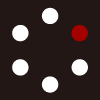
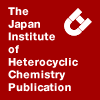
HETEROCYCLES
An International Journal for Reviews and Communications in Heterocyclic ChemistryWeb Edition ISSN: 1881-0942
Published online by The Japan Institute of Heterocyclic Chemistry
e-Journal
Full Text HTML
Received, 16th March, 2010, Accepted, 14th May, 2010, Published online, 17th May, 2010.
DOI: 10.3987/COM-10-S(E)14
■ Simple and Efficient One Pot Synthetic Protocol to Construct Morpholin-2-ones
Gorakh S. Yellol, Debendra K. Mohapatra, Mukund K. Gurjar,* and Chung-Ming Sun*
Department of Applied Chemistry, National Chiao Tung University, Hsinchu 300-10, Taiwan, R.O.C.
Abstract
A new one pot synthetic method to construct 3-substituted morpholine-2-one derivatives is presented. Amino acids refluxed with 1,2-dibromoethane and potassium carbonate in acetonitrile followed by treatment with benzyl bromide in same pot furnished the 3-substituted N-benzyl-morpholine-2-ones in good yields. The simplicity of the reaction conditions and versatility of resulted scaffold to generate wide variety of molecules makes this method more attractive for synthetic organic chemists.INTRODUCTION
Heterocycles are among the most frequently encountered scaffolds in drugs and pharmaceutically relevant substances. Because of the drug-like character and considerable range of structural diversity, large collections or libraries of diverse heterocycles are routinely employed in high-throughput screening at early stages of drug discovery programs. Consequently, relatively small focused libraries of heterocycles are frequently generated for SAR studies. Among the different synthetic methodologies available for the production of compound libraries, one-pot multi-step synthesis constitutes a convenient alternative as it can avoid workup and purification of the intermediates between different reaction steps.1
The asymmetric synthesis of chiral heterocycles has been traditionally challenging as the process involves stereo-controlled formation of chiral centers. The synthesis of N-heterocycles in enantiomerically pure form is of great ongoing interest, particularly for the synthesis of bioactive natural products and medicinal applications.2 Although many strategies are available for the preparation of such compounds, there is still a need to develop new and practical methods for the synthesis of these molecules. The chiral amino acids act as privileged chiral tools to generate the relative chiral centers in stereo-controlled manner.3 The rigid geometry of 5- or 6-membered ring system embedded with amino acid allows unprecedented diastereoselectivity during establishment of new chiral center in a molecular structure. In particular, morpholin-2-one system has been fully tested as an effective template for asymmetric reactions.4 Harwood and Williams have shown that 5-phenyl-morpholinone system is an excellent template for making enantiomerically cyclic and acyclic amino acids.5
Moreover, morpholinones are known subunits in many natural products and biologically active pharmaceuticals.6 Varied biological activities have been attributed to morpholinone compounds. Compounds containing morpholin-2-one derivatives demonstrated for blocking T-type calcium channel, for prevention and treatment of myocardial disease, pain, heart attack, hypertension and epilepsy.7 Recently morpholin-2-one is identified as one of the major metabolite in an inhibition of human immunodeficiency virus-type 1 (HIV-1) protease.8 Morpholine ring embedded compounds like UR-9751 have been demonstrated high efficacy in animal models of systemic and vaginal candidosis9 (Figure 1). Some of the morpholine constituted natural products possess the anti-platelet activity.10 Tadalafil shows potential phosphordiesterase type 5 (PDE-5) inhibition activities.11 Aprepitant is a potent and orally active antiemetic drug well-known in the class of non-peptide antagonists to the tachykinin neurokinin NK1 receptor12 (Figure 1).
Additionally, the morpholine-2-ones have also been studied as chiral building blocks for many pharmaceutically interesting compounds. The morpholine-2-ones would investigated as a privileged synthons to generate various types of scaffolds (Figure 2). It would used for the stereoselective synthesis of quaternary proline analogues13 (A), opening of ring by ammonia to get a variety of amides14 (B), stereoselective reduction of carbonyl carbon to afford chiral alcoholes15 (C), stereocontrolled substitution at α-position to produce α-disubstituted amino acid derivatives16 (D) and also its dimerized product17 (E).
Based on these applications of morpholinone derivatives, we have sought to develop a protocol which enables us to synthesis a number of 3-substituted morpholin-2-one derivatives starting from commercially available α–amino acids. Herein, we present a concise approach to both enantiomeric forms of substituted morpholinones from amino acids which could be useful as chiral building blocks for pharmaceutically important compounds.
RESULTS AND DISCUSSION
Recently, Kim et al. have reported an efficient synthesis of morpholin-2-one derivatives using glycol-aldehyde dimer with various α–amino acids and isocyanides using Ugi’s multicomponent reaction.18 Kashima and his co-worker described the synthesis of morpholine-2-ones by treatment of amino-acids with 2-bromoethanol and also with its tosyl derivative in two-three steps reaction sequence.19 In further studies, they observed the formation of morpholinone in poor yields and sometimes with loss of chirality when chiral amino-acids heated with dichloroethanol or dibromoethane in DMF. The present paper concerns the extension of our earlier work20 and moreover the one pot synthesis of novel 3-substituted morpholine-2-ones in moderate to good yields.
In our first attempt, treatment of L-phenyl alanine 1 with 1,2-dibromoethane in refluxing acetonitrile in present of excess K2CO3 furnished desired product 2a with very low yield (Scheme 1). After few attempts with varying conditions, it was concluded that the low yield was due to less stability of these compounds at room temperature. Hence we decided to carry out the experiments with protected amino acids. In first attaimpt the reaction of N-Boc protected L-phenyl alanine 3 furnished the N-Boc-morpholinone 4 in low yield due to the stearic crowding to form morpholinone ring. Whereas N-benzyl protected L-phenyl alanine 5 demonstrated a substantial increase in the yield of N-benzyl-morpholinone 2a (Scheme 2).
In our effort to develop a one pot strategy, we treated the L-phenyl alanine with one equivalent of 1,2-dibromoethane and three equivalents of K2CO3 in acetonitrile. The reaction was monitored by thin layer chromatography. After complete conversion within 6 h, additional one equivalent of K2CO3 and one equivalent of benzyl bromide was added in the reaction mixture and the reaction was further refluxed for 4 h (Scheme 3). The workup procedure was very simple involving evaporation of the solvent under reduced pressure and the residue purified by column chromatography to afford the (S)-3,4-dibenzylmorpholin-2-one (2a) in 78% yield over two steps.
After successful development of the one-pot protocol for the synthesis of morpholin-2-ones, we investigate with other 1,2-dihaloethanes and observed that 1,2-dibromoethane gave superior results. We also tested various other bases and solvents and concluded that K2CO3 as a base and acetonitrile as a solvent at 80 °C temperature are the most favorable conditions for this one-pot conversion.
We have verified the versatility of this protocol by examining a number of commercially available α–amino acids (Table 1). This methodology was applied to variety of aromatic amino acids as well as aliphatic amino acids. Both the amino acids acts as a prominent substrates giving rise to moderate to good yields. This strategy is highly versatile and allows the synthesis of many types of novel molecules from readily available amino acids.
CONCLUSION
In conclusion, we have developed simple, scalable and synthetically useful one pot protocol for the preparation of 3-substituted-N-benzyl-morpholin-2-one derivatives. This method is expected to have high potential for the synthesis of other biologically active molecules containing the morpholin-2-one ring system.
EXPERIMENTAL
Solvents were distilled following standard procedures before use. Dimethylformamide and acetonitrile were dried over calcium hydride. TLC was performed on the pre-coated silica gel on aluminium plates Merck 60 F254. IR spectra were recorded on ATI Mattson RS-1 FT-IR spectrometer. 1H and 13C NMR spectra were recorded on Bruker AC-200 spectrometer with TMS as an internal standard. Mass spectra were obtained with a TSQ 70, Finningen MAT mass spectrometer. Optical rotations were determined on JASCO 370 digital polarimeter with sodium light source. Elemental analyses were carried out on a Carlo Erba CHNS-O analyzer.
(S)-3,4-Dibenzylmorpholin-2-one (2a). [α]D25 +4.6 (c 1.18, CHCl3); IR (neat) 3019, 1731, 1215, 757 cm−1; 1H NMR (200 MHz, CDCl3) δ 7.28 (m, 10H), 4.58 (s, 2H), 4.00 (dt, J = 9.7, 2.9 Hz, 1H), 3.76 (dt, J = 9.7, 2.9 Hz, 1H), 3.32 (d, J = 13.2 Hz, 1H), 3.22 (dd, J = 12.7, 4.8 Hz, 2H), 2.72 (dt, J = 13.2, 2.9 Hz, 1H), 2.46 (ddd, J = 12.7, 9.7, 2.9 Hz, 1H); 13C NMR (50 MHz, CDCl3) δ 169.9, 140.9, 136.6, 129.5, 128.8, 128.2, 127.6, 126.8, 126.2, 65.3, 63.9, 58.2, 45.7, 36.8; MSI m/z: 281 (M+). Anal. Calcd for C18H19NO2 (281.35): C, 76.84; H, 6.80; N, 4.98. Found: C, 77.04; H, 7.11; N, 4.25.
4-Benzylmorpholin-2-one (2b). IR (neat) 3028, 1729 cm−1; 1H NMR (200 MHz, CDCl3) δ 7.30 (m, 5 H), 4.19 (t, J = 6.3 Hz, 2H), 3.65 (s, 2H), 3.38 (s, 2H), 2.80 (t, J = 6.3 Hz, 2H); 13C NMR (50 MHz, CDCl3) δ 171.2, 136.8, 129.4, 128.8, 127.2, 68.3, 63.5, 58.7, 45.7. Anal. Calcd for C11H13NO2 (191.23): C, 69.09; H, 6.85; N, 7.32. Found: C, 70.08; H, 7.12, N, 7.19.
(S)-4-Benzyl-3-methylmorpholin-2-one (2c). [α]D25 +16.5 (c 0.98, CHCl3); IR (neat) 3024, 1735 cm−1; 1H NMR (200 MHz, CDCl3) δ 7.30 (m, 5 H), 4.27 (m, 2H), 3.95 (d, J = 13.2 Hz, 1H), 3.36 (q, J = 6.8 Hz, 1H), 3.30 (d, J = 13.2 Hz, 1H), 2.85 (dt, J = 12.7, 3.6 Hz, 1H), 2.48 (dt, J = 12.7, 3.6 Hz, 1H), 1.54 (d, J = 6.8 Hz, 3H); 13C NMR (50 MHz, CDCl3) δ 170.7, 137.2, 129.4, 128.7, 127.0, 68.0, 60.0, 58.3, 46.3, 16.3. Anal. Calcd for C12H15NO2 (205.26): C, 70.22; H, 7.37; N, 6.83. Found: C, 70.44; H, 7.43, N, 7.32.
(S)-4-Benzyl-3-isopropylmorpholin-2-one (2d). [α]D25 +24.5 (c 1.12, CHCl3); IR (neat) 3019, 2964, 1729 cm−1; 1H NMR (200 MHz, CDCl3) δ 7.30 (m, 5H), 4.29 (m, 2H), 3.94 (d, J = 13.6 Hz, 1H), 3.45 (d, J = 13.6 Hz, 1H), 3.24 (d, J = 3.9 Hz, 1H), 2.86 (dt, J = 12.7, 2.9 Hz, 1H), 2.52 (ddd, J = 12.7, 2.9 Hz, 1H), 2.20 (m, 1H), 1.17 (d, J = 6.8 Hz, 3H), 1.10 (d, J = 6.8 Hz, 3H); 13C NMR (50 MHz, CDCl3) δ 169.2, 137.9, 128.5, 128.2, 127.3, 70.3, 67.1, 60.3, 46.8, 32.3, 19.9, 18.3. Anal. Calcd for C14H19NO2 (233.28): C, 72.08; H, 8.20; N, 6.00. Found: C, 71.56; H, 8.23, N, 5.53.
(S)-4-Benzyl-3-isobutylmorpholin-2-one (2e). [α]D25 +34.2 (c 1.17, CHCl3); IR (neat) 2957, 1737, 1214, 755 cm−1; 1H NMR (200 MHz, CDCl3) δ 7.31 (m, 5 H), 4.35 (m, 2H), 3.95 (d, J = 13.2 Hz, 1H), 3.35 (d, J = 13.2 Hz, 1H), 3.30 (t, J = 6.8 Hz, 1H), 2.94 (dt, J = 12.7, 4.4 Hz, 1H), 2.50 (dt, J = 12.7, 4.4 Hz, 1H), 1.98 (m, 1H), 1.86 (t, J = 6.8 Hz, 2H), 0.95 (d, J = 6.8 Hz, 3H), 0.92 (d, J = 6.8 Hz, 3H); 13C NMR (50 MHz, CDCl3) δ 170.8, 137.3, 128.4, 127.5, 126.8, 67.0, 62.8, 58.6, 45.9, 39.6, 24.7, 23.0, 22.2; MSI m/z: 247 (M+).
(3S)-4-Benzyl-3-sec-butylmorpholin-2-one (2f). [α]D +28.7 (c 0.91, CHCl3); IR (neat) 3023, 1737, 758 cm−1; 1H NMR (200 MHz, CDCl3) δ 7.26 (m, 5H), 4.32 (m, 2H), 3.92 (d, J = 13.2 Hz, 1H), 3.79 (d, J = 13.2 Hz, 1H), 3.29 (d, J = 6.8 Hz, 1H), 2.54 (dt, J = 12.7, 4.8 Hz, 1H), 2.00 (m, 2H), 1.84 (m, 2H), 1.23 (d, J = 6.3 Hz, 3H), 0.98 (t, J = 6.3 Hz, 3H); 13C NMR (50 MHz, CDCl3) δ 168.8, 139.6, 128.3, 127.8, 126.7, 68.6, 64.8, 60.4, 47.9, 37.6, 24.7, 21.0, 18.2; MSI m/z: 247 (M+).
(S)-4-Benzyl-3-(2-(methylthio)ethyl)morpholin-2-one (2g). [α]D25 +6.4 (c 1.10, CHCl3); IR (neat) 3027, 1730, 1151, 698 cm−1; 1H NMR (200 MHz, CDCl3) δ 7.28 (m, 5H), 4.47 (m, 2H), 3.90 (d, J = 13.2 Hz, 1H), 3.72 (d, J = 13.2 Hz, 1H), 3.57 (m, 3H), 2.42 (m, 2H), 1.98 (q, J = 6.8 Hz, 2H), 1.97 (s, 3H); 13C NMR (50 MHz, CDCl3) δ 170.2, 137.0, 128.8, 128.7, 128.1, 67.5, 63.3, 58.6, 46.9, 30.0, 29.3, 15.0; MSI m/z: 265 (M+).
(S)-4-Benzyl-3-phenylmorpholin-2-one (2h). [α]D25 +9.0 (c 1.06, CHCl3); IR (neat): 3025, 1737, 1216, 699 cm−1; 1H NMR (200 MHz, CDCl3) δ 7.29 (m, 10H), 4.64 (s, 1H), 4.47 (m, 2H), 3.76 (s, 2H), 3.51 (t, J = 5.9 Hz, 3H); 13C NMR (50 MHz, CDCl3) δ 171.3, 139.2, 136.4, 128.7, 128.6, 128.2, 128.0, 127.3, 126.4, 65.7, 63.7, 54.2, 28.4; MSI m/z: 267 (M+).
(S)-4-Benzyl-3-(4-(benzyloxy)benzyl)morpholin-2-one (2i). [α]D25 −14.3 (c 1.05, CHCl3); IR (neat) 3024, 1734 cm−1; 1H NMR (200 MHz, CDCl3) δ 7.29 (m, 12 H), 7.17 (d, J = 7.1 Hz, 2H), 5.14 (s, 2H), 4.24 (m, 2H), 3.78 (d, J = 12.7 Hz, 1H), 3.63 (m, 1H), 3.55 (d, J = 12.7 Hz, 1H), 3.09 (m, 2H), 2.67 (dt, J = 13.2, 4.4 Hz, 1H), 2.58 (dt, J = 13.2, 4.4 Hz, 1H); 13C NMR (50 MHz, CDCl3) δ 170.9, 156.2, 137.4, 136.6, 129.5, 129.2, 129.0, 128.2, 128.0, 127.6, 127.4, 126.2, 72.4, 68.5, 66.3, 64.9, 46.7, 36.8; MSI m/z: 281 (M+). Anal. Calcd for C25H25NO3 (387.47): C, 77.49; H, 6.50, N, 3.61. Found: C, 77.01; H, 6.16, N, 3.72.
(S)-4-Benzyl-3-((1-benzyl-1H-indol-3-yl)methyl)morpholin-2-one (2j). [α]D25 −17.34 (c 1.0, CHCl3); IR (neat) 3022, 1728 cm-1; 1H NMR (200 MHz, CDCl3) δ 8.16 (d, J = 7.8 Hz, 1H), 7.65 (d, J = 7.8 Hz, 1H), 7.24 (m, 12H), 7.01 (s, 1H), 5.06 (s, 2H), 4.69 (m, 1H), 3.84 (m, 1H), 3.82 (d, J = 13.2 Hz, 1H), 3.64 (d, J = 13.2 Hz, 1H), 3.55 (t, J = 6.8 Hz, 1H), 3.22 (dd, J = 13.2, 4.4 Hz, 1H), 2.81 (dd, J = 13.2, 4.4 Hz, 1H), 2.46 (m, 2H); 13C NMR (50 MHz, CDCl3) δ 169.6, 137.0, 136.3, 135.9, 128.0, 127.8, 127.6, 127.5, 127.2, 126.3, 125.7, 120.9, 118.6, 118.4, 108.6, 66.3, 65.2, 58.5, 49.0, 45.9, 26.8; MSI m/z: 410 (M+). Anal. Calcd for C27H26N2O2 (410.51): C, 79.00; H, 6.38; N, 6.82. Found: C, 78.61; H, 6.16; N, 7.02.
ACKNOWLEDGEMENTS
Gorakh S. Yellol thanks to CSIR, India and NSC, Taiwan for a research fellowship.
References
1. (a) D. Basavaiah and K. Aravindu, Org. Lett., 2007, 9, 2453; CrossRef (b) T. Ishikawa, T. Miyahara, M. Asakura, S. Higuchi, Y. Miyauchi, and S. Saito, Org. Lett., 2005, 7, 1211; CrossRef (c) L. Hong, W. Sun, C. Liu, L. Wang, and R. Wang, Chem. Eur. J., 2010, 16, 746; CrossRef (d) S. A. Neogi Roy and D. Naskar, J. Comb. Chem., 2010, 12, 75; CrossRef (e) S. Ryabukhin, A. Plaskon, D. Volochnyuk, A. Shivanyuk, and A. Tolmachev, J. Org. Chem., 2007, 72, 7417. CrossRef
2. (a) S. Minakata, Acc. Chem. Res., 2009, 42, 1172; CrossRef (b) F. Portela-Cubillo, J. Scott, and J. Walton, J. Org. Chem., 2008, 73, 5558; CrossRef (c) J. Han, B. Xu, and G. Hammond, J. Am. Chem. Soc., 2010, 132, 916; CrossRef (d) T. Driver, Angew. Chem. Int. Ed., 2009, 48, 7974; CrossRef (e) I. Bonnaventure and A. Charette, Tetrahedron, 2009, 65, 4968. CrossRef
3. (a) H. Blaser, Chem. Rev., 1992, 92, 935; CrossRef (b) S. Hanessian, Pure Appl. Chem., 1993, 65, 1189; CrossRef (c) M. Falorni, A. Porcheddu, and G. Giacomelli, Recent Research Developments in Synthetic Organic Chemistry, 1998, 1, 225.
4. (a) R. Williams and J. Hendrix, Chem. Rev., 1992, 92, 889; CrossRef (b) R. Williams, Advances in Asymmetric Synthesis, Vol. 1, ed. by A. Hassner, JAI: London, 1995, p. 45; (c) R. Duthaler, Tetrahedron, 1994, 50, 1539. CrossRef
5. (a) L. Harwood, S. Mountford, and R. Yan, Pept. Sci., 2009, 15, 1; CrossRef (b) D. Aldous, E. Hamelin, L. Harwood, and S. Thurairatnam, Synlett, 2001, 12, 1841; CrossRef (c) L. Harwood and I. Lilley, Synlett, 1996, 10, 1010. CrossRef
6. (a) K. Brands, J. Payack, J. Rosen, T. Nelson, A. Candelario, M. Huffman, M. Zhao, J. Li, B. Craig, Z. Song, D. Tschaen, K. Hansen, P. Devine, P. Pye, K. Rossen, P. Dormer, R. Reamer, C. Welch, D. Mathre, N. Tsou, J. McNamara, and P. Reider, J. Am. Chem. Soc., 2003, 125, 2129; CrossRef (b) W. Kazmierski, E. Furfine, A. Spaltenstein, and L. Wright, Bioorg. Med. Chem. Lett., 2006, 16, 5226; CrossRef (c) A. Dahlgren, P. Johansson, I. Kvarnstrom, D. Musil, I. Nilsson, and B. Samuelsson, Bioorg. Med. Chem., 2002, 10, 1829; CrossRef (d) G. Viti, R. Nannicini, R. Ricci, V. Pestellini, L. Abelli, and M. Furio, Eur. J. Med. Chem., 1994, 29, 401. CrossRef
7. (a) I. Ku, S. Cho, M. Doddareddy, M. Jang, G. Keum, J. Lee, B. Chung, Y. Kim, H. Rhim, and S. Kang, Bioorg. Med. Chem. Lett., 2006, 16, 5244; CrossRef (b) G. Keum, Y. Kim, S. Kang, H. Lim, M. Jang, and I. Ku, Repub. Korea, 2007, 19.
8. S. Balani, S. Pitzenberger, M. Schwartz, H. Ramjit, and W. Thompson, Drug Metab. Dispos., 1995, 23, 185.
9. J. Bartroli, E. Turmo, M. Alguer, E. Boncompte, M. Vericat, J. Garcia-Rafanell, and J. Forn, J. Med. Chem., 1995, 38, 3918. CrossRef
10. B. Park, D. Son, W. Choi, G. Takeoka, S. Han, T. Kim, and S. Lee, Phytother. Res., 2008, 22, 1195. CrossRef
11. L. Wang, H. Huang, Z, Ye, Y. Wu, and X. Wang, Synth. Commun., 2006, 36, 2627. CrossRef
12. N. Kolla, C. Elati, M. Arunagiri, S. Gangula, P. Vankawala, Y. Anjaneyulu, A. Bhattacharya, S. Venkatraman, and V. Mathad, Org. Process Res. Dev., 2007, 11, 455. CrossRef
13. A. Anslow, G. Cox, and L. Harwood, Chem. Heterocycl. Comp., 1995, 31, 1222. CrossRef
14. C. Elati, N. Kolla, S. Gangula, A. Naredla, P. Vankawala, M. Avinigiri, S. Chalamala, V. Sundaram, V. Mathad, A. Bhattacharya, and R. Bandichhor, Tetrahedron Lett., 2007, 48, 8001. CrossRef
15. W. Lee, B. Berridge, L. Ross, and L. Goodman, J. Med. Chem., 1963, 6, 567. CrossRef
16. P. Vankawala, R. Elati, N. Kolla, S. Chlamala, and S. Gangula, WO Patent 2007044829, 2007.
17. M. E. Wood, S. Bissiriou, C. Lowe, and K. Windeatt, Org. Biomol. Chem., 2008, 6, 3048. CrossRef
18. Y. Kim, E. Choi, G. Keum, S. Kang, D. Lee, H. Koh, and Y. Kim. Org. Lett., 2001, 26, 4149. CrossRef
19. (a) C. Kashima and K. Harada, J. Chem. Soc., Perkin Trans.1, 1988, 1521; CrossRef (b) C. Kashima and K. Harada, J. Org. Chem., 1989, 54, 789. CrossRef
20. S. Karmarkar and D. Mohapatra, Synlett, 2001, 8, 1326 CrossRef