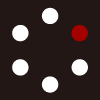
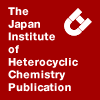
HETEROCYCLES
An International Journal for Reviews and Communications in Heterocyclic ChemistryWeb Edition ISSN: 1881-0942
Published online by The Japan Institute of Heterocyclic Chemistry
e-Journal
Full Text HTML
Received, 26th August, 2010, Accepted, 14th September, 2010, Published online, 15th September, 2010.
DOI: 10.3987/COM-10-S(E)114
■ De Novo Asymmetric Approach to the Disaccharide Portion of SCH-47554
Xiaomei Yu, Miaosheng Li,* and George A. O'Doherty*
Department of Chemistry, West Virginia University, Morgantown, WV 26506-6045, U.S.A.
Abstract
A method for the asymmetric synthesis of the disaccharide portion of SCH-47554 has been developed in 6 steps. The route is shorter than the reported route to a related disaccharide. The route involves the use of the Noyori reduction to establish the asymmetry of the D- and L-sugar portion of the molecule. Diastereoselective Pd-glycosylation reaction and subsequent post-glycosylation transformation are used to establish the remaining stereocenter.INTRODUCTION
The angucycline family of antibiotics have been of interest to both the synthetic and biological communities due to their unique structures and potent antifungal, antibacterial and antitumor activities.2 The structural diversity of this class of natural products is nicely typified by the trisaccharide natural product: SCH-47554 (1).3 SCH-47554 (1) was isolated by Schering-Plough from the fermentation broths of a strain of Streptomyces sp. (SCC-2136) and gained particular interest because of its display of antifungal activity against a variety of yeasts and dermatophytes. Like all of the angucycline antibiotics, SCH-47554 shares a β-C-glycoside linked sugar ortho to the phenol on the anthroquinone portion of the aglycone. A less common feature of SCH-47554 as compared to the typical angucyclines, is that its C-glycoside disaccharide portion consists of the rare β-D-amicetose and α-L-aculose sugars (Scheme 1).
As part of our ongoing interest in the synthesis and study of this class of natural products we became interested in the synthesis and study of the disaccharide portion of SCH-47554.4 Retrosynthetically, we envisioned that SCH-47554 could be prepared by a convergent annulation of arylbromide 2 with a suitably metalated fragment like 3. In turn, we hoped bromide 2 could be prepared from the direct C-glycosylation of 4 with bromo-phenol 5. In turn disaccharide 4 could be prepared from pseudo-enantiomeric pyranones 6 and 7. It is worth noting that a route to a molecule similar to disaccharide 2 was reported by Barrett.5 We set out to try to prepare a similar disaccharide (4 and/or 2) via our de novo methodology in significantly fewer steps from 2-acylfuran. Herein we report our successful efforts at the de novo synthesis of disaccharide 4, as well as our unsuccessful efforts to couple it with 5 to form C-aryl disaccharide 2.
RESULTS AND DISCUSSION
Previously, we have described a stereospecific Pd-catalyzed glycosylation reaction, which uses glycosyl-donors α-L-pyranones 7 and α-D-pyranones (ent)-7.6 The enantiomeric donors are readily prepared in 3 steps from acyl furan 8 (Scheme 2). The stereodivergent route employs an enantioselective Noyori reduction (8 to 9(R)/ 9(S)),7,8 an Achmatowicz oxidation and diastereoselective t-butyl carbonate formation (Scheme 2).9 By this route, the α-L-pyranone 7 could be isolated in ~50% overall yield, when the Boc-protection was performed selectively prepared in 82% (α:β = 3:1) at low temperature (–78 °C). For our purposes, we needed a more acid stable anomeric leaving group that a t-butyl carbonate group for our desired C-glycosylation. To these ends we decided to prepare in an analogous fashion the α-D-pyranone 6 with an anomeric pivalate group in similar yields and slightly better selectivity (α:β = 10:1).
The synthesis of the desired α-D-amiceto-sugar 11 began with a Luche reduction (NaBH4/CeCl3) of pyranone 6, which diastereoselectively gave allylic alcohol 10 (68% yield) with the requisite C-4 equatorial alcohol (Scheme 3). A diimide reduction (excess o-nitrobenzenesulfonylhydrazide/Et3N) was then preformed to remove the pyran double bond yielding the D-amiceto-sugar 11 (80%). Previously, we had found that a significant amount of hydrogenolysis of the allylic bonds can occur under typical hydrogenation conditions.10 Our investigation into a cobalt catalyzed one-pot concomitant 1,4-/1,2-reduction of the enone 6 to the equatorial alcohol 11 led only to decomposition.
We turned to the palladium glycosylation condition to install the L-sugar of the desired disaccharides 4 (Scheme 4). Exposure of 11 to a slight excess of α-L-Boc-pyranone 7 under our typical Pd-catalyzed glycosylation conditions (2.5% Pd2(dba)3•CHCl3/10% PPh3)11 gave a good yield of disaccharide 4 (70% yield). Finally, another Luche reduction (NaBH4/CeCl3) of pyranone 4 followed by acylation (Ac2O/DMAP) gave the backup disaccharide 12 in 70% for the two steps.
With the two D,L-disaccharides 4 and 12 in hand, we explored the use of various Lewis acids to promote its C-glycosylation coupling with phenol 5 (Scheme 5). Unfortunately, all the Lewis acids (TiCl4, Ti(Oi-Pr)4, SnCl4, BF3, and TMSOTf) used for the coupling of 5 and the D,L-disaccharides 4 led to decomposition of the disaccharide 4. Similarly, when the D,L-disaccharides 12 was used, the only coupling products detected were structure consistent with C-O glycosidic bond cleavage.
In conclusion highly efficient and stereocontrolled synthesis of the amicetose/aculose disaccharide 12 was achieved in 6 steps from acylfuran. This route to the disaccharide portion of SCH47554 was significantly shorter than previous carbohydrate based approaches. Preliminary efforts to transfer the disaccharide to napthol containing molecules proved to be unsuccessful. Future works along these lines are ongoing and will be reported in due course.
EXPERIMENTAL
General Methods and Materials. 1H and 13C NMR spectra were recorded on 270 MHz, and 600 MHz spectrometers. Chemical shifts were reported relative to internal tetramethylsilane (δ 0.00) or CDCl3 (δ 7.26) for 1H NMR and CDCl3 (δ 77.0) for 13C NMR. Infrared (IR) spectra were obtained on FT-IR spectrometer. Optical rotations were measured with a digital polarimeter in the solvent specified. Flash column chromatography was performed on 60-200 mesh silica gel. Analytical thin-layer chromatography was performed with precoated glass-backed plates (60 Å, F254) and visualized by quenching of fluorescence and by charring after treatment with p-anisaldehyde or phosphomolybdic acid or potassium permanganate stain. Rf values are obtained by elution in the stated solvent ratios (v/v). Ether (Et2O), THF, methylene chloride (CH2Cl2) and triethylamine (Et3N) were dried by passing through activated alumina columns with argon gas pressure. Commercial reagents were used without purification unless otherwise noted. Air- and/or moisture-sensitive reactions were carried out under an atmosphere of argon/nitrogen using oven-dried glassware and standard syringe/septa techniques.
(6R)-6-PIVALOYLOXY-(2R)-2-METHYL-2H-PYRAN-3(6H)-ONE (6).
(6R)-6-Hydroxy-(2R)-2-methyl-2H-pyran-3(6H)-one (2.22 g, 8.59 mmol), 30 mL of CH2Cl2, and 1.5 mL of Et3N were added to a round bottom flask and cooled to –78 °C. A catalytic amount (53 mg, 0.43 mmol) of DMAP and pivaloyl chloride (1.06 mL, 8.59 mmol) were added and the solution was stirred for 3 h at –78 °C. The reaction was quenched with 50 mL of sat. aq. NaHCO3, extracted (3 x 50 mL) with Et2O, dried (Na2SO4), and concentrated under reduced pressure. The crude product was purified using silica gel flash chromatography eluting with 10 % EtOAc/hexanes to give 2.41 g (7.04 mmol, 82 %) of 6. Rf (20 % EtOAc/hexanes) = 0.4; [α]21D –66 (c 0.1, CH2Cl2); IR (thin film, cm-1) 2935, 2910, 2855, 1738, 1692, 1461, 1399, 1279; 1H NMR (600 MHz, CDCl3) δ 6.88 (dd, J = 10.2, 3.6 Hz, 1H), 6.46 (d, J = 3.6 Hz, 1H), 6.19 (d, J = 10.2 Hz, 1H), 4.57 (q, J = 6.6 Hz, 1H), 1.40 (d, J = 6.6 Hz, 3H), 1.23 (s, 9H), 13C NMR (150 MHz, CDCl3) δ 195.9, 177.0, 141.8, 128.2, 86.9, 72.3, 39.2, 27.0, 15.4; CI HRMS calculated for [C11H16O4]+: 212.1049. Found 212.1045.
((2R,5S,6R)-5,6-DIHYDRO-5-HYDROXY-6-METHYL-2H-PYRAN-2-YL PIVALATE (10).
A solution of pyranone 6 (580 mg, 2.74 mmol) in CH2Cl2 (1.5 mL) and 0.4 M CeCl3/MeOH (1.5 mL) was cooled to –78 °C. NaBH4 (58.4 mg, 1.54 mmol) was added and the reaction mixture was stirred for 4 h at –78 °C. The resulting solution was diluted with ether (10 mL), quenched with 5 mL of saturated NaHCO3, extracted (3 x 5 mL) with Et2O, dried (Na2SO4), and concentrated under reduced pressure. The crude product was purified using silica gel chromatography eluting with 40% EtOAc/hexane to give enol 10 (400 mg, 1.90 mmol, 68%) as a white solid, mp: 62.5-64.0 °C; Rf = 0.30 (40% EtOAc/Hexane); [α]21D (c 0.55, CH2Cl2); IR (thin film, cm-1) 3391, 2972, 2933, 2837, 1720, 1612, 1513, 1246, 1029, 999, 819; 1H NMR (600 MHz, CDCl3) δ 6.21 (dd, J = 2.4, 1.8 Hz, 1H), 6.04 (d, J = 9.6 Hz, 1H), 5.75 (ddd, J = 10.2, 2.4, 2.4 Hz, 1H), 3.90 (d, J = 8.4 Hz, 1H), 3.72 (m, 1H), 2.20 (s, 1H), 1.31 (d, J = 6.6 Hz, 3H), 1.20 (s, 9H); 13C NMR (150 MHz, CDCl3) δ 177.7, 134.7, 125.1, 88.4, 70.3, 69.2, 39.1, 27.1, 18.0; CIHRMS: Calculated for [C11H18O4Na+]: 237.1102. Found: 237.1099.
((2R,5S,6R)-3,4,5,6-TETRAHYDRO-5-HYDROXY-6-METHYL-2H-PYRAN-2-YL PIVALATE (11).
A solution of allylic alcohol 10 (370 mg, 1.73 mmol) was dissolved in CH2Cl2 (11 mL) at room temperature. Ortho-nitrobenzenesulfonylhydrazide (1.33 g, 6.55 mmol) and Et3N (0.88 g, 8.74 mmol) were added while stirring. The reaction was tracked by TLC. After 12 h, the reaction was quenched by adding 10 mL of satd. aq. NaHCO3, extracted (3 x 50 mL) with methylene chloride, dried over Na2SO4, and concentrated under reduced pressure. The crude product was purified using silica gel flash chromatography eluting with 30% EtOAc/hexanes to give 11 (420 mg, 1.09 mmol, 80%) as a yellow oil. Rf (50% EtOAc/hexanes) = 0.15; [α]21D –105 (c = 1.0, CH2Cl2); IR (thin film, cm-1) 3390, 2933, 1724, 1443, 1368, 1246, 1166, 1114, 1066, 1011, 940, 818; 1H NMR; 1H NMR (600 MHz, CDCl3) δ 6.01 (s, 1H), 6.04 (d, J = 9.6 Hz, 1H), 3.65 (dq, J = 9, 6.6, 1H), 3.30 (ddd, J = 10.2, 5.4, 1.8, 1H), 1.98-1.65 (m, 4H), 1.23 (d, J = 6.6 Hz, 3H), 1.20 (s, 9H); 13C NMR (150 MHz, CDCl3) δ 177.0 , 90.7, 71.8, 71.5, 39.1, 28.5, 27.2, 27.0, 17.9; CIHRMS: Calculated for [C11H20O4Na+]: 239.1259. Found: 239.1264.
((2R,5S,6R)-4-((2’S,6’R)-5,6-DIHYDRO-6-METHYL-5-OXO-2H-PYRAN-2-YLOXY)-3,4,5,6-TETRAHYDRO-5-HYDROXY-6-METHYL-2H-PYRAN-2-YL PIVALATE (4).
A CH2Cl2 (1.0 mL) solution of Boc pyranone 7 (422 mg, 1.85 mmol) and alcohol 11 (200 mg, 0.93 mmol) was cooled to 0 °C. Pd2(DBA)3•CHCl3 (24 mg, 2.5 mol%) and PPh3 (21 mg, 10 mol%) were added to the reaction mixture at 0 °C. After stirring at room temperature for 8 h, the reaction mixture was quenched with 10 mL of satd. aq. NaHCO3, extracted (3 x 10 mL) with Et2O, dried over Na2SO4 and concentrated under reduced pressure. The crude product was purified using silica gel flash chromatography eluting with 20% EtOAc/hexanes to give pyranone 4 (210 mg, 0.65 mmol, 70%) as a yellow oil: Rf (30% EtOAc/hexanes) = 0.5; [α]21D –105 (c 1.0, CH2Cl2); IR (thin film, cm-1) 2933, 1731, 1701, 1443, 1368, 1246, 1166, 1114, 1066, 1011, 940, 818; 1H NMR; 1H NMR (600 MHz, CDCl3) δ 6.75 (dd, J = 10.2, 3.6, 1H), 6.04 (d, J = 10.2, 1H), 5.98 (s, 1H), 5.25 (d, J = 9.6 Hz, 1H), 4.55 (q, J = 6.6, 1H), 3.73 (dq, J = 9, 6.6, 1H), 3.30 (m, 1H), 2.09-2.01 (m, 2H), 1.89-1.79 (m, 2H), 1.33 (d, J = 6.6 Hz, 3H), 1.19 (d, J = 6.6 Hz, 3H), 1.20 (s, 9H); 13C NMR (150 MHz, CDCl3) δ 196.4, 176.6, 142.7, 127.2, 94.7, 90.4, 80.2, 70.2, 69.8, 38.9, 28.2, 26.9, 25.8, 17.9, 15.0; CIHRMS: Calculated for [C17H26NaO6+]: 349.3745. Found: 349.3750.
((2R,5S,6R)-4-((2’S,6’R)-5,6-DIHYDRO-5-(4-ACETATE)-6-METHYL-2H-PYRAN-2-YLOXY)- 3,4,5,6-TETRAHYDRO-5-HYDROXY-6-METHYL-2H-PYRAN-2-YL PIVALATE (12).
A solution of pyranone 4 (50 mg, 2.74 mmol) in CH2Cl2 (1.5 mL) and 0.4 M CeCl3/MeOH (1.5 mL) was cooled to –78 °C. NaBH4 (58.4 mg, 1.54 mmol) was added and the reaction mixture was stirred for 4 h at –78 °C. The resulting solution was diluted with ether (10 mL), quenched with 5 mL of saturated NaHCO3, extracted (3 x 5 mL) with Et2O, dried (Na2SO4), and concentrated under reduced pressure. The crude product was dissolved in CH2Cl2 (1.5 mL) and DMAP (10 mg) and acetic anhydride (2 mL) was added. After 10 min the reaction was quenched by adding of Et2O (5 mL) and 10 mL of satd. aq. NaHCO3. Resulting mixture was extracted (3 x 10 mL) with Et2O, dried over Na2SO4 and concentrated under reduced pressure. The crude product was purified using silica gel flash chromatography eluting with 20% EtOAc/hexanes to give allylic acetate 12 (65 mg, 0.65 mmol, 70%) as a yellow oil: Rf (30% EtOAc/hexanes) = 0.5; [α]21D –65 (c 1.0, CH2Cl2); IR (thin film, cm-1) 2933, 1731, 1725, 1443, 1368, 1246, 1166, 1114, 1066, 1011, 940, 818; 1H NMR; 1H NMR (600 MHz, CDCl3) δ 6.02 (s, 1H), 5.85 (d, J = 10.2, 1H), 5.77 (ddd, J = 10.2, 2.4, 2.4 1H), 5.05 (m, 1H), 5.03 (m, 1H), 3.99 (dq, J = 9.6, 6.6, 1H), 3.76 (dq, J = 9.6, 6.6, 1H), 3.30 (m, 1H), 2.06 (s, 3H), 1.89-1.79 (m, 2H), 1.20 (d, J = 6.6 Hz, 3H), , 1.21 (s, 9H) 1.19 (d, J = 6.6 Hz, 3H); 13C NMR (150 MHz, CDCl3) δ 177.1, 170.7, 130.2, 127.6, 96.3, 91.0, 80.1, 71.0, 70.5, 65.0, 39.3, 28.7, 27.3, 26.6, 21.2, 18.3, 18.1; CIHRMS: Calculated for [C19H30NaO7+]: 393.4271. Found: 393.4269.
ACKNOWLEDGEMENTS
We are grateful to Kung Wang for his supervision of this project. We are grateful to NIH (GM088839 & 090259) and the NSF (CHE-0749451) for their financial support of our research program.
References
1. This paper is dedicated to Professor Albert Eschenmoser on the occasion of his 80th birthday.
2. (a) J. Rohr and R. Thiericke, Nat. Prod. Rep., 1992, 9, 103; CrossRef (b) K. Krohn and J. Rohr, Top. Curr. Chem., 1997, 188, 127; CrossRef (c) L. Andriy, V. Andreas, and B. Andreas, Mol. BioSyst., 2005, 1, 117. CrossRef
3. For the isolation of SCH-47554, see: M. Chu, R. Yarborough, J. Schwartz, M. G. Patel, A. C. Horan, V. P. Gullo, P. R. Das, and M. S. Puar, J. Antibiot., 1993, 46, 861.
4. For our synthesis as biological study of related molecules see: X. Yu and G. A. O’Doherty, Org. Lett., 2008, 10, 4529. CrossRef
5. The Barrett route to a related disaccharide occurred in 21 total steps from D-glucose and L-rhamnose. G. E. Morton and A. G. M. Barrett, Org. Lett., 2006, 8, 2859. CrossRef
6. (a) M. Shan, Y. Xing, and G. A. O’Doherty, J. Org. Chem., 2009, 74, 5961; CrossRef (b) H. Guo and G. A. O’Doherty, J. Org. Chem., 2008, 73, 5211; CrossRef (c) M. Zhou and G. A. O’Doherty, Org. Lett., 2008, 10, 2283; CrossRef (d) M. Zhou and G. A. O’Doherty, J. Org. Chem., 2007, 72, 2485. CrossRef
7. A. Fujii, S. Hashiguchi, N. Uematsu, T. Ikariya, and R. Noyori, J. Am. Chem. Soc., 1996, 118, 2521. CrossRef
8. M. Li, J. G. Scott, and G. A. O’Doherty, Tetrahedron Lett., 2004, 45, 1005; CrossRef (b) M. Li and G. A. O’Doherty, Tetrahedron Lett., 2004, 45, 6407. CrossRef
9. (a) R. S. Babu and G. A. O’Doherty, J. Carb. Chem., 2005, 24, 169; (b) H. Guo and G. A. O’Doherty, Org. Lett., 2005, 7, 3921. CrossRef
10. M. H. Haukaas and G. A. O’Doherty, Org. Lett., 2002, 4, 1771. CrossRef
11. (a) R. S. Babu, M. Zhou, and G. A. O’Doherty, J. Am. Chem. Soc., 2004, 126, 3428; CrossRef (b) R. S. Babu and G. A. O’Doherty, J. Am. Chem. Soc., 2003, 125, 12406. CrossRef