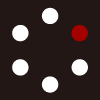
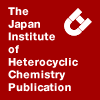
HETEROCYCLES
An International Journal for Reviews and Communications in Heterocyclic ChemistryWeb Edition ISSN: 1881-0942
Published online by The Japan Institute of Heterocyclic Chemistry
e-Journal
Full Text HTML
Received, 26th August, 2010, Accepted, 18th October, 2010, Published online, 19th October, 2010.
DOI: 10.3987/COM-10-S(E)115
■ Second Generation Palladium-Catalyzed Cycloalkenylation in Iridoid Lactone Synthesis: Total Syntheses of (±)-Onikulactone and (±)-Mitsugashiwalactone
Megumi Saeki and Masahiro Toyota*
Department of Chemistry, Graduate School of Science, University of Osaka Prefecture, Sakai, Osaka 593-8531, Japan
Abstract
Type-II iridoid lactones, onikulactone (1) and mitsugashiwalactone (2), were synthesized by employing the second generation palladium-catalyzed cycloalkenylation reaction as the key step.We recently developed a palladium-catalyzed cycloalkenylation of olefinic keto and lactone esters (second generation palladium-catalyzed cycloalkenylation)1 and successfully applied this protocol to the total syntheses of (±)-cis- and trans-195A, a member of the decahydroquinoline class of dendrobatid alkaloids.2 In an effort to further expand the utility of this catalytic cyclization, we focused on the concise construction of iridoid lactones through strategic application of this reaction. Here, we describe the total syntheses of (±)-onikulactone (1) and (±)-mitsugashiwalactone (2).
Onikulactone (1) and mitsugashiwalactone (2) were isolated by Sakan and coworkers as the biological active principles of Boschniakia rossica Hult and Menyanthes trifoliate L., which have attractive physiological actions on Felidae.3 Although most natural iridoids include a terpenoid ten-carbon core, onikulactone (1) and mitsugashiwalactone (2) type-II iridoid lactones are notable exceptions.4
A retrosynthetic analysis of (±)-onikulactone (1) and (±)-mitsugashiwalactone (2) is shown in Scheme 1. We anticipated that both natural products (1 and 2) could be synthesized by manipulating the functional groups of the bicyclic lactones 3, which could be prepared from the olefinic lactone ester 4 by the second generation palladium-catalyzed cycloalkenylation developed by us.1
Conjugate addition of homoallyl magnesium bromide to commercially available 5,6-dihydro-2H-pyran-2-one (5) gave rise to 6, in 90% yield, which was transformed to the lactone ester 41a as a 1:3 mixture of diastereoisomers. Surprisingly, application of a tandem conjugate addition–trapping procedure to 5 using methyl cyanoformate provided 6 (< 70%) instead of 4. The second generation palladium-catalyzed cycloalkenylation of 4 was conducted for 24 h in the presence of 10 mol % Pd(OAc)2 to afford the desired cyclization products 71a and 85 in 70% total yield together with 9 (4%) (Scheme 2).
The Krapcho reaction of the isomeric mixture 3 was performed by application of lithium chloride to give the unsaturated bicyclic lactones 10 and 115 in a 5:2 mixture in 98% yield. The ratio between 10 and 11 appeared to reflect the ratio of the cyclization products 7 and 8. This was confirmed by individual conversion of 7 and 8 to 10 and 11, respectively. Finally, hydrogenation of a mixture of unsaturated lactones 10 and 11 furnished (±)-onikulactone (1) and (±)-mitsugashiwalactone (2) in 40% and 37% yield, respectively. Interestingly, each hydrogenation of 10 and 11 led to (±)-1 and (±)-2 as a 1:1 mixture. (±)-Mitsugashiwalactone (2) could be generated through syn-addition of hydrogen followed by epimerization (Scheme 3). Each stereoisomer was easily isolated by flash column chromatography, and the NMR spectral characterizations of the synthetic (±)-1 and (±)-2 were identical to the corresponding previously reported spectra.6
EXPEIMENTAL
IR spectra were measured on a SHIMADZU FT-IR 8300 spectrophotometer. 1H NMR spectra were recorded on Varian 400 MR (400 MHz) or JEOL JX-500 (500 MHz) spectrometers with tetramethylsilane (δ 0) or CHCl3 (δ 7.26) as an internal standard. Mass spectra were recorded on JEOL JMS-AX 700 spectrometers.
Onikulactone (1) and Mitsugashiwalactone (2)
To a stirred solution of a 5:2 mixture of 10 and 11 (3.5 mg, 2.30×10-5 mol) in MeOH (1.0 mL) was added 10% Pd-C (0.5 mg) and the resulting mixture was stirred at room temperature for 1.5 h under an atmosphere of hydrogen. The mixture was filtered through Celite and the filtrate was concentrated in vacuo, and the residue was purified by flash column chromatography with hexane-EtOAc (3:1 v/v) as an eluent, giving onikulactone (1) (1.4 mg, 40%) and mitsugashiwalactone (2) (1.3 mg, 37%) as a colorless oil respectively.
Data for onikulactone (1): 1H NMR (400 MHz, CDCl3) δ 4.35 (1H, ddd, J = 11.5, 6.4 and 3.7 Hz), 4.22 (1H, ddd, J = 11.5, 8.7 and 3.2 Hz), 3.02 (1H, dd, J = 10.1 and 8.7 Hz), 2.63-2.58 (1H, m), 2.50-2.42 (1H, m), 2.01-1.94 (2H, m), 1.79-1.72 (1H, m), 1.65-1.46 (3H, m), 1.10 (3H, d, J = 6.8 Hz); LRMS m/z 154 (M+), 99; HRMS calcd for C9H14O2 154.0994, found 154.0992.
Data for mitsugashiwalactone (2); IR (neat) 1733, 1257, 1074 cm-1; 1H NMR (400 MHz, CDCl3) δ 4.32 (1H, ddd, J = 11.2, 6.8 and 2.8 Hz), 4.19 (1H, ddd, J = 11.2, 8.4 and 2.8 Hz), 2.61-2.51 (1H, m), 2.37 (1H, dd, J = 10.4 and 9.2 Hz), 2.28-2.17 (1H, m), 2.06-1.98 (2H, m), 1.92 (1H, dddd, J = 12.0, 6.0, 6.0 and 1.6 Hz), 1.56-1.48 (1H, m), 1.37-1.14 (2H, m), 1.20 (3H, d, J = 6.8 Hz); LRMS m/z 154 (M+), 99; HRMS calcd for C9H14O2 154.0994, found 154.0992.
References
1. (a) Catalytic conditions: A. Hibi and M. Toyota, Tetrahedron Lett., 2009, 50, 4888; CrossRef (b) Stoichiometric conditions: A. Hibi, K. Takeda, and M. Toyota, Heterocycles, 2009, 77, 173. CrossRef
2. M. Saeki and M. Toyota, Tetrahedron Lett., 2010, 51, 4620. CrossRef
3. (a) Isolations: T. Sakan, F. Murai, S. Isoe, S. B. Hyeon, and Y. Hayashi, J. Chem. Soc. Jpn., 1969, 90, 507; (b) Recent total syntheses: H. Nagata and K. Ogasawara, Tetrahedron Lett., 1999, 40, 6617; CrossRef (c) D. Bouyssi, N. Monteiro, and G. Balme, Tetrahedron Lett., 1999, 40, 1297; CrossRef (d) K.-W, Liang, M. Chandrasekharam, C.-L. Li, and R.-S, Liu, J. Org. Chem., 1998, 63, 7289. CrossRef
4. Reviews: (a) D. H. Grayson, Nat. Prod. Rep., 2000, 17, 385; CrossRef (b) A. Nangia, G. Prasuna, and P. B. Rao, Tetrahedron, 1997, 53, 14507. CrossRef
5. Satisfactory analytical data were obtained for all new compounds. Compound 8: IR (neat) 1745 and 1730 cm-1. 1H NMR (400 MHz, CDCl3) δ 5.74 (1H, br s), 4.35 (1H, ddd, J = 11.2, 7.6 and 3.2 Hz), 4.28 (1H, dddd, J = 11.2, 7.2, 2.8, and 0.4 Hz), 3.78 (3H, s), 3.08-3.01 (1H, m), 2.79-2.70 (1H, m), 2.22-2.06 (2H, m), 1.87 (3H, br s), and 1.77-1.69 (1H, m). 13C NMR (100 MHz, CDCl3) δ 171.1, 168.3, 137.0, 131.4, 67.7, 66.9, 53.1, 41.1, 38.1, 29.4, and 14.8. LRMS m/z 210 (M+). HRMS calcd for C11H14O4 (M+) 210.0892, found 210.0890. Compound 11: IR (neat) 1713 cm-1 1H NMR (400 MHz, CDCl3) δ 5.55 (1H, br s), 4.35-4.20 (2H, m), 3.80-3.50 (1H, m), 3.00-2.84 (1H, m), 2.74 (1H, dddd, J = 16.4, 9.2, 4.8, and 2.4 Hz), 2.14-1.98 (2H, m), 1.81 (3H, br s), and 1.72-1.61 (1H, m). LRMS m/z 152 (M+). HRMS calcd for C9H12O2 152.0837, found 152.0836.
6. H. Ohta, T. Kobori, and T. Fujiwara, J. Org. Chem., 1977, 42, 1231. CrossRef