/hexane) (82%), mp 62-64 oC; 1H NMR (300 MHz, CDCl3) δ 7.71 (br s, 0.1H from 10A), 7.62 (br s, 1.8H from 10B and 0.1H from 10A), 5.39 (s, 1.8 from 10B), 5.33 (s, 0.2H from 10A), 2.79-2.69 (m, 4H from 10B and 10A), 1.77-1.65 (m, 4H from 10B and 10A); 1H NMR (300 MHz, DMSO-d6) δ 8.13 (s, 0.3H from 10A), 7.79 (s, 1.4H from 10B), 7.72 (s, 0.3H from 10A), 5.34 (s, 1.4H from 10B), 5.32 (s, 0.6H from 10A), 2.66-2.62 (m, 2.8H from 10B) 2.60-2.54 (m, 1.2H from 10A), 1.60-1.55 (m, 4H from 10B and 10A); Anal. Calcd for C7H12N4: C, 55.24; H, 7.95; N, 36.81. Found: C, 55.38; H, 8.44; N, 36.83.
Preparation of 9
1,2,3-1H-Triazole (0.35 g, 5.0 mmol) and aniline (0.46 mL, 5.0 mmol) were mixed in water (10 mL) for 5 min. Paraformaldehyde 96% (0.16 g, 5.0 mmol) was added to the reaction mixture and it was stirred vigorously for 4 h. The precipitate formed was filtered off and washed with ether to give 9.
N-((2H-1,2,3-Triazol-2-yl)methyl)aniline (9). Colorless prisms (from toluene) (95%), mp 97-99 °C; 1H NMR (300 MHz, CDCl3) δ 7.60 (s, 2H), 7.18 (td, J=7.6, 0.8, 2H), 6.84 (dd, J=7.8, 0.7, 2H), 6.79 (td, J=7.4, 0.8, 1H), 5.79 (d, J=7.8, 2H), 5.18-5.16 (m, 1H); 1H NMR (300 MHz, DMSO-d6) δ 7.77 (s, 2H), 7.19 (t, J=7.3 Hz, 1H), 7.09 (t, J=7.9 Hz, 2H), 6.82 (d, J=7.9 Hz, 2H), 6.61 (t, J=7.3 Hz, 1H), 5.73 (d, J=7.4, Hz 2H); Anal. Calcd for C9H10N4: C, 62.05; H, 5.79; N, 32.16. Found: C, 62.39; H, 5.79; N, 32.25.
Preparation of chloromethyl-1,2,3-triazole hydrochloride
(2H-1,2,3-Triazol-2-yl)methanol (0.25 g, 2.5 mmol) in SOCl2 (1.25 mL, 17.0 mmol) was left for 5 min and then evaporated to dryness under reduced pressure at a temperature below 25 oC. The resulting crude solid was used immediately without purification.
Preparation of 13
(2H-1,2,3-Triazol-2-yl)methanol (4.0 mmol, 1 equiv.) and benzensulfonamide (4.0 mmol, 1 equiv.) in toluene (10 mL) were stirred under reflux. The water resulting from the reaction was removed using a Dean-Stark apparatus. The reaction was considered finished when the exact volume of water (4.0 mmol, 1 equiv.) was collected. The solvent was removed under reduced pressure and the solid products were recrystallized from EtOH.
N-((1H-1,2,3-Triazol-1-yl)methyl)benzenesulfonamide (13A) and N-((2H-1,2,3-triazol-2-yl)methyl)-benzenesulfonamide(13B). Colorless microcrystals (EtOH) (95%), mp 141-142 oC; 1H NMR (300 MHz, CDCl3) δ 7.66 (m, 2H), 7.45-7.27 (m, 5H), 6.24 (br s, 1H), 7.73 (d, J=7.0 Hz, 2H), 1H NMR (300 MHz, DMSO-d6) δ 9.42 (br s, 0.5H from 16A), 7.65 (d, J=0.8 Hz, 0.25H from 16A), 7.69-7.62 (m, 0.25H from 16A), 7.60-7.52 (m, 4H from 16B and 16A), 7.51-7.49 (m, 1H from 16B and 16A), 7.47-7.38 (m, 2H from 16B and 16A), 5.63 (br s, 0.5H from 16A), 5.60 (d, J=5.4 Hz, 1.5H from 16B). Anal. Calcd for C9H10N4O2S: C, 45.37; H, 4.23; N, 23.51. Found: C, 45.77; H, 4.01; N, 23.02.
Preparation procedure for 15
To a solution of carbazole (0.42 g, 2.5 mmol) in MeCN (20 mL), NaOH powder (0.4g, 10.0 mmol) was added slowly and the mixture was heated under reflux for 2 h. To the reaction mixture freshly prepared chloromethyl-1,2,3-triazole hydrochloride in 5 mL MeCN was added and kept under reflux for an additional 4 h. The precipitate was filtered off and the filtrate was concentrated under reduced pressure to give crude product as a brown solid that was washed 3 times with cold EtOH to give 15 (0.37 g, 60%) as a white solid.
9-((2H-1,2,3-Triazol-2-yl)methyl)-9H-carbazole (15). White needles (60%), mp 198-199 oC; 1H NMR (300 MHz, CDCl3) δ 8.09 (d, J=7.6, 2H), 7.64 (d, J=7.3, 3H), 7.56-7.49 (m, 3H), 7.37-7.27 (m, 2H), 6.85 (s, 2H); 1H NMR (300 MHz, DMSO-d6) δ 8.37 (d, J=0.8, 1H), 8.15 (d, J=7.7, 2H), 7.99 (d, J=8.2, 2H), 7.69 (d, J=0.8, 1H), 7.52 (tripletoidd, J=7.0, 8.4, 1.1, 2H), 7.27 (t, J=7.1, 2H), 7.12 (s, 2H); Anal. Calcd for C15H12N4: C, 72.56; H, 4.87; N, 22.57. Found: C, 72.39; H, 4.82; N, 22.60.
Preparation of 17
A mixture of saccharin (1.83 g, 10.0 mmol) and formalin 37% (2.43 mL, 30.0 mmol) in water (20 mL) was heated under reflux for 30 min. After storing at 5 °C overnight, the solid was filtered off and dried to produce N-hydroxymethyl saccharin (2.08 g, 98%) as a white pure solid.
N-Hydroxymethylsaccharin (17). White solid (98%), mp 124-126 oC (lit.,23 mp 136-137 oC) 1H NMR (300 MHz, DMSO-d6) δ 8.30 (d, J=7.3 Hz, 1H), 8.14 (d, J=7.0 Hz, 1H), 8.07 (td, J=7.4, 1.4 Hz, 1H), 8.00 (td, J=7.4, 1.1 Hz, 1H), 5.17 (s, 2H); 1H NMR (75 MHz, DMSO-d6) δ 158.5, 137.2, 136.1, 135.2, 126.1, 125.3, 121.5, 62.8; Anal. Calcd for C8H7NO4S: C, 45.07; H, 3.31; N, 6.57. Found: C, 45.12; H, 3.39; N, 6.44.
Preparation of 18
A mixture of N-hydroxymethylsaccharin (1.00 g, 4.7 mmol) and thionyl chloride (3.00 mL, mmol) was heated under reflux and under anhydrous conditions. After 10 minutes, the residual oil was evaporated free of excess thionyl chloride and dried overnight to obtain N-chloromethylsaccharin (1.06 g, 98%) as a yellow pure solid.
N-Chloromethylsaccharin (18). Yellow solid (98%) mp 137-138 oC (lit.,23 mp 137-138 oC). 1H NMR (300 MHz, DMSO-d6) δ 8.40 (d, J=7.6 Hz, 1H), 8.20 (d, J=7.6 Hz, 1H), 8.13 (td, J=7.6, 1.1 Hz, 1H), 8.05 (td, J=7.6, 1.1 Hz, 1H), 5.83 (s, 2H); 1H NMR (75 MHz, DMSO-d6) δ 157.5, 136.7, 136.7, 135.6, 125.7, 125.5, 121.9, 46.4. Anal. Calcd for C8H7ClNO3S: C, 41.48; H, 2.61; N, 6.05. Found: C, 41.56; H, 2.92; N, 5.93.
Preparation of 19
A mixture of 1,2,3-1H-triazole (0.10 g, 1.4 mmol) and NaOH (0.06 g, 1.4 mmol) in MeCN (7 mL) was heated under reflux. After 30 minutes, the heating was stopped and N-chloromethylsaccharine (0.303g, 1.4 mmol) dissolved in MeCN (5 mL) was added. The reaction was cooled at room temperature and stirred for 2 h. The solid was filtered and the filtrate was concentrated under reduced pressure. Theresidue was dissolved in CH2Cl2 (15 mL), and the suspension was filtered thorough celite. The clear solution was concentrated under reduced pressure. The white solid was recrystallized from EtOH to give N-(triazol-1-yl)methylsaccharin (0.30 g, 78%) as colorless needles.
N-(Triazol-1-yl)methylsaccharin (19). Colorless needles (78%), mp 168-170 oC; 1H NMR (300 MHz, DMSO-d6) δ 8.36 (d, J=7.1 Hz, 1H), 8.26 (d, J=1.0 Hz, 1H), 8.18 (dd, J=7.3, 0.8 Hz, 1H), 8.09 (td, J=7.4, 1.2 Hz, 1H), 8.02 (td, J=7.4, 1.1 Hz, 1H), 7.80 (d, J=1.0 Hz, 1H), 6.43 (s, 2H); Anal. Calcd for C10H8N4O3S: C, 45.45; H, 3.05; N, 21.20. Found: C, 45.73; H, 2.95; N, 20.93.
ACKNOWLEDGEMENTS
We thank Dr. Hall for his help and useful suggestions.
References
1. J. R. Lindsay Smith and J. S. Sadd, J. Chem. Soc., Perkin Trans. 1, 1975, 12, 1181. CrossRef
2. A. R. Katritzky, K. Yannakopoulou, W. Kuzmierkiewicz, J. M. Aurrecoechea, G. J. Palenik, A. E. Koziol, and M. Szczesniak, J. Chem. Soc., Perkin Trans. 1, 1987, 12, 2673. CrossRef
3. F. Tomás, J.-L. M. Abboud, J. Laynez, R. Notario, L. Santos, S. O. Nilsson, J. Catalán, R. M. Claramunt, and J. Elguero, J. Am. Chem. Soc., 1989, 111, 7348. CrossRef
4. A. R. Katritzky and K. Yannakopoulou, Heterocycles, 1989, 28, 1121. CrossRef
5. A. R. Katritzky, Z. Najzarek, and Z. Dega-Szafran, Synthesis, 1989, 1, 66. CrossRef
6. M. Breza and V. Milata, Arkivoc, 2005, 9, 80.
7. M. Perez-Torralba, R. M. Claramunt, I. Alkorta, and J. Elguero, Arkivoc, 2007, 12, 55.
8. A. N. Nesmeyanov, V. N. Babin, L. A. Fedorov, M. I. Rybinskaya, and E. I. Fedin, Tetrahedron, 1969, 25, 4667. CrossRef
9. J. Elguero, C. Martin, A. R. Katritzky, and P. Linda, 'The Tautomerism of Heterocycles,' Academic Press, New York, 1976, pp 283.
10. F. Tomas, J. Catalan, P. Perez, and J. Elguero, J. Org. Chem., 1994, 59, 2799. CrossRef
11. M. Begtrup, C. J. Nielsen, L. Nygaard, S. Samdal, C. E. Sjøgren, and G. O. Sørensen, Acta Chem. Scand., 1988, 500. CrossRef
12. Z. Zhou, R. Liu, J. Wang, S. Li, M. Liu, and J.-L. Brédas, J. Phys. Chem. A, 2006, 110, 2322. CrossRef
13. L. Lunazzi, F. Parisi, and D. Macciantelli, J. Chem. Soc. Perkin Trans 2, 1984, 6, 1025. CrossRef
14. M. Begtrup, J. Chem. Soc. Perkin Trans. 2, 1976, 6, 736. CrossRef
15. A. Albert and P. J. Taylor, J. Chem. Soc. Perkin Trans 2, 1989, 11, 1903. CrossRef
16. P. I. Nagy, F. R. Tejada, and W. S. Messer Jr., J. Phys. Chem. B, 2005, 109, 22588. CrossRef
17. J.-L. M. Abboud, C. Foces-Foces, R. Notario, R. E. Trifonov, A. P. Volovodenko, V. A. Ostrovskii, I. Alkorta, and J. Elguero, Eur. J. Org. Chem., 2001, 16, 3013. CrossRef
18. G. Rauhut, Phys. Chem. Chem. Phys., 2003, 5, 791. CrossRef
19. S. Kamijo, T. Jin, Z. Huo, and Y. Yamamoto, J. Org. Chem., 2004, 69, 2386. CrossRef
20. S. W. Kwok, J. E. Hein, V. V. Fokin, and K. B. Sharpless, Heterocycles, 2008, 76, 1141. CrossRef
21. D.-R. Hou, S. Alam, T.-C. Kuan, M. Ramanathan, T.-P. Lin, and M.-S. Hung, Bioorg. Med. Chem. Lett., 2009, 19, 1022. CrossRef
22. A. R. Katritzky, G. Yao, X. Lan, and X. Zhao, J. Org. Chem., 1993, 58, 2086. CrossRef
23. W. A. Siddiqui, S. Ahmad, I. U. Khan, H. L. Siddiqui, and G. W. Weaver, Synth. Commun., 2007, 37, 767. CrossRef