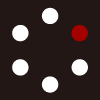
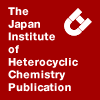
HETEROCYCLES
An International Journal for Reviews and Communications in Heterocyclic ChemistryWeb Edition ISSN: 1881-0942
Published online by The Japan Institute of Heterocyclic Chemistry
e-Journal
Full Text HTML
Received, 15th April, 2010, Accepted, 14th May, 2010, Published online, 17th May, 2010.
DOI: 10.3987/COM-10-S(E)19
■ Studies on the Biosynthesis of the Stephacidins and Notoamides. Total Synthesis of Notoamides
Timothy J. McAfoos, Shengying Li, Sachiko Tsukamoto, Daivd H. Sherman, and Robert M. Williams*
Department of Chemistry, Colorado State University, Fort Collins, CO 80523-1872, U.S.A.
Abstract
Notoamide S has been suggested to be the final common precursor between two different Aspergillus sp. fungal strains before diverging to form enantiomerically opposite natural products (+)- and (–)-stephacidin A and (+)- and (-)-notoamide B. The synthesis of notoamide S comes from the coupling of N-Fmoc proline with a 6-hydroxy-7-prenyl-2-reverse prenyl tryptophan derivative that was synthesized via a late stage Claisen rearrangement from a 6-propargyl-2-reverse prenylated indole.INTRODUCTION
The paraherquamide,1 brevianamide,2 stephacidin,3 and notoamide4 families of prenylated indoles are of great interest to chemists and biologists because of their structural complexity and their biological properties; in particular, the members of these families containing the unique bicyclo[2.2.2]diazaoctane ring system have drawn the most attention. Since the isolation of (+)-stephacidin A (2) in 2002 by Bristol-Myers Squibb from Aspergillus ochraceus,3 the biosynthetic pathway of the stephacidin family of alkaloids has been of particular interest to our laboratories.5 (+)-Stephacidin A was also subsequently identified as a co-metabolite with several new prenylated indole alkaloids, the notoamides, by Tsukamoto and co-workers from a marine derived Aspergillus sp.4 Our interest in the biosynthesis of the stephacidin and notoamide families was heightened when the corresponding enantiomer, (–)-stephacidin A (4), was isolated from a terrestrial fungi, Aspergillus versicolor that was found growing in a Hawaiian forest (Figure 1).6
This most intriguing discovery lead to the as of yet unanswered biosynthetic question, at what point does the biosynthetic pathway of these fungi diverge to produce these two enantomerically distinct secondary metabolites? This assumes of course, that the biosynthesis of stephacidin in each respective species of the Aspergillus sp. are related through a common intermediate. Previous work by Sammes7 and Birch,8 as well as from our laboratories,9 has shown that the bicyclo[2.2.2]diazaoctane ring system found in many of these natural products likely arises via a biosynthetic intramolecular Diels-Alder of an azadiene. One postulate concerning the enantiomeric selectivity seen in each fungus is that the facial selectivity during the key intramolecular Diels-Alder is respectively, enantioselective in the two fungi.10 If the intramolecular Diels-Alder is the point of stereochemical divergence, then both fungi could potentially produce the same achiral azadiene intermediate, which in turn is believed to be produced via a common biosynthetic pathway from a mutual precursor. During our genome-based characterization experiments of the marine-derived Aspergillus sp. aimed at elucidation of each step of the biosynthesis, it was shown that brevianamide F (5) undergoes a reverse prenylation at the C2 position of the indole to give deoxybrevianamide E (6) by a reverse prenyl transferase NotF (Scheme 1).11
After oxidation of deoxybrevianamide E at C6, it was found that a prenylation takes place at C7 via the prenyl transferase NotC giving a previously undiscovered metabolite 8, which we have named notoamide S.11 We identified notoamide S (8) by 1H and 13C NMR as a metabolite constituted of two isoprene units, tryptophan and proline residues but lacking the bicyclo[2.2.2]diazaoctane ring system and pyranyl indole ring, as the possible precursor to the achiral azadiene.11 Previously, we learned that notoamide E (9) is not a biosynthetic precursor to stephacidin A (2) through labeled precursor incorporation experiments, mandating that the putative intramolecular Diels-Alder preceeds the formation of the pyran ring. This narrowed the realm of possible precursors from 7 to stephacidin, suggesting that notoamide S is potentially the penultimate metabolite leading to the achiral azadiene, which can be transformed to either (+) or (–)-stephacidin A (Scheme 2).12 In order to confirm the structure of natural 8, an authentic sample was required; herein we detail the total synthesis of notoamide S (8).
RESULTS AND DISCUSSION
We envisioned that, notoamide S (8) could come from the coupling of commercially available N-Fmoc L-proline (12) with the 6-hydroxy-7-prenyl-2-reverseprenyl tryptophan derivative (11). This tryptophan derivative can be derived from the corresponding 6-hydroxy-7-prenyl-2-reverseprenyl indole (13), which can be synthesized from the known indole 1413 (Scheme 3).
The assembly of notoamide S began from the previously reported indole 14.13 The treatment of 14 with Lindlar’s catalyst under an atmosphere of hydrogen resulted in partial reduction of the alkyne (Scheme 4). The propargylic ether was heated under microwave conditions in toluene to give the desired 6-hydroxy-7-prenyl-2-reverseprenyl indole 13. Indole 13 was treated with dimethyl amine and aqueous formaldehyde in acetic acid to give the desired gramine 15, however in only 20% yield.
With the poor yield of the 15 it was decided to reverse the order of steps to see if the desired gramine could be synthesized in a higher yield. Formation of gramine 16 from 14 was followed by reduction and subsequent Claisen rearrangement to give the desired gramine 15 in a 71% overall yield (Scheme 5). Somei14-Kametani,15 coupling of 15 with N-(diphenylmethylene)glycine ethyl ester in the presence of tributylphosphine followed by deprotection of the incipient benzophenone imine gave only a 10% yield of the desired tryptophan derivative 17. It is believed that the free phenol is problematic in this reaction due to the acidity of the phenolic proton, therefore a different approach was adopted to avoid this problem.
With 14 being readily available on multi-gram scale, we decided to explore the use of the propargyl group as a temporary protecting group for the phenol by performing the reduction and Claisen rearrangement at a later stage (Scheme 6). Under Somei-Kametani14,15 conditions 16 can be converted to the desired tryptophan derivative which upon removal of the benzophenone imine with aqueous acid gave the free amine 18. Coupling of 18 with N-Fmoc L-proline (12) in the presence of 2-(7-aza-1H-benzotriazole-1-yl)-1,1,3,3-tetramethyluronium hexafluorophosphate (HATU) and diisopropylethyl amine gave the coupled product 19 in 85% yield. However, after removal of the Fmoc group in 19, the cyclization to the diketopiperazine was also accompanied by undesired pyran formation to give the natural product notoamide E (9) and epi-notoamide E (20) (Scheme 6).
Although unanticipated, the adventitious synthesis of notoamide E is three steps shorter than our previously reported route starting from the same indole substrate 14.16 To obviate the formation of the pyran ring it was decided to reduce the alkyne to the alkene prior to the formation of the dioxopiperazine, thus allowing for the possibility of performing the desired Claisen and dioxopiperazine formation in one operation. Reduction of 19 with Lindlar’s catalyst gave the desired alkene in quantitative yield. With the full skeleton in hand all that remained was cleavage of the Fmoc group of the proline residue followed by cyclization to the dioxopiperazine ring (Scheme 7). As shown previously with the formation of the pyran ring, it was believed that the Claisen rearrangement of the reverse prenyl ether would take place during the cyclization of the dioxopiperazine. Removal of the Fmoc group proceeded smoothly in the presence of diethylamine, and upon refluxing in toluene the desired compound 8 (notoamide S) was isolated in a modest yield (18%); however, three other products were also found to be present in the mixture. These include: epi-notoamide S (21) followed by notoamide E and epi-notoamide E. Further investigation revealed that the formation of the pyran ring was occurring during the Somei-Kametani14,15 reaction, and these inseparable by-products were invariably carried through the synthesis. Suppression of this undesired side reaction can be achieved by lowering the temperature of the Somei-Kametani14,15 reaction below reflux. Under these conditions, notoamide S was obtained in 29% isolated yield along with 21 (20%), 9 (8%) and 20 (5%). The synthetic notoamide S exactly matched the natural metabolite obtained from the marine-derived Aspergillus sp. (Tsukamoto) by 1H nmr and retention time on LC.11
CONCLUSION
The synthesis of notoamide S (8) was accomplished in seven steps from known indole 14 in 19% overall yield. Synthetic access to this potentially pivotal intermediate, will allow us to further interrogate the steps from notoamide S to the distinct natural enantiomers of stephacidin A and notoamide B. These studies are in progress and will be reported in due course.
EXPERIMENTAL
General. Unless otherwise noted, all materials were obtained from commercial sources and used without purification. All reactions were carried out under anhydrous conditions, unless otherwise specified, and performed under positive pressure of argon using flame-dried glassware. Dichloromethane, acetonitrile, toluene, and tetrahydrofuran were degassed with argon and dried through a solvent purification system (J. C. Meyer of Glass Contour). Flash chromatography was performed on standard grade silica gel (230 x 400 mesh) from Sorbent Technologies with the indicated solvent. Microwave reactions were run using a CEM Discover set to a constant temperature and allowing for variable power. 1H NMR and 13C NMR spectra were recorded on Varian 300 and 400 MHz spectrometers as indicated. Chemical shifts are reported in parts per million downfield from tetramethylsilane. Peak multiplicities are denoted s (singlet), bs (broad singlet), d (doublet), t (triplet), m (multiplet) or by a combination of these e.g. dd (doublet of doublets). Infrared spectra were recorded on a Bruker Tensor 27 IR spectometer on NaCl plates. Mass spectra were obtained at the Colorado State University CIF on a Fisons VG Autospec.
7-(3-Methylbut-2-en-1-yl)-2-(2-methylbut-3-en-2-yl)-1H-indol-6-ol (13). To 14 (100 mg, 0.37 mmol, 1 eq) in MeOH (3 mL) was added Lindlar’s catalyst (10 mg, 10 wt %) at rt followed by purging with a balloon of hydrogen gas. The reaction was vigorously stirred for 30 min. The reaction was monitored by NMR for loss of alkyne proton. The reaction was then filtered through a plug of Celite and concentrated under reduced pressure to give a crude yellow oil. The resultant crude yellow oil was then taken up in toluene (3 mL) and the reaction was heated to 150 ºC in a microwave reactor for 30 min. The reaction was then concentrated under reduced pressure to give the crude 13 as a brown oil. The residue was purified by flash chromatograph (AcOEt/hexane, 1:20) to give indole 13 (87 mg, 87%) as an off white solid. Rf = 0.38 (AcOEt/hexane, 1:4); 1H NMR (400 MHz, CDCl3) δ 7.79 (bs, 1 H), 7.20 (d, J = 8.3 Hz, 1 H), 6.57 (d, J = 8.3 Hz, 2 H), 6.18 (s, 1 H), 6.03 (dd, J = 17.3, 10.5 Hz, 1 H), 5.37 (t, J = 7.25 Hz, 1 H), 5.10 (d, J =17.3 Hz, 1 H), 5.08 (d, J = 10.5 Hz, 1 H), 4.70 (s, 1 H), 3.55 (d, J = 7.25 Hz, 2 H), 1.84 (s, 3 H), 1.75 (s, 3 H), 1.42 (s, 6 H); 13C NMR (100 MHz, CDCl3) δ 148.7, 146.5, 144.5, 136.4, 134.0, 123.1, 122.3, 118.4, 112.0, 110.1, 108.1, 97.99, 38.3, 27.5, 25.9, 24.5, 18.1; IR (νmax) 3447, 1621, 1103, 913, 852 cm–1; HRMS (ESI/APCI+) calcd for C18H23NO (M + H) 270.1852, found 270.1851.
3-((Dimethylamino)methyl)-7-(3-methylbut-2-en-1-yl)-2-(2-methylbut-3-en-2-yl)-1H-indol-6-ol (15) from 13. To AcOH (500 µL) was added at rt, Me2NH (6 µL, 0.074 mmol, 1 eq, 40% aqueous solution) followed by H2CO (8 µL, 0.074 mmol, 1 eq, 37% aqueous solution) and stirred for 30 min. To this was added at rt a solution of 13 (20 mg, 0.074 mmol, 1 eq) in AcOH (500 µL) and stirred for 4 h. The reaction was basified with 2M NaOH to a pH of 10–12 and extracted with Et2O (3 x 10 mL). The organic layers were combined and dried over anhydrous MgSO4, filtered and concentrated under reduced pressure, to give 15 (4 mg, 20%) as a yellow oil. 1H NMR (400 MHz, CDCl3) δ 7.89 (bs, 1 H), 7.35 (d, J = 8.4 Hz, 1 H), 6.60 (d, J = 8.4 Hz, 1 H), 6.16 (dd, J = 17.5, 10.6 Hz, 1 H), 5.35 (t, J = 7.3, 1 H), 5.16 (d, J = 17.3, 1 H), 5.12 (d, J = 10.6, 1 H), 3.59 (bs, 1 H), 3.53 (d, J = 7.3 Hz, 2 H), 2.24 (s, 6 H), 1.84 (s, 3 H), 1.75 (s, 3 H), 1.51 (s, 6 H); 13C NMR (100 MHz, CDCl3) δ 148.8, 146.5, 134.4, 133.8, 122.6, 117.4, 111.8, 109.9, 107.8, 54.0, 45.3, 39.5, 30.5, 29.8, 27.3, 25.9, 25.7, 24.4, 18.1; IR (νmax) 3457, 1623, 1241, 1011, 915, 842 cm–1; HRMS (ESI/APCI+), calcd for C19 H24NO (M – N(CH3)2) 282.1852, found 282.1860.
N,N-Dimethyl-1-(2-(2-methylbut-3-en-2-yl)-6-((2-methylbut-3-yn-2-yl)oxy)-1H-indol-3-yl)methanamine (16). To AcOH (1.5 mL) was added at rt Me2NH (320 µL, 2.84 mmol, 1.05 eq, 40% aqueous solution) followed by H2CO (230 µL, 2.84 mmol, 1.05 eq, 37% aqueous solution) and stirred for 30 min. To this was added at rt a solution of 14 (724 mg, 2.70 mmol, 1 eq) in AcOH (1.5 mL) and stirred for 4 h. The reaction was basified with 2M NaOH to a pH of 10–12 and washed with Et2O (3 x 10 mL). The organic layers were combined and dried over anhydrous MgSO4, and concentrated under reduced pressure, to give 16 (835 mg, 95%) as a yellow solid. 1H NMR (400 MHz, CDCl3) δ 7.78 (bs, 1 H), 7.52 (d, J = 8.5 Hz, 1 H), 7.15 (d, J = 1.9 Hz, 1 H), 6.90 (dd, J = 8.5, 1.9 Hz, 1 H), 6.16 (dd, J = 17.5, 10.5, 1 H), 5.17 (d, J = 17.5, 1 H), 5.13 (d, J = 10.5, 1 H), 3.54 (s, 2 H), 2.50 (s, 1 H), 2.20 (s, 6 H), 1.60 (s, 6 H), 1.53 (s, 6 H); 13C NMR (100 MHz, CDCl3) δ 146.4, 140.8, 133.9, 127.0, 119.0, 115.9, 112.0, 103.9, 73.3, 73.0, 54.2, 45.5, 42.1, 39.4, 29.8, 27.3, 25.8; IR (νmax) 3301, 1622, 1463, 1231, 1134, 1042, 1012, 968, 914 cm–1; HRMS (ESI/APCI+), calcd for C19H22NO (M – N(CH3)2) 280.1696, found 280.1695.
3-((Dimethylamino)methyl)-7-(3-methylbut-2-en-1-yl)-2-(2-methylbut-3-en-2-yl)-1H-indol-6-ol (15). To 16 (100 mg, 0.30 mmol, 1 eq) in MeOH (3 mL) was added at rt Lindlar’s catalyst (10 mg, 10 wt %) followed by purging with a balloon of hydrogen gas. The reaction was vigorously stirred for 30 min. The reaction was monitored by NMR for loss of the alkyne proton. The reaction was then filtered through a plug of Celite and concentrated under reduced pressure. The resultant yellow oil was then taken up in toluene (3 mL) and subjected to microwave conditions of 150 ºC for 30 min. The reaction was then concentrated under reduced pressure to give crude 15 (75 mg, 75%) as a yellow oil. 1H NMR (400 MHz, CDCl3) δ 7.89 (bs, 1 H), 7.35 (d, J = 8.4 Hz, 1 H), 6.60 (d, J = 8.4 Hz, 1 H), 6.16 (dd, J = 17.5, 10.6 Hz, 1 H), 5.35 (t, J = 7.3, 1 H), 5.16 (d, J = 17.3, 1 H), 5.12 (d, J = 10.6, 1 H), 3.59 (bs, 1 H), 3.53 (d, J = 7.3 Hz, 2 H), 2.24 (s, 6 H), 1.84 (s, 3 H), 1.75 (s, 3 H), 1.51 (s, 6 H); 13C NMR (100 MHz, CDCl3) δ 148.8, 146.5, 134.4, 133.8, 122.6, 117.4, 111.8, 109.9, 107.8, 54.0, 45.3, 39.5, 30.5, 29.8, 27.3, 25.9, 25.7, 24.4, 18.1; IR (νmax) 3457, 1623, 1241, 1011, 915, 842 cm–1; HRMS (ESI/APCI+), calcd for C19 H24NO (M – N(CH3)2) 282.1852, found 282.1860.
Ethyl 2-amino-3-(6-hydroxy-7-(3-methylbut-2-en-1-yl)-2-(2-methylbut-3-en-2-yl)-1H-indol-3-yl)propanoate (17). To a solution of 15 (61 mg, 0.18 mmol, 1 eq) and N-(diphenylmethylene)glycine ethyl ester (52 mg, 0.19 mmol, 1.05 eq) in acetonitrile (2 mL) was added at rt Bu3P (10 µL, 0.074 mmol, 0.4 eq). The reaction was heated under reflux for 24 h. The reaction was cooled to rt and concentrate under reduced pressure. The residue was taken up in THF (3 mL) and 1 M HCl (2 mL) was added and the reaction was stirred at rt for 3 h. The solution was basified with sat. aq. NaHCO3 and washed with AcOEt (3 x 10 mL). The organics were combined and dried over anhydrous Na2SO4, filtered and concentrated under reduced pressure. The yellow residue was purified by flash chromatography (MeOH/CH2Cl2, 0:1–1:20) to give 17 (7 mg, 10%) as a yellow oil. Rf = 0.36 (MeOH/CH2Cl2, 1:20); 1H NMR (400 MHz, CDCl3) δ 7.86 (bs, 1 H), 7.13 (d, J = 8.4 Hz, 1 H), 6.49 (d, J = 8.4 Hz, 1 H), 6.11 (dd, J = 17.5, 10.5 Hz, 1 H), 5.33 (t, J = 7.3 Hz, 1 H), 5.14 (dd, J = 17.4, 11.8 Hz, 2 H), 4.16-4.07 (m, 2 H), 3.85-3.81 (m, 1 H), 3.53 (d, J = 7.3 Hz, 2 H), 3.29 (dd, J = 14.4, 4.7 Hz, 2 H), 3.00 (dd, J = 14.4, 4.5 Hz, 2 H), 1.84 (s, 3 H), 1.74 (s, 3 H), 1.49 (s, 6 H), 1.18 (t, J = 7.1 Hz, 3 H); 13C NMR (100 MHz, CDCl3) δ 175.4, 149.2, 146.4, 139.0, 134.9, 133.4, 124.2, 122.9, 116.8, 111.9, 110.1, 108.3, 106.7, 61.1, 55.8, 39.3, 31.2, 28.0, 27.9, 25.9, 24.3, 18.1, 14.2; IR (νmax) 3449, 1730, 1621, 1444, 1100, 917, 799 cm–1; HRMS (ESI/APCI+) calcd for C23H32N2O3 (M + H) 385.2486, found 385.2483.
Ethyl 2-amino-3-(2-(2-methylbut-3-en-2-yl)-6-((2-methylbut-3-yn-2-yl)oxy)-1H-indol-3-yl)propane- ate (18). To a solution of 16 (180 mg, 0.52 mmol, 1 eq) and N-(diphenylmethylene)glycine ethyl ester (155 mg, 0.57 mmol, 1.1 eq) in acetonitrile (5 mL) was added at rt Bu3P (29 µL, 0.21 mmol, 0.4 eq). The reaction was taken to 70ºC and stirred for 24 h. Cool to rt and concentrate under reduced pressure. The residue was then taken up in THF (5 mL) at rt was added 1M HCl (1 mL) and stirred at rt for 1 h. The solution was basified with 2M NaOH and washed with Et2O (3 x 10 mL). The combined organic layers were washed with Brine (15 mL), dried of over anhydrous Na2SO4, filtered and concentrated under reduced pressure to give crude 18 as a yellow oil. The residue was purified by flash chromatography (MeOH/CH2Cl2, 0:100–1:20) to give 18 (160 mg, 80%) as a light yellow foam. Rf = 0.36 (MeOH/CH2Cl2, 1:20); 1H NMR (300 MHz, CDCl3) δ 8.41 (bs, 1 H), 7.35 (d, J = 8.5 Hz, 1 H), 7.16 (s, 1 H), 6.89 (d, J = 8.5 Hz, 1 H), 6.12 (dd, J = 17.5, 10.5 Hz, 1 H), 5.12 (d, J = 17.5 Hz, 1 H), 5.08 (d, J = 10.5 Hz, 1 H), 4.08-3.96 (m, 2 H), 3.79 (q, J = 5.3 Hz, 1 H), 3.25 (dd, J = 14.4, 5.3 Hz, 1 H), 3.02 (dd, J = 14.3, 9.3 Hz, 1 H), 2.49 (s, 1 H), 1.56 (s, 6 H), 1.48 (s, 6 H), 1.07 (t, J = 7.1 Hz, 3 H); 13C NMR (75 MHz, CDCl3) δ 175.8, 151.1, 146.2, 140.2, 134.4, 118.5, 116.1, 112.2, 104.3, 87.0, 73.5, 73.1, 61.0, 56.1, 39.3, 31.5, 29.8, 28.0, 24.6, 24.4, 23.9; IR (νmax) 3267, 1733, 1622, 1464, 1136, 969 cm–1; HRMS (ESI/APCI+) calcd for C23H31N2O3 (M + H) 383.2329, found 383.2328.
Ethyl (2S)-(9H-Fluoren-9-yl)methyl-2-((1-(2-(2-methylbut-3-en-2-yl)-6-((2-methylbut-3-yn-2-yl)oxy)- 1H-indol-3-yl)butan-2-yl)carbamoyl)pyrrolidine-1-carboxylate (19). To a solution of 18 (50 mg, 0.130 mmol, 1 eq) and N-(9-fluorenylmethoxycarbonyl)-L-proline (46 mg, 0.137 mmol, 1.05 eq) in acetonitrile (1.5 mL) at 0 ºC was added HATU (62 mg, 0.163 mmol, 1.25 eq) followed by i-Pr2NEt (47 µL, 0.287 mmol, 2.2 eq). The reaction was allowed to warm to rt and was stirred for 12 h. The solution was concentrated under reduced pressure and the residue was purified by flash chromatography (AcOEt/hexane, 1:5–9:20) to give dipeptide 19 (77 mg, 85%) as a white foam. Rf = 0.35 (AcOEt/hexane, 1:1); 1H NMR (300 MHz, CDCl3, mixture of rotomers, diastereomers) δ 7.79-7.77 (comp, 3 H), 7.61-7.58 (comp, 2 H), 7.37-7.26 (comp, 5 H), 7.14 (bs, 1), 6.93-6.89 (comp, 1 H), 6.17-6.07 (comp, 1 H), 5.22-5.14 (comp, 2 H), 4.79-4.70 (comp, 1 H), 4.45-4.33 (comp, 2 H), 4.29-4.15 (comp, 3 H), 4.00-3.77 (comp, 3 H), 3.51-3.33 (comp, 2 H), 3.29-3.08 (comp, 3 H), 2.48 (bs, 1 H) 1.99-1.93 (comp, 2 H), 1.92-1.80 (comp, 2 H), 1.59 (bs, 6 H), 1.54 (bs, 6H), 1.01-0.89 (comp, 3 H).
13C NMR (100 MHz, CDCl3, mixture of diastereomers) δ 172.6, 151.1, 151.0, 145.9, 144.2, 144.1, 141.4, 134.3, 127.9, 127.3, 125.4, 120.1, 112.4, 104.5, 87.1, 87.0, 73.6, 73.1, 67.8, 61.4, 60.6, 53.8, 47.3, 39.3, 29.8, 27.7, 21.2, 14.4, 13.9, 13.8; IR (νmax) 3346, 1684, 1126, 968, 739 cm–1; HRMS (ESI/APCI+) calcd for C43H47N3NaO6 (M + Na) 724.3357, found 724.3355.
(3S,8aS)-3-((7,7-Dimethyl-2-(2-methylbut-3-en-2-yl)-1,7-dihydropyrano[2,3-g]indol-3-yl)methyl)hexahydropyrrolo[1,2-a]pyrazine-1,4-dione (9) and (3R,8aS)-3-((7,7-Dimethyl-2-(2-methylbut-3- en-2-yl)-1,7-dihydropyrano[2,3-g]indol-3-yl)methyl)hexahydropyrrolo[1,2-a]pyrazine-1,4-dione (20). To 19 (71 mg, 0.10 mmol, 1 eq) in acetonitrile (1.5 mL) at rt wasadded Et2NH (700 µL) and the reaction was stirred for 1 h at rt. The reaction was concentrated under reduced pressure to give an orange/brown residue. The residue was dissolved in toluene (3 mL) and heated under reflux for 18 h. The solution was then concentrated under reduced pressure and the residue was purified by flash chromatography (MeOH/CH2Cl2, 1:20) to give 9 (15 mg, 36%) & 20 (19 mg, 44%). (9) Rf = 0.41 (MeOH/CH2Cl2, 1:20); 1H NMR (300 MHz, CDCl3) δ 7.91 (s, 1 H), 7.21 (d, J = 8.5 Hz, 1 H), 6.65 (d, J = 8.5 Hz, 1 H), 6.58 (d, J = 9.7 Hz, 1 H), 6.13 (dd, J = 17.5, 10.4 Hz, 1 H), 5.70 (bs, 1 H), 5.67 (d, J = 9.7 Hz, 1 H), 5.21-5.10 (m, 2 H), 4.44-4.33 (m, 1 H), 4.12-4.00 (m, 1 H), 3.75-3.51 (m, 3 H), 3.12 (dd, J = 15.3, 11.7 Hz, 1 H), 2.41-2.27 (m, 1 H), 2.21-1.81 (m, 3 H), 1.54 (s, 6 H), 1.46 (s, 6 H); 13C NMR (75.5 MHz, CDCl3) δ 169.5, 166.0, 149.1, 146.0, 140.1, 131.0, 130.2, 123.9, 118.2, 117.0, 112.8, 111.1, 105.2, 105.1, 76.0, 59.4, 55.1, 45.6, 39.2, 28.6, 28.1, 27.6, 27.5, 26.1, 22.8; HRMS (FAB) calcd for C26H32N3O3 [M + H] 434.2444, found 434.2422. (20) Rf = 0.29 (MeOH/CH2Cl2, 1:20); 1H NMR (300 MHz, CDCl3) δ 7.87 (bs, 1 H), 7.25 (d, J = 8.4 Hz, 1 H), 6.64 (d, J = 8.4 Hz, 1 H), 6.58 (d, J = 9.7 Hz, 1 H), 6.13 (dd, J = 17.5, 10.6 Hz, 1 H), 5.84 (d, J = 3.7 Hz, 1 H), 5.66 (d, J = 9.9, 1 H), 5.19 (d, J = 11.7 Hz, 1 H), 5.15 (d, J = 4.4 Hz, 1 H), 4.31-4.22 (m, 1 H), 3.76-3.55 (m, 2 H), 3.52-3.35 (m, 2 H), 3.26 (dd, J = 14.6, 9.0 Hz, 1 H), 2.37-2.21 (m, 1 H), 2.05-1.62 (m, 4 H), 1.52 (s, 6 H), 1.45 (s, 6 H); 13C NMR (75.5 MHz, CDCl3) δ 168.8, 166.0, 148.9, 146.4, 140.2, 130.9, 130.0, 123.7, 118.9, 117.0, 112.0, 110.8, 105.7, 104.8, 75.9, 58.7, 58.4, 45.7, 39.3, 30.0, 29.2, 28.2, 28.0, 27.5, 22.1; HRMS (FAB) calcd for C26H32N3O3 [M + H] 434.2444, found 434.2422.
(3S,8aS)-3-((6-Hydroxy-7-(3-methylbut-2-en-1-yl)-2-(2-methylbut-3-en-2-yl)-1H-indol-3-yl)methyl)hexahydropyrrolo[1,2-a]pyrazine-1,4-dione (8) (Notoamide S). To 19 (286 mg, 0.41 mmol, 1 eq) in MeOH (4 mL) was added at rt Lindlar’s catalyst (28 mg, 10 wt %) followed by purging with a balloon of hydrogen. The reaction was vigorously stirred for 30 min. The reaction was monitored by NMR for loss of the alkyne proton. The reaction was then filtered through a plug of Celite and concentrated under reduced pressure to give the alkene as yellow foam which was directly taken on to the next step without further purification. Et2NH (2 mL) was added to a solution of the crude alkene in acetonitrile (3 mL) and the reaction was stirred for 1 h at rt. The reaction was concentrated under reduced pressure to give a yellow oil. The residue was dissolved in toluene (5 mL) and heated under reflux for 18 h. The reaction was then concentrated under reduced pressure and purified by flash chromatography (MeOH/CH2Cl2, 1:20) to give 8 (51 mg, 29%), as a yellow oil. Rf = 0.67 (MeOH/CH2Cl2, 1:10); 1H NMR (300 MHz, CDCl3) δ 8.01 (bs, 1 H), 7.15 (d, J = 8.5 Hz, 1 H), 6.65 (d, J = 8.5 Hz, 1 H), 6.15 (dd, J = 17.5, 10.3 Hz, 1 H), 5.75 (bs, 1 H), 5.38 (t, J = 7.2 Hz, 1 H), 5.18 (d, J = 5.35 Hz, 1 H), 5.13 (s, 1 H), 4.41 (dd, J = 10.7, 2.5 Hz, 1 H), 4.08 (t, J = 7.3 Hz, 1 H), 3.71-3.61 (m, 2 H), 3.57 (d, J = 7.2 Hz, 2 H), 3.15 (dd, J = 15.3, 11.6 Hz, 1 H), 2.39-2.31 (m, 1 H), 2.12-2.00 (m, 2 H), 1.88 (s, 3 H), 1.79 (s, 3 H), 1.50 (s, 6 H); 13C NMR (100 MHz, CDCl3) δ 169.4, 166.0, 149.4, 145.9, 139.9, 134.9, 133.9, 123.6, 122.5, 116.2, 112.6, 110.5, 104.5, 59.4, 55.1, 45.5, 39.1, 29.8, 28.5, 28.0, 27.9, 25.9, 24.3, 22.8, 18.1; IR (νmax) 3362, 1665, 1438, 919, 800 cm–1; HRMS (ESI/APCI+) calcd for C26H32DN3O3 [M + Na] 459.2477, found 459.2475. (21) (35 mg, 20%) Rf = 0.53 (MeOH/CH2Cl2, 1:10); 1H NMR (400 MHz, CDCl3) δ 7.94 (bs, 1 H), 7.15 (d, J = 8.4 Hz, 1 H), 6.63 (d, J = 8.4 Hz, 1 H), 6.11 (dd, J = 17.4, 10.3 Hz, 1 H), 5.83 (bs, 1 H), 5.76 (bs, 1 H), 5.32 (t, J = 7.4 Hz, 1 H), 5.15 (dd, J = 17.4, 10.3 Hz, 2 H), 4.24-4.21 (m, 2 H), 3.78-3.74 (m, 1 H), 3.68-3.61 (m, 1 H), 3.53 (d, J = 7.4 Hz, 2 H), 3.48-3.37 (m, 3 H), 3.22 (dd, J = 14.4, 10 Hz, 1 H), 2.32-2.27 (m, 1 H), 1.99-1.86 (m, 2 H), 1.84 (s, 3 H), 1.74 (s, 3 H), 1.45 (s, 6 H); 13C NMR (100 MHz, CDCl3) δ 168.7, 166.0, 149.4, 146.3, 139.9, 135.0, 133.6, 123.3, 122.7, 116.7, 111.8, 110.3, 108.3, 105.0, 58.9, 58.4, 45.7, 39.2, 30.1, 29.2, 28.0, 27.8, 25.9, 24.3, 22.1, 18.1; IR (νmax) 3336, 1655, 1448, 918, 802 cm–1; HRMS (ESI/APCI+) calcd for C26H33N3NaO3 [M + Na] 458.2414, found 458,2413.
ACKNOWLEDGEMENTS
This paper is dedicated to Professor Albert Eschenmoser on the occasion of his 85th birthday. We wish to acknowledge the National Institutes of Health for financial support (Grant CA CA070375 (R.M.W. and D.H.S), and the H. W. Vahlteich Professorship (D.H.S). T.S. acknowledges financial support from a Grant-in-aid for Scientific Research (No. 19310140) from the Ministry of Education, Culture, Sports, Science and Technology of Japan and also by a grant from the Naito Foundation.
References
1. M. Yamazaki, E. Okuyama, M. Kobayashi, and H. Inoue, Tetrahedron Lett., 1981, 22, 135; CrossRef J. G. Ondeyka, R. T. Goegelman, J. M. Schaeffer, L. Kelemen, and L. Zitano, J. Antibiot. (Tokyo), 1990, 43, 1375; J. M. Liesch and C. F. Wichmann, J. Antibiot. (Tokyo), 1990, 43, 1380; R. M. Banks, S. E. Blanchflower, J. R. Everett, B. R. Manger, and C. Reading, J. Antibiot. (Tokyo), 1997, 50, 840.
2. A. J. Birch and J. J. Wright, J. Chem. Soc., Chem. Commun., 1969, 644; A. J. Birch and J. J. Wright, Tetrahedron, 1970, 26, 2329; CrossRef A. J. Birch and R. A. Russell, Tetrahedron, 1972, 28, 2999. CrossRef
3. (a) J. Qian-Cutrone, S. Huang, Y. Z. Shu, D. Vyas, C. Fairchild, A. Menendez, K. Krampitz, R. Dalterio, S. E. Klohr, and Q. Gao, J. Am. Chem. Soc., 2002, 124, 14556; CrossRef (b) J. K. Quin-Cutrone, Y. Z. Shu, and L. P. Chang, U.S. Patent 6,291,461. 2001.
4. H. Kato, T. Yoshida, T. Tokue, Y. Nojiri, H. Hirota, T. Ohta, R. M. Williams, and S. Tsukamoto, Angew. Chem. Int. Ed., 2007, 46, 2254. CrossRef
5. T. J. Greshock, A. W. Grubbs, S. Tsukamoto, and R. M. Williams, Angew. Chem. Int. Ed., 2007, 46, 2262; CrossRef T. J. Greshock and R. M. Williams, Org. Lett., 2007, 9, 4255. CrossRef
6. S. T. Deyrup, D. C. Swenson, J. B. Gloer, and T. W. Donald, J. Nat. Prod., 2006, 69, 608; CrossRef S. H. Shim, D. C. Swenson, J. B. Gloer, P. F. Dowd, and D. T. Wicklow, Org. Lett., 2006, 8, 1225; CrossRef S. V. Mudur, J. B. Gloer, and D. T. Wicklow, J. Antibiot., 2006, 59, 500. CrossRef
7. A. E. A. Porter and P. G. Sammes, J. Chem. Soc., Chem. Commun., 1970, 1103. CrossRef
8. J. Baldas, A. J. Birch, and R. A. Russell, J. Chem. Soc., Perkin Trans. 1, 1974, 50. CrossRef
9. R. M. Williams, J. F. Sanz-Cervera, F. Sancenon, J. A. Marco, and K. Halligan, J. Am. Chem. Soc., 1998, 120, 1090; CrossRef R. M. Williams, J. F. Sanz-Cervera, F. Sancenon, J. A. Marco, and K. M. Halligan, Bioorg. Med. Chem., 1998, 6, 1233; CrossRef R. M. Williams, E. M. Stocking, and J. F. Sanz-Cevra, Topics Curr. Chem., 2000, 209, 97; CrossRef J. F. Sanz-Cervera, R. M. Williams, J. A. Marco, J. M. Lopez-Sanchez, F. Gonzalez, M. E. Martinez, and F. Sancenon, Tetrahedron, 2000, 56, 6345; CrossRef R. M. Williams, Chem. Pharm. Bull., 2002, 50, 711; CrossRef R. M. Williams and R. J. Cox, Acc. Chem. Res., 2003, 36, 127; CrossRef E. M. Stocking and R. M. Williams, Angew. Chem. Int. Ed., 2003, 42, 3078; CrossRef L. A. Adams, M. W. N. Valente, and R. M. Williams, Tetrahedron, 2006, 62, 5195; CrossRef K. A. Miller and R. M. Williams, Chem. Soc. Rev., 2009, 38, 3160. CrossRef
10. T. J. Greshock, A. W. Grubbs, P. Jiao, D. T. Wicklow, J. B. Gloer, and R. M. Williams, Angew. Chem. Int. Ed., 2008, 47, 3573; CrossRef S. Tsukamoto, T. Kawabata, H. Kato, T. J. Greshock, H. Hirota, T. Ohta, and R. M. Williams, Org. Lett., 2009, 11, 1297. CrossRef
11. Y. Ding, J. R. de Wet, J. Cavalcoli, S. Li, T. J. Greshock, K. A. Miller, J. M. Finefield, J. D. Sunderhaus, T. J. McAfoos, S. Tsukamoto, R. M. Williams, and D. H. Sherman (unpublished results).
12. S. Tsukamoto, H. Kato, T. J. Greshock, H. Hirota, T. Ohta, and R. M. Williams, J. Am. Chem. Soc., 2009, 131, 3834. CrossRef
13. R. J. Cox and R. M. Williams, Tetrahedron Lett., 2002, 43, 2149. CrossRef
14. M. Somei, Y. Karasawa, and C. Kaneko, Heterocycles, 1981, 16, 941. CrossRef
15. T. Kametani, N. Kanaya, and M. Ihara, J. Chem. Soc., Perkin Trans. 1, 1981, 959. CrossRef
16. A. W. Grubbs, G. D. Artman, 3rd, S. Tsukamoto, and R. M. Williams, Angew. Chem. Int. Ed., 2007, 46, 2257. CrossRef