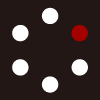
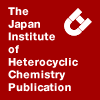
HETEROCYCLES
An International Journal for Reviews and Communications in Heterocyclic ChemistryWeb Edition ISSN: 1881-0942
Published online by The Japan Institute of Heterocyclic Chemistry
e-Journal
Full Text HTML
Received, 31st August, 2010, Accepted, 21st October, 2010, Published online, 2nd November, 2010.
DOI: 10.3987/COM-10-S(E)119
■ Asymmetric Intramolecular Aldol Reaction Mediated by (S)-N-Substituted-N-(2-pyrrolidinylmethyl)amine to Prepare Wieland-Miescher Ketone
Yuichi Akahane, Kohei Inomata,* and Yasuyuki Endo
Tohoku Pharmaceutical University, 4-4-1 Komatsushima, Aoba-ku, Sendai 981-8558, Japan
Abstract
New or known N-substituted-N-(2-pyrrolidinylmethyl)amine deriva- tives bearing a variety of alkyl and aryl substituents were easily prepared from N-Boc-proline or N’-Boc-N-(2-pyrrolidinylmethyl)amine. The enantioselectivity of the intramolecular asymmetric aldol reaction mediated by a combination of the amine derivative and TFA to prepare Wieland-Miescher ketone was examined. During the examination, optimal amount of TFA in the reaction was identified.Wieland-Miescher ketone (2), which was prepared by L-proline-mediated asymmetric intramolecular aldol reaction of the trione (1), has been a highly useful synthon in total syntheses of a variety of natural products.1,2 This asymmetric aldol reaction has become known as the Hajos-Parrish-Eder-Sauer-Wiechert (HPESW) reaction,3 and has been widely recognized to involve an enamine-based mechanism. In the reaction, a hydrogen bond between an oxygen atom on the cyclohexane and a carboxylic acid in L-proline has played a very important role to stabilize a transition state to achieve a highly enantioselective process.4-6
In the area of organocatalysis,7 (S)-2-(pyrrolidinylmethyl)pyrrolidine (4) has been employed as a new catalyst to achieve highly efficient asymmetric reactions, including intra- and intermolecular aldol reaction.8 Amine-Brønsted acid catalysis has also been utilized in asymmetric Diels-Alder reaction and 1,4-addition reaction to construct new chiral centers.9,10 However, to our knowledge, there have been few attempts to use a combination of chiral amine and Brønsted acid in the HPESW reaction.11
Recently, we have reported that a combination of (S)-2-(pyrrolidinylmethyl)pyrrolidine (4) and TFA successfully mediated the HPESW reaction to afford (R)-Wieland-Miescher ketone [(R)-2] accompanied with 81% ee.12 The process was characterized by an inversion of enantioselectivity when compared with the similar reaction mediated by L-Proline. Also, the reaction using 4 without a Brønsted acid afforded (S)-2 with lower enantioselectivity (Scheme 1). We have also established HPESW reaction utilizing N-benzyl-N-(2-pyrrolidinylmethyl)amine derivatives in the presence of TFA.13 In the study, we have observed that steric hindrance around N-benzyl moiety was played an important role to achieve higher enantioselectivity. From these aspects, chiral amines bearing various N-substituents have been still required to find more effective mediators. We report herein alternative chiral amine mediators in the HPESW reaction. Specifically, we attempted to use known and new N-(2-pyrrolidinylmethyl)amine (5) containing a variety of substituents such as alkyl, cycloalkyl, branched alkyl and arylalkyl (Scheme 1).
Among the amines (5) shown in scheme 1, 5a was prepared from N-cbz-L-Proline according to the known method reported by Iseki et al.14 Amines (5b8, 5c15) were prepared from N-Boc-L-Proline (6) respectively in the similar manner reported by Mukaiyama et al.15 Thus, DCC-mediated coupling reaction with 6 and a corresponding amine, and a following deprotection of Boc-moiety in the presence of TFA yielded amide (7). Lithium aluminium hydride (LAH) reduction of amide group afforded 5b and 5c respectively. The other ones (5d,15 5e, 5f16 and 5g) were easily prepared by a simple reductive alkylation of N’-Boc-N-(2-pyrrolidinylmethyl)amine (8)13 with corresponding carbonyl compounds (9), and a following acidic deprotection of Boc moiety (Scheme 2).
We first examined to determine the optimal amount of TFA in the HPESW reaction mediated by a chiral amine. Thus, the effect of TFA quantities on the yield and ee of 2 in the HPSEW reaction, mediated by 4, was examined in detail. All reactions were carried out with 1.0 equiv of 4 in DMSO at rt in the presence of TFA over the range of 0 to 170 mol% (Table 1 and Figure 1). Although the yield of 2 revealed almost same level around 50-65% in all cases, the absolute configuration of 2 was inverted from S to R at the range of 40-50 mol% of TFA. Higher ee was observed upon increasing the amount of TFA, and the parameter reached to a plateau over 100 mol% of TFA. In the presence of more than 100 mol% of TFA, longer time was required to complete the reaction. From these results, the optimal amount of TFA to achieve a high ee was in the range from 100 mol% to 170 mol%. Since the highest ee value was observed in entry 9, 150 mol% of TFA was selected for further application.
Next, the aldol reactions of 1 using a stoichiometric amount of 5 in the presence of 1.5 equiv. of TFA to yield (R)-2 were carried out. The results were compiled in Table 2. All of the reactions mediated by 5 afforded (R)-2 in moderate yield. The reaction proceeded slowly in the case of 5a bearing a simple methyl substituent and the reaction was not completed even after 48 h. Cycloalkyl-substituted derivatives such as 5c and 5e were not also very effective and (R)-2 was obtained in 47% and 53% yield respectively (entries 3 and 5). However, 5b and 5d bearing a tertiary or a secondary branched alkyl substituent respectively improved the yield of (R)-2 (entries 2 and 4). These results suggested us that steric hindrance on N-substituents in 5 obviously affected the reactivity but not greatly improved the enatioselectivity. In comparison with entries 6 and 7, the reaction mediated by N-(9-fluorenyl)methyl derivative (5g) revealed slightly higher ee than the case of diphenylmethyl one (5f). This result suggested that a planarity and a conformationally restriction of two benzene rings might be very important role to the enatioselectivity. In the previous report, we described that the ammonium moiety, which was produced from an amino substituent and a Brønsted acid, stabilized a transition state by hydrogen bonds between a mediator and a substrate.12 Since the present mediators bearing various N-substituents revealed the slightly lower ee than the case of previous N-benzyl derivatives,13 the stabilization of a transition state was due to the circumstance around the ammonium moiety. Thus, the mediators substituted by a bulky group directly such as 5b-5g may inhibit the formation of strong hydrogen bonds to stabilize a favor transition state. These aspects suggested us that N-substituents such as a benzyl group having both a moderate steric hindrance and a moderate planarity were a very important structure to achieve higher ee in the HPESW reaction.
In conclusion, we have established a procedure to prepare N-(2-pyrrolidinylmethyl)amine derivatives (5) bearing a variety of alkyl and aryl substituents. We also have established a new chiral route to provide (R)-Wieland-Miescher ketone [(R)-2] by using the combination of synthetic 5 and TFA. Furthermore, we have determined the optimal amount of TFA in HPESW reaction mediated by a chiral amine. These results may enable the creation of efficient organocatalysts for a wide variety of asymmetric reactions. Further work on a detail of the reaction mechanism and the development of a more efficient catalyst for the reactions is currently in progress.
EXPERIMENTAL
Melting points are uncorrected. IR spectra were recorded on a JASCO-FT-IR-5000 spectrometer. 1H NMR spectra and 13C NMR spectra were recorded on a JEOL-AX-400 (1H 400 MHz, 13C 100 MHz) spectrometer and calibrated using trimethysilane as the internal standard. Mass spectra were recorded on a JEOL-DX-303 or a JEOL-JMS-MS700 spectrometer. Enantiomeric excesses were determined on a Waters-HPLC 600 instrument equipped with a chiral stationary phase column. Optical rotations were measured with a JASCO-DIP-370 digital polarimeter.
(S)-N-t-Butyl-N-(2-pyrrolidinylmethyl)amine (5b)
To a stirred solution of N-Boc-L-Proline (6) 5.0 g (23.3 mmol) in CH2Cl2 (50 mL) were added DCC 4.9 g (23.8 mmol) and 1-hydroxybenzotriazole (HOBt) 3.2 g (23.8 mmol) at rt. After stirring at the same temperature for 30 min, t-butylamine 1.7 g (23.3 mmol) was added and the mixture was further stirred at rt for 24 h. The mixture was filtered through a Celite pad and the filtrate was washed with saturated aqueous NaHCO3 and brine. After drying (Na2SO4), the solvent was removed under reduced pressure to afford crude product. To the stirred solution of the crude product in CH2Cl2 (70 mL) was added TFA 17.7 mL (233 mmol) at rt and the mixture was stirred at the same temperature for 10 h. The mixture was extracted with H2O. The combined aqueous layer was basified by 10% aqueous NaOH and was extracted with CH2Cl2. The combined organic layer was dried (Na2SO4) and the solvent was removed under reduced pressure to afford crude 7. To a stirred suspension of LAH 1.3 g (35 mmol) in THF (70 mL) was added a solution of 7 in THF (20 mL) at 0 oC. The mixture was further heated under reflux for 24 h. 10% aqueous NaOH (14 mL) was carefully added to the mixture at 0 oC and the mixture was stirred at rt for 2 h. The mixture was filtered through a Celite pad and the filtrate was evaporated under reduced pressure. The residue was chromatographed (CHCl3/MeOH/NH4OH =15/1/0.1) to afford 5b 2.1 g (58% from 6) as a yellow oil.
[α]D27 +11.8 (c 1.00, CHCl3), [lit.,8a [α]D25 +12.0 (c 1.98, CHCl3)]; IR (film) ν cm-1 3429, 2980, 1407; 1H-NMR (400 MHz, CDCl3) δ 1.09 (9H, s), 1.29-1.37 (1H, m), 1.65-1.81 (4H, m), 1.84-1.92 (1H ,m), 2.47 (1H, dd, J = 8.4 Hz, 11.2 Hz), 2.55 (1H, dd, J = 4.8 Hz, 11.2 Hz), 2.84-2.94 (2H, m), 3.11-3.17 (1H, m); 13C-NMR (100 MHz, CDCl3) δ 25.5, 28.9, 29.7, 46.3, 47.7, 49.9, 59.0; EIMS (m/z) 156 (M+), 70 (100%); HRMS calcd. for C9H20N2 156.1626, found 156.1623.
(S)-N-Cyclohexyl-N-(2-pyrrolidinylmethyl)amine (5c)
In the similar manner described for 5b, 5c was obtained by the use of cyclohexylamine instead of t-butylamine.
Yield 59% (yellow oil); [α]D28 +9.8 (c 1.00, EtOH), [lit.,15 [α]D24 +10.3 (c 1.01, EtOH)]; IR (film) ν cm-1 3401, 2931, 2856, 1408; 1H- NMR (400 MHz, CDCl3) δ 1.03-1.34 (6H, m), 1.60-1.63 (1H, m), 1.67-1.78 (6H, m), 1.84-1.91 (3H, m), 2.37-2.43 (1H, m), 2.49 (1H, dd, J = 8.8 Hz, 11.6 Hz), 2.66 (1H, dd, J = 4.8 Hz, 11.2 Hz), 2.86-2.92 (2H, m), 3.19 (1H, ddd, J = 4.8 Hz, 7.4 Hz, 15.6 Hz); 13C-NMR (100 MHz, CDCl3) δ 25.0, 25.6, 29.7, 33.4, 33.4, 46.3, 52.0, 57.0, 58.5; EIMS (m/z) 182 (M+), 70 (100%); HRMS calcd. for C11H22N2 182.1783, found 182.1786.
(S)-N-Isopropyl-N-(2-pyrrolidinylmethyl)amine (5d)
To a stirred solution of 813 3.5 g (17.5 mmol) in acetone (30 mL) at rt. After stirring at the same temperature for 8 h, the solvent was removed under reduced pressure. The residue was dissolved to MeOH (30 mL) and NaBH4 333 mg (8.75 mmol) was added to the mixture as a small portion over 10 min at 0 oC. After stirring at 0 oC for 7 h, the solvent was removed under reduced pressure. The residue was dissolved to AcOEt and the mixture was washed with saturated aqueous NaHCO3 and brine. The organic layer was dried (Na2SO4) and the solvent was removed under reduced pressure. The residue was dissolved to CH2Cl2 (70 mL) and TFA 13 mL (175 mmol) was added to the mixture at rt. After stirring at the same temperature for 15 h, the mixture was extracted with H2O. The combined aqueous layer was basified by 10% aqueous NaOH and the mixture was extracted with CH2Cl2. The combined organic layer was dried (Na2SO4) and the solvent was removed under reduced pressure. The residue was chromatographed (CHCl3/MeOH/NH4OH =10/1/0.1) to afford 5d 1.6 g (64% from 8) as a yellow oil.
[α]D28 +16.0 (c 1.00, MeOH), [lit.,15 [α]D26 +16.5 (c 1.07, EtOH)]; IR (film) ν cm-1 3401, 2977, 1411; 1H-NMR (400 MHz, CDCl3) δ 1.05 (3H, d, J = 4.3 Hz), 1.06 (3H, d, J = 4.3 Hz), 1.28-1.37 (1H, m), 1.68-1.76 (4H, m), 1.84-1.91 (1H, m), 2.46 (1H, dd, J = 4.8 Hz, 11.4 Hz), 2.63 (1H, dd, J = 4.8 Hz, 11.4 Hz), 2.75-2.81 (1H, m), 2.88-2.92 (2H, m), 3.20 (1H, ddd, J = 4.8 Hz, 7.4 Hz, 15.9 Hz); 13C-NMR (100 MHz, CDCl3) δ 23.0, 25.7, 29.8, 46.4, 48.8, 52.9, 58.5; EIMS (m/z) 142 (M+), 70 (100%); HRMS calcd. for C8H18N2 142.1470, found 142.1437.
(S)-N-Cyclohexylmethyl-N-(2-pyrrolidinylmethyl)amine (5e)
To a stirred solution of 8 3.5 g, (17.5 mmol) in dichloromethane (35 mL) was added cyclohexanecarboxaldehyde 2.3 mL (19.3 mmol) at rt. After stirring at the same temperature for 9 h, the solvent was removed under reduced pressure. The residue was dissolved to MeOH (35 mL) and NaBH4 333 mg (8.75 mmol) was added to the mixture as a small portion over 10 min at 0 oC. After stirring at 0 oC for 7 h, the solvent was removed under reduced pressure. The residue was dissolved to AcOEt and the mixture was washed with saturated aqueous NaHCO3 and brine. The organic layer was dried (Na2SO4) and the solvent was removed under reduced pressure. The residue was dissolved to CH2Cl2 (70 mL) and TFA 13 mL (175 mmol) was added to the mixture at rt. After stirring at the same temperature for 15 h, the mixture was extracted with H2O. The combined aqueous layer was basified by 10% aqueous NaOH and the mixture was extracted with CH2Cl2. The combined organic layer was dried (Na2SO4) and the solvent was removed under reduced pressure. The residue was chromatographed (CHCl3/MeOH/NH4OH =10/1/0.1) to afford 5e 1.6 g (47% from 8) as a yellow oil.
[α]D28 +12.1 (c 1.00, MeOH); IR (film) ν cm-1 3400, 2924, 2851, 1409; 1H-NMR (400 MHz, CDCl3) δ 0.85-0.94 (2H, m), 1.10-1.36 (4H, m), 1.40-1.49 (1H, m), 1.65-1.78 (9H, m), 1.83-1.91 (1H, m), 2.44 (2H, dd, J = 2.4 Hz, 6.8 Hz), 2.49 (1H, dd, J = 8.2 Hz, 11.6 Hz), 2.59 (1H, dd, J = 4.8 Hz, 11.8Hz), 2.85-2.95 (2H, m), 3.18-3.25 (1H, m); 13C-NMR (100 MHz, CDCl3) δ 25.6, 26.0, 26.6, 29.7, 31.3, 37.8, 46.4, 55.3, 56.9, 58.2; EIMS (m/z) 196 (M+), 70 (100%); HRMS calcd. for C12H24N2 196.1939, found 196.1916.
(S)-N-Diphenylmethyl-N-(2-pyrrolidinylmethyl)amine (5f)
In the smilar manner described for 5e, 5f was obtained by the use of benzophenone instead of cyclohexanecarboxaldehyde.
Yield 42% (yellow oil); [α]D20 +19.2 (c 0.84, MeOH), [lit.,16 [α]D25 +20.0 (c 1.0, MeOH)]; IR (film) ν cm-1 3283, 2956, 2869, 1452, 1405; 1H-NMR (400 MHz, CDCl3) δ 1.30-1.37 (1H, m), 1.65-1.92 (3H, m), 2.48 (1H, dd, J = 8.4 Hz, 11.6 Hz), 2.59 (1H, dd, J = 4.8 Hz, 11.8 Hz), 2.87-2.91 (2H, m), 3.27 (1H, ddd, J = 4.8 Hz, 7.2 Hz, 11.7 Hz), 4.81 (1H, s), 7.19-7.21 (2H, m), 7.29 (4H, dt, J = 2.0 Hz, 7.2 Hz), 7.38-7.41 (4H, m); 13C-NMR (100 MHz, CDCl3) δ 25.4, 29.5, 46.2, 52.8, 58.5, 67.6, 126.9, 127.2, 128.4, 144.0; EIMS (m/z) 266 (M+), 70 (100%); HRMS calcd. for C18H22N2 266.1783, found 266.1787.
(S)-N-(9-Fluorenyl)-N-(2-pyrrolidinylmethyl)amine (5g)
In the smilar manner described for 5e, 5g was obtained by the use of 9-fluorenone instead of cyclohexanecarboxaldehyde.
Yield 48% (yellow oil); [α]D21 -8.6 (c 1.00, CHCl3); IR (film) ν cm-1 3285, 2926, 2871, 144.7, 1403; 1H-NMR (400 MHz, CDCl3) δ 1.17-1.26 (1H, m), 1.61-1.68 (2H, m), 1.72-1.80 (1H, m), 2.08 (2H, brs), 2.22 (1H, dd, J = 8.0 Hz, 11.8 Hz), 2.34 (1H, dd, J = 4.8 Hz, 11.8 Hz), 2.82-2.86 (2H, m), 3.09-3.15 (1H, m), 4.96 (1H, s), 7.33-7.39 (4H, m), 7.59-7.62 (2H, m), 7.69-7.71 (2H, m); 13C-NMR (100 MHz, CDCl3) δ 25.4, 29.3, 46.2, 48.6, 58.9, 63.4, 119.8, 124.7, 127.2, 127.8, 140.6, 145.6; EIMS (m/z) 264 (M+), 70 (100%); HRMS calcd. for C18H20N2 264.1626, found 264.1624.
The effect of quaintities of TFA in the HPESW reaction
To a stirred solution of the trione (1) 147 mg (0.75 mmol) in DMSO (1.5 mL) was added the amine (4) 121 µL (0.75 mmol) and TFA 86 µL (1.1 mmol) at rt. The reaction mixture was stirred at rt for 48 h, then diluted with ethyl acetate (AcOEt), washed with saturated aqueous NaHCO3 and brine, and dried (MgSO4). The solvent was removed under reduced pressure. The crude product was chromatographed on silica gel (AcOEt/CH2Cl2/hexane = 1/1/4) to afford (R)-2 70 mg (52%) as a pale yellow oil. The optical purity was determined to be 81% ee by HPLC with a chiral stationary phase. HPLC conditions: Chiralcel OD, 2-propanol/hexane = 1/10 (v/v), flow rate 1.0 mL/min, detected at 254 nm, tR = 14.6 min for (S)-2, 15.7 min for (R)-2. The results in Table 1 were obtained by the same manner changing the amount of TFA in the range from 0 to 170 mol%.
Typical procedure of HPESW reaction mediated by chiral amine (5)
To a stirred solution of 5d 203 mg (0.70 mmol) and TFA 86 μL (1.13 mmol) in DMSO (1.5 mL) was added 1 147 mg (0.75 mmol) at rt. After stirring at the same temperature for 9 h, the mixture was diluted with AcOEt and the mixture was washed with saturated aqueous NaHCO3 and brine. The organic layer was dried (Na2SO4) and the solvent was removed under reduced pressure. The residue was chromatographed (AcOEt/CH2Cl2/hexane = 1/1/4) to afford (R)-2 84 mg (67%) as a pale yellow oil. The optical purity was determined to be 70% ee by the same manner described above.
[α]D25 -69.1: 70% ee (c 1.00, benzene), [lit., [(S)-2],3l [α]D25 +100 (c 1.40, benzene)]; IR (film) ν cm-1 1714, 1668; 1H-NMR (400 MHz, CDCl3) δ 1.46 (3H, s), 1.67-1.78 (1H, m), 2.10-2.19 (3H, m), 2.44-2.53 (4H, m), 2.68-2.77 (2H, m), 5.87 (1H, s); 13C-NMR (100 MHz, CDCl3) δ 22.8, 23.2, 29.6, 31.7, 33.5, 37.6, 50.5, 125.8, 165.8, 198.2, 211.0; EIMS (m/z) 178 (M+, 100%); HRMS calcd. for C11H14O2 178.0992, found 178.0988.
References
1. (a) P. Wieland and K. Miescher, Helv. Chim. Acta, 1950, 33, 2215; CrossRef (b) S. Ramachandran and M. S. Newman, Organic Syntheses, 1961, 41, 38; (c) J. Gutzwiller, P. Buchschacher, and A. Fürst, Synthesis, 1977, 167; CrossRef (d) R. Uma, S. Swaminathan, and K. Rajagopalan, Tetrahedron Lett., 1984, 25, 5825; CrossRef (e) G. Ottolina, G. Gonzalo, G. Carrea, and B. Danieli, Adv. Synth. Catal., 2005, 347, 1035; CrossRef (f) Y. Kasai, K. Shimanuki, S. Kuwahara, M. Watanabe, and N. Harada, Chirality, 2006, 18, 177. CrossRef
2. Recent applications of 2: (a) N. Shah and T. S. Scanlan, Bioorg. Med. Chem. Lett., 2004, 14, 5199; CrossRef (b) S. Díaz, A González, B. Bradshaw, J. Cuesta, and J. Bonjoch, J. Org. Chem., 2005, 70, 3749; CrossRef (c) H. Liu, D. R. Siegel, and S. J. Danishefsky, Org. Lett., 2006, 8, 423; CrossRef (d) A. B. Smith III, L. Kürti, A. H. Davulcu, and Y. S. Cho, Org. Process Res. Dev., 2007, 11, 19; CrossRef (e) K. P. Kaliappan and V. Ravikumar, Org. Lett., 2007, 9, 2417; CrossRef (f) B. Bradshaw, G. Etxebarria-Jardí, and J. Bonjoch, Org. Biomol. Chem., 2008, 6, 772; CrossRef (g) K. Ma, C. Zhang, M. Liu, Y. Chu, L. Zhou, C. Hu, and D. Ye, Tetrahedron Lett., 2010, 51, 1870. CrossRef
3. (a) Z. G. Hajos and D. R. Parrish, German Patent DE 2102623, 1971; (b) U. Eder, G. Sauer and R. Wiechert, German Patent DE 2014757, 1971; (c) U. Eder, G. Sauer, and R. Wiechert, Angew. Chem., 1971, 83, 492; CrossRef (d) Z. G. Hajos and D. R. Parrish J. Org. Chem., 1973, 38, 3239; CrossRef (e) Z. G. Hajos and D. R. Parrish, J. Org. Chem., 1974, 39, 1615; CrossRef (f) R. A. Micheli, Z. G. Hajos, N. Cohen, D. R. Parrish, L. A. Portland, W. Sciamanna, M. A. Scott, and P. A. Wehri, J. Org. Chem., 1975, 40, 675; CrossRef (g) P. Buchschacher and A. Fuerst, Organic Syntheses, 1985, 63, 37; (h) Z. G. Hajos and D. R. Parrish, Organic Syntheses, Collect. Vol. VII, p. 363, Wiley: New York, 1990; (i) N. Harada, T. Sugioka, H. Uda, and T. Kuriki, Synthesis, 1990, 53; CrossRef (j) D. Rajagopal, K. Rajagopalan, and S. Swaminathan, Tetrahedron: Asymmetry, 1996, 7, 2189; CrossRef (k) T. Bui and C. F. Barbas III, Tetrahedron Lett., 2000, 41, 6951; CrossRef (l) D. Rajagopal, R. Narayanan, and S. Swaminathan, Tetrahedron Lett., 2001, 42, 4887. CrossRef
4. (a) G. Zhong, T. Hoffmann, R. A. Lerner, S. Danishefsky, and C. F. Barbas III, J. Am. Chem. Soc., 1997, 119, 8131; CrossRef (b) K. Fuhshuku, N. Funa, T. Akeboshi, H. Ohta, H. Hosomi, S. Ohba, and T. Sugai, J. Org. Chem., 2000, 65, 129; CrossRef (c) H. Hioki, T. Hashimoto, and M. Kodama, Tetrahedron: Asymmetry, 2000, 11, 829; CrossRef (d) A. Gassama, J. d’Angelo, C. Cavé, J. Mahuteau, and C. Riche, Eur. J. Org. Chem., 2000, 3165; CrossRef (e) S. G. Davies, R. L. Sheppard, A. D. Smith, and J. E. Thomson, Chem. Commun., 2005, 3802; CrossRef (f) M. Hosseini, N. Stiasni, V. Barbieri, and C. O. Kappe, J. Org. Chem., 2007, 72, 1417; CrossRef (g) S. Sulzer-Mossé, M. Laars, K. Kriis, T. Kanger, and A. Alexakis, Synthesis, 2007, 1729; CrossRef (h) B. Bradshaw, G. Etxebarria-Jardi, J. Bonjoch, S. F. Viózquez, G. Guillena, and C. Nájera, Adv. Synth. Catal., 2009, 351, 2842; CrossRef (i) Á. L. Fuentes de Arriba, L. Simón, C. Raposo, V. Alcázar, and J. R. Morán, Tetrahedron, 2009, 65, 4841; CrossRef (j) K. Mori, T. Katoh, T. Suzuki, T. Noji, M. Yamanaka, and T. Akiyama, Angew. Chem. Int. Ed., 2009, 48, 9652. CrossRef
5. (a) K. L. Brown, L. Damm, J. D. Dunitz, A. Eschenmoser, R. Hobi, and C. Kratky, Helv. Chim. Acta, 1978, 61, 3108; CrossRef (b) C. Agami and H. Sevestre, J. Chem. Soc., Chem. Commun., 1984, 1385; CrossRef (c) C. Agami, C. Puchot, and H. Sevestre, Tetrahedron Lett., 1986, 27, 1501; CrossRef (d) C. Agami and C. Puchot, J. Mol. Catal., 1986, 38, 341; CrossRef (e) C. Agami, N. Platzer, and H. Sevestre, Bull. Soc. Chim. Fr., 1987, 358; (f) C. Agami, Bull. Soc. Chim. Fr., 1988, 499; (g) N. Gathaergood, Aust. J. Chem., 2002, 55, 615. CrossRef
6. (a) S. Bahmanyar and K. N. Houk, J. Am. Chem. Soc., 2001, 123, 11273; CrossRef (b) S. Bahmanyar and K. N. Houk, J. Am. Chem. Soc., 2001, 123, 12911; CrossRef (c) L. Hoang, S. Bahmanyar, K. N. Houk, and B. List, J. Am. Chem. Soc., 2003, 125, 16; CrossRef (d) S. Bahmanyar, K. N. Houk, H. J. Martin, and B. List, J. Am. Chem. Soc., 2003, 125, 2475; CrossRef (e) B. List, L. Hoang, and H. J. Martin, Proc. Nat. Acad. Sci. U. S. A., 2004, 101, 5839; CrossRef (f) B. List, Acc. Chem. Res., 2004, 37, 548; CrossRef (g) C. Allemann, R. Gordillo, F. R. Clemente, P. H.-Y. Cheong, and K. N. Houk, Acc. Chem. Res., 2004, 37, 558; CrossRef (h) P. H.-Y. Cheong and K. N. Houk, Synthesis, 2005, 1533. CrossRef
7. For reviews: (a) B. List, Tetrahedron, 2002, 58, 5573; CrossRef (b) W. Notz, F. Tanaka, and C. F. Barbas III, Acc. Chem. Res., 2004, 37, 580; CrossRef (c) P. I. Dalko and L. Moisan, Angew. Chem. Int. Ed., 2004, 43, 5138; CrossRef (d) A. Berkessel and H. Gröger, Asymmetric Organocatalysis, Wiley-VCH: Weinheim, 2005; CrossRef (e) B. List, Chem. Commun., 2006, 819; CrossRef (f) H. Pellissier, Tetrahedron, 2007, 63, 9267; CrossRef (g) G. Guillena, C. Nájera, and D. J. Ramón, Tetrahedron: Asymmetry, 2007, 18, 2249; CrossRef (h) A. Dondoni and A. Massi, Angew. Chem. Int. Ed., 2008, 47, 4638; CrossRef (i) P. Melchiorre, M. Marigo, A. Carlone, and G. Bartoli, Angew. Chem. Int. Ed., 2008, 47, 6138. CrossRef
8. (a) S. Saito, M. Nakadai, and H. Yamamoto, Synlett, 2001, 1245; CrossRef (b) M. Nakadai, S. Saito, and H. Yamamoto, Tetrahedron, 2002, 58, 8167; CrossRef (c) S. Saito and H. Yamamoto, Acc. Chem. Res., 2004, 37, 570; CrossRef (d) S. Saito, N. Momiyama, and H. Yamamoto, J. Synth. Org. Chem. Jpn., 2008, 66, 774.
9. Some selected examples: (a) K. Ishihara and K. Nakano, J. Am. Chem. Soc., 2005, 127, 10504; CrossRef and 13079; CrossRef (b) A. Sakakura, K. Suzuki, K. Nakano, and K. Ishihara, Org. Lett., 2006, 8, 2229; CrossRef (c) A. Sakakura, K. Suzuki, and K. Ishihara, Adv. Synth. Catal., 2006, 348, 2457; CrossRef (d) H. Sundén, R. Rios, Y. Xu, L. Eriksson, and A. Córdova, Adv. Synth. Catal., 2007, 349, 2549. CrossRef
10. Some selected examples: (a) S. Mossé, M. Laars, M. Kriis, T. Kanger, and A. Alexakis, Org. Lett., 2006, 8, 2559; CrossRef (b) S. Mossé and A. Alexakis, Chem. Commun., 2007, 3123; CrossRef (c) H. Chen, Y. Wang, S. Wei, and J. Sun, Tetrahedron: Asymmetry, 2007, 18, 1308; CrossRef (d) D. A. Alonso, S. Kitagaki, N. Usumi, and C. F. Barbas III, Angew. Chem. Int. Ed., 2008, 47, 4588. CrossRef
11. (a) T. Kanger, K. Kriis, M. Laars, T. Kailas, A-M. Müürisepp, T. Pehk, and M. Lopp, J. Org. Chem., 2007, 72, 5168; CrossRef (b) E. Lacoste, E. Vaique, M. Berlande, I. Pianet, J.-M. Vincent, and Y. Landais, Eur. J. Org. Chem., 2007, 167; CrossRef (c) M. Laars, K. Kriis, T. Kailas, A.-M. Müürisepp, T. Pehk, T. Kanger, and M. Lopp, Tetrahedron: Asymmetry, 2008, 19, 641. CrossRef
12. Y. Akahane, N. Inage, T. Nagamine, K. Inomata, and Y. Endo, Heterocycles, 2007, 74, 637. CrossRef
13. Y. Akahane, K. Inomata, and Y. Endo, Heterocycles, 2009, 77, 1065. CrossRef
14. K. Iseki, Y. Kuroki, M. Takahashi, S. Kishimoto, and Y. Kobayashi, Tetrahedron, 1997, 53, 3513. CrossRef
15. M. Asami, H. Ohno, S. Kobayashi, and T. Mukaiyama, Bull. Chem. Soc. Jpn., 1978, 51, 1869. CrossRef
16. J. L. Olivares-Romero and E. Juaristi, Tetrahedron, 2008, 64, 9992. CrossRef