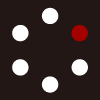
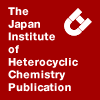
HETEROCYCLES
An International Journal for Reviews and Communications in Heterocyclic ChemistryWeb Edition ISSN: 1881-0942
Published online by The Japan Institute of Heterocyclic Chemistry
e-Journal
Full Text HTML
Received, 21st June, 2010, Accepted, 19th August, 2010, Published online, 20th August, 2010.
DOI: 10.3987/COM-10-S(E)64
■ Preparation and Electrochemical Properties of Phthalocyaninato Titanium (IV) and Porphyrinato Titanium (IV) Benzenedichalcogenolates
Takeshi Kimura,* Kaori Amano, Arata Yamamoto, and Toshiharu Namauo
Center for Instrumental Analysis, Iwate University, 4-3-5 Ueda, Morioka, Iwate 020-8551, Japan
Abstract
The reaction of phthalocyaninato titanium (IV) oxides with benzenedithiol or benzenediselenol produced the corresponding complexes with titanium-sulfur or titanium-selenium bonds. Tetraphenylporphyrinato titanium (IV) benzenedichalcogenolates were obtained by the similar procedure. Their optical and electrochemical properties were determined by UV-vis spectroscopy and cyclic voltammetry.INTRODUCTION
Phthalocyanines (Pcs) and porphyrins have attracted much attention because of their potential applications in electrochromic displays, field-effect transistors, nonlinear optical materials, and dye-sensitized solar cells.1-3 The optical and electrochemical characteristics of these compounds could be based on their large and flat π-conjugated ring systems. The structures of the complexes depend on the central metal atom and its oxidation state and ligands. It was reported that phthalocyaninato titanium (IV) complexes could have two ligands in one face of the Pc-plane.4 As related compounds with bidentate ligands, Hanack reported phthalocyaninato titanium(IV) complexes with titanium-oxygen or titanium-sulfur bonds,5 while Kobayashi synthesized a mutually perpendicular phthalocyanine pentamer and related derivatives.6 In addition, we reported a double-decker phthalocyanine complex linked with four titanium-sulfur bonds.7,8 Alternatively, porphyrinato titanium(IV) complexes with titanium-oxygen or titanium-sulfur bonds were described.9 To construct new types of phthalocyanine and porphyrin complexes with an axial ligand connected by titanium-sulfur or titanium-selenium bonds, octa-n-butoxyphthalocyaninato titanium(IV) oxide (4), tetra-t-butylphthalocyaninato titanium(IV) oxide (5),5b octamethylthiophthalocyaninato titanium(IV) oxide (6), and tetraphenylporphyrinato titanium(IV) oxide (10) were reacted with 3,6-diethyl-4,5-dibromobenzene-1,2-dithiol (3a) or 3,6-diethyl-4,5-dibromobenzene-1,2-diselenol (3b). This paper reports the preparation and electrochemical properties of octabutoxyphthalocyaninato titanium(IV) benzenedithiolate (7a) and related compounds.
RESULT AND DISCUSSION
1,2-Bis(2-cyanoethylchalcogeno)-4,5-dibromo-3,6-diethylbenzenes (2a,b) were prepared from 5,6-dibromo-4,7-diethylbenzo[1,2,3]trichalcogenoles (1a,b) in 96% and 70% yields, respectively, upon treatment with NaBH4 and then 2-cyanoethyl bromide (Scheme 1).8 To generate benzenedithiol (3a) as a bidentate sulfur ligand, 2a was reacted with cesium hydroxide in THF/MeOH under Ar (Scheme 1). The reaction mixture was acidified with concentrated hydrochloric acid to give 3a. Benzenediselenol (3b) could be generated from 2b by the similar procedure.
The prepared solution of 3a was transferred to a reactor containing 4, and the reaction mixture was stirred at room temperature for 24 h (Scheme 2). After workup and purification with silica gel column chromatography and Bio-beads column chromatography, phthalocyanine (7a) with benzenedithiolate as the axial ligand was obtained in 67% yield. Compound (6) was prepared from the corresponding metal-free derivative on treatment with titanium(IV) tetrabutoxide by the procedure reported previously.7 The treatment of 6 with 3a produced phthalocyanine (9a) in 51% yield. Tetra-t-butyl derivative (8a) was also prepared from 5 by the similar process in 58% yield.
In the 1H NMR spectrum of 7a, the signals of the butoxy groups appeared at δ = 1.20 (t), 1.81 (sext), 2.18 (quint), 4.63 (dt), and 4.67 (dt) ppm. These chemical shifts are located at a slightly upper field compared with those of 4: δ = 1.25 (t), 1.86 (sext), 2.21 (quint), 4.64 (dt), and 4.68 (dt) ppm. Signals for the ethyl group of benzenedithiolate were observed at δ = -0.02 (t) and 1.48 (bq) ppm, which are a higher magnetic field than those of 2a: δ = 1.29 (t) and 3.28 (q) ppm. These results could indicate that the benzene ring lies on the phthalocyanine plane and is strongly affected in the magnetic shielding range of the macrocycle. The 13C NMR spectrum of 7a showed four signals for the phthalocyanine skeleton, six signals for the ethyl and butoxy groups, and three signals for the coordinating benzene ring. Apparently, the phthalocyanine skeleton of 7a is a C4v symmetric structure in the NMR time scale at 25 °C, although benzenedithiolate coordinates to the central titanium atom.
Compound (9a) showed the signals for the ethyl and methylthio groups of the phthalocyanine core at δ = 1.60 (t), 2.72 (s), and 4.68-5.00 (m) ppm in the 1H NMR spectrum. The signals for the ethyl group of benzenedithiolate were observed at δ = 0.23 (t) and 1.81 (q) ppm. The 13C NMR spectrum showed twelve signals for the compound, suggesting that 9a has also a C4v symmetric structure in the NMR time scale. The FABMS spectra of 7a and 9a exhibited the corresponding molecular ion peaks at m/z = 1488.4187 [M+] for 7a and m/z = 1504.13 [M+] for 9a.
A similar treatment of 6 with 3b produced phthalocyanine (9b) with benzenediselenolate in 33% yield. The 1H NMR and 13C NMR spectra of 9b were similar to those of 9a, while the signals for the ethyl group of 9b were observed at a slightly lower magnetic field than were those of 9a. This result could be related to the difference of the bond distance between the titanium-sulfur and titanium-selenium bonds. The 77Se NMR spectrum showed the signal at δ = 448.5 ppm, which is an extremely higher magnetic field than that of the bis(cyclopentadienyl)benzodiselenatitanole derivatives.10 The FABMS spectrum of 9b exhibited the molecular ion peak at m/z = 1600.13 [M+].
The solution of benzenedithiol was then added to 10 and the reaction gave 11a with benzenedithiolate as the axial ligand in 89% yield (Scheme 3). Compound (11a) seems more unstable than 7a. A similar treatment of 10 with 3b produced 11b with benzenediselenolate in 91% yield. In the 1H NMR spectrum, 11a showed the signals of the phenyl groups and the porphyrin core at δ = 7.74-7.85 (m, Ph), 8.16 (m, Ph), 8.25 (m, Ph), and 9.05 (s, β) ppm. These chemical shifts are situated at a slightly upper field compared with those of 10. The signals for the ethyl group of 11a were observed at δ = 0.20 (t) and 1.64 (q) ppm. The 13C NMR spectrum of 11a showed fourteen signals because two ortho and two meta carbon atoms of the phenyl group are unsymmetric, respectively. Although the 1H and 13C NMR of 11b are similar to those of 11a, the signals for the ethyl group were observed at δ = 0.27 (t) and 1.80 (q) ppm in 1H NMR. The FABMS spectrum
of 11a showed the corresponding molecular ion peak at m/z = 1012.0384 [M+], while that of 11b exhibited the molecular ion peak at m/z = 1108.0 [MH+].
In the UV-vis spectra of 7a and 8a measured in chloroform, the Q band absorptions were observed at λmax = 702 nm (ε = 120000) for 7a and λmax = 698 nm (ε = 110000) for 8a (Figure 1). These absorptions were wider bandwidths compared with that of 4 (λmax = 702 nm, ε = 220000) and 5 (λmax = 701 nm, ε = 150000), respectively. Compounds (9a) and (9b) showed the Q band absorptions at λmax = 764 (ε = 110000) and λmax = 766 nm (ε = 62000), respectively, which were wider bandwidths and slightly longer wavelengths than that of 6 (λmax = 760 nm, ε = 140000). The absorption of the selenium derivative was observed at a slightly red-shifted region compared with the sulfur derivative. In contrast, 11a and 11b exhibited two split Soret band absorptions λ max = 399 nm (ε = 66000) and 432 nm (ε = 110000) for 11a and λmax = 396 nm (ε = 74000) and 427 nm (ε = 78000) for 11b, although the Soret band absorption of 10 was observed at λmax = 424 nm (log ε = 330000).
The electrochemical properties of 7a, 8a, 9a, 9b, 11a, and 11b were examined by cyclic voltammetry using Ag/AgNO3 as a reference electrode (measured in dichloromethane, scan rate: 200 mV/s). The results are summarized in Table 1. The redox potentials of 7a were observed at E1/2 = –1.82, –1.34, and –1.03 V for the reduction and E1/2 = 0.59 V for the oxidation (Figure 2). Compound (8a) showed three reversible couples for reduction potentials (E1/2 = –1.84, –1.27, and –0.89 V) and one quasi-reversible (E1/2 = 0.82 V) and one reversible couples (E1/2 = 1.15 V) for oxidation potentials. The cyclic voltammogram of 9a contained an irreversible oxidation (Ep = 0.87 V) and three quasi-reversible reduction potentials (E1/2 = –1.73, –1.06, and –0.76 V). Compound (9b) exhibited an irreversible oxidation potential at Ep = 0.85 V and three quasi-reversible reduction potentials at E1/2 = –1.78, –1.04, and –0.75 V. The oxidation potential of 11a was found at E1/2 = 1.04 V (reversible) and Ep = 1.37 V (irreversible), while one reversible couple (E1/2 = –1.30 V) was observed for the reduction potential. Compound (11b) showed one irreversible oxidation peak (Ep = 0.96 V) and one reversible and one irreversible reduction peak (E1/2 = –1.29 V and Ep = –1.05 V). It appeared that the first oxidation and the first reduction potential of 7a, 9a, 9b, 11a, and 11b show slight anode shifts compared with those of the corresponding titanium oxides.
CONCLUSION
The reactions of phthalocyaninato titanium(IV) oxides (4), (5), and (6) and tetraphenylporphyrinato titanium(IV) oxide (10) with benzenedithiol or benzenediselenol produced corresponding complexes with titanium-sulfur or titanium-selenium bonds. Compounds (7a), (9a), (9b), (11a), and (11b) showed the C4v symmetric structure in the NMR time scale. Their optical and electrochemical properties were determined by UV-vis spectroscopy and cyclic voltammetry.
EXPERIMENTAL
General
NMR spectra were measured with a Bruker AVANCE-500 III spectrometer and a Bruker AC400 spectrometer at 25 °C. For measurements of the NMR spectra, tetramethylsilane (1H and 13C) and dimethyl selenide (77Se) were used as reference compounds. Mass spectra were obtained using a JEOL JMS-700 mass spectrometer. UV-vis spectra were recorded with a JASCO Ubest V-570 spectrometer. A Hokuto Denko Co. Model HAB-151 apparatus was employed for measuring oxidation potentials. Bio-beads (SX-1) for column chromatography was purchased from Nippon Bio-Rad Laboratories.
Oxidation potentials
All measurements were performed by cyclic voltammetry, using Ag/AgNO3 (0.01 mol/L) as a reference electrode (scan rate: 200 mV/s). A solution of n-Bu4NClO4 in CH2Cl2 (0.1 mol/L) was used as an electrolyte. The oxidation potential of ferrocene was observed at E1/2 = 0.09 V by the apparatus without any correction.
Materials
Compounds (1a), (1b), (2a), and (5) were prepared using a method described previously.8
1,2-Bis(2-cyanoethylseleno)-4,5-dibromodiethylbenzene (2b)
To a solution of 1b (1.604 g, 3 mmol) and K2CO3 (831 mg, 6 mmol) in THF (20 mL)/MeOH (10 mL), NaBH4 (347 mg, 9.1 mmol) was added under Ar, and the solution was stirred for 30 min. 3-Bromopropionitrile (0.97 mL, 12 mmol) was added to the solution. After stirring for 6 h and acidification with HCl, the solvent was evaporated. H2O was added and the product was extracted with CHCl3. The solvent was evaporated and the residue was purified by column chromatography (Wakogel C-400, n-hexane : CHCl3 = 1 : 1) to produce 2b in 70% yield (1.182 g); colorless crystals; mp 83-86 °C; 1H NMR (400 MHz, CDCl3) δ = 1.18 (t, J = 7.4 Hz, 6H), 2.71 (t, J = 7.2 Hz, 4H), 3.20 (t, J = 7.2 Hz, 4H), 3.43 (q, J = 7.4 Hz, 4H) ppm; 13C NMR (101 MHz, CDCl3) δ = 14.3, 18.7, 26.2, 36.2, 118.3, 130.3, 137.7, 149.5 ppm; 77Se NMR (76 MHz, CDCl3) δ = 325.2 ppm; IR (KBr) 2244 cm-1 (CN); HRMS Calcd for C16H1879Br2N280Se2, 555.8167. Found (m/z) 555.8167 (M+).
Generation of 3,6-diethyl-4,5-dibromobenzene-1,2-dithiol (3a)
Compound (2a) (287.5 mg, 0.62 mmol) and CsOH (955.5 mg, 6.37 mmol) were placed in a glass reactor under Ar. THF (4 mL) and MeOH (4 mL) were added to the reactor and the solution was stirred for 2 h. HCl (0.6 mL) in MeOH (2.0 mL) was added to the solution to generate the corresponding dithiol (3a).
Octa-n-butoxyphthalocyaninato titanium(IV) benzenedithiolate (7a)
The solution of 3a, prepared as described above, was transferred by a syringe to the reactor containing 4 (195.7 mg, 0.17 mmol) under Ar. The solution was stirred for 24 h and the solvent was evaporated. The residue was purified by column chromatography (Wakogel C-300HG, CHCl3) and (Bio-beads, CHCl3) to produce 7a in 67% yield (168.7 mg); Green powder; 1H NMR (500 MHz, CDCl3) δ = -0.02 (t, J = 6.4 Hz, 6H, CH3), 1.20 (t, J = 7.4 Hz, 24H, CH3), 1.48 (br-q, J = 6.4 Hz, CH2), 1.81 (sext, J = 7.4 Hz, 16H, CH2), 2.18 (quint, J = 7.4 Hz, 16H, CH2), 4.64 (dt, J = 8.0, 7.4 Hz, 8H, CH2), 4.68 (dt, J = 8.0, 7.4 Hz, 8H, CH2), 8.77 (bs, 8H, Ar-H); 13C NMR (126 MHz, CDCl3) δ = 11.4, 14.1, 19.6, 29.7, 31.6, 69.5, 105.4, 124.3, 130.7, 135.5, 148.5, 150.8, 153.0; HRFAB MS: calcd for C74H90Br2N8O8S2Ti = 1488.4169, found: m/z = 1488.4187 (M+).
Tetra-t-butylphthalocyaninato titanium(IV) benzenedithiolate (8a)
58%; Green powder; 1H NMR (500 MHz, CDCl3) δ = 0.01-0.17 (m, 6H, CH3), 1.50-1.67 (m, 4H, CH2), 1.77-1.93 (m, 36H, t-Bu), 8.35-8.43 (m, 4H, Ar-H), 9.32-9.62 (m, 8H, Ar-H); HRFAB MS: calcd for C58H5879Br2N8O8S246Ti = 1134.2119, found: m/z = 1134.2086 (M+).
Octakis(methylthio)octaethylphthalocyaninato titanium(IV) benzenedithiolate (9a)
51%; Dark green powder; mp > 300 °C; 1H NMR (400 MHz, CDCl3) δ = 0.23 (t, J = 7.2 Hz, 6H, CH3), 1.60 (t, J = 7.2 Hz, 24H, CH3), 1.81 (q, J = 7.2 Hz, 4H, CH2), 2.72 (s, 24H, SCH3), 4.68-5.00 (m, 16H, CH2) ppm; 13C NMR (101 MHz, CDCl3) δ = 11.5, 16.4, 22.7, 25.4, 30.0, 124.9, 132.9, 136.4, 146.7, 146.8, 147.9, 151.4 ppm; UV-vis (CHCl3) λmax= 763.5 nm; FAB MS (m/z) 1504.13 [M+]; Anal. Calcd for C66H74Br2N8S10Ti: C, 52.58; H, 4.95; N, 7.43. Found: C, 52.36; H, 5.09; N, 6.53.
Octakis(methylthio)octaethylphthalocyaninato titanium(IV) benzenediselenolate (9b)
33%; Dark green powder: mp 225 °C; 1H NMR (400 MHz, CDCl3) δ = 0.29 (t, J = 7.4 Hz, 6H, CH3), 1.59 (t, J = 7.2 Hz, 24H, CH3), 1.93 (q, 4H, CH2), 2.74 (s, 24H, SCH3), 4.60-4.99 (m, 16H, CH2) ppm; 13C NMR (101 MHz, CDCl3) δ = 11.7, 16.4, 22.7, 25.4, 29.7, 125.2, 132.9, 139.5, 146.6, 146.8, 148.5, 151.2 ppm; 77Se NMR (76 MHz, CDCl3) δ = 448.5 ppm; FAB MS (m/z) 1600.13 [M+].
Tetraphenylporphyrinato titanium(IV) benzenedithiolate (11a)
89%; Black green powder: 1H NMR (400 MHz CDCl3) δ = 0.20 (t, J = 7.4 Hz, 6H, CH3), 1.64 (q, J = 7.4
Hz, 4H, CH2), 7.74-7.85 (m, 12H, m,p-PhH), 8.16 (m, 4H, o-PhH), 8.25 (m, 4H, o-PhH), 9.05 (s, 8H, β); 13C NMR (126 MHz CDCl3) δ = 11.4, 29.6, 123.7, 125.5, 127.1, 127.2, 128.3, 132.6, 133.1, 134.7, 141.2, 148.8, 150.3; HRFAB MS: Calcd for C54H38Br2N4S248Ti = 1012.0384. Found: m/z = 1012.0384 (M+); Anal. Calcd for C54H38Br2N4S2Ti: C, 63.92; H, 3.77; N, 5.52%. Found: C, 63.46; H, 4.02; N, 5.15%.
Tetraphenylporphyrinato titanium(IV) benzenediselenolate (11b)
91%; Black green powder: 1H NMR (400 MHz CDCl3) δ = 0.27 (t, J = 7.3 Hz, 6H, CH3), 1.80 (q, J = 7.3 Hz, 4H, CH2), 7.44-7.86 (m, 12H, m,p-PhH), 8.15 (d, J = 6.72 Hz, 4H, o-PhH), 8.29 (d, J = 6.72 Hz, 4H, o-PhH), 9.05 (s, 8H, β); 13C NMR (126 MHz CDCl3) δ = 11.6, 29.7, 124.2, 125.4, 127.1, 127.2, 128.3, 132.6, 133.0, 134.8, 138.0, 141.1, 149.3, 150.3; FABMS m/z = 1108.0 [MH+].
References
1. ‘The Porphyrin Handbook’, Vols. 15-20, ed. by K. M. Kadish, K. M. Smith, and R. Guilard, Academic Press, San Diego, 2003; Phthalocyanines: Properties and Applications’, Vols. 1-4, ed. by C. C. Leznoff and A. B. P. Lever, VCH, New York, 1989-1996.
2. a) D. Wöhrle, O. Suvorova, R. Gerdes, O. Bartels, L. Lapok, N. Baziakina, S. Makarov, and A. Slodek, J. Porphyrins Phthalocyanines, 2004, 8, 1020; CrossRef b) G. de la Torre, P. Vázquez, F. Agulló-López, and T. Torres, Chem. Rev., 2004, 104, 3723; CrossRef c) G. de la Torre, C. G. Claessens, and T. Torres, Chem. Commun., 2007, 2000. CrossRef
3. a) A. Morandeira, I. López-Duarte, M. V. Martínez-Díaz, B. O’Regan, C. Shuttle, N. A. Haji-Zainulabidin, T. Torres, E. Palomares, and J. R. Durrant, J. Am. Chem. Soc., 2007, 129, 9250; CrossRef b) L. Matín-Gomis, K. Ohkubo, F. Fernández-Lázaro, S. Fukuzumi, and Á. Sastre-Santos, Org. Lett., 2007, 9, 3441; CrossRef c) B. C. O’Regan, I. López-Duarte, M. V. Martínez-Díaz, A. Forneli, J. Albero, A. Moranderia, E. Palomares, T. Torres, and J. R. Durrant, J. Am. Chem. Soc., 2008, 130, 2906. CrossRef
4. V. L. Goedken, G. Dessy, C. Ercolani, V. Fares, and L. Gastaldi, Inorg. Chem., 1985, 24, 991. CrossRef
5. a) M. Barthel and M. Hanack, J. Porphyrins Phthalocyanines, 2000, 4, 635; CrossRef b) M. Barthel, D. Dini, S. Vagin, and M. Hanack, Eur. J. Org. Chem., 2002, 3756. CrossRef
6. a) N. Kobayashi and A. Muranaka, Chem. Commun., 2000, 1855; CrossRef b) A. Muranaka, M. Okuda, N. Kobayashi, K. Somers, and A. Ceulemans, J. Am. Chem. Soc., 2004, 126, 4596; CrossRef c) A. Muranaka, K. Yoshida, T. Shoji, N. Moriichi, S. Masumoto, T. Kanda, Y. Ohtake, and N. Kobayashi, Org. Lett., 2006, 8, 2447; CrossRef A. Muranaka, K. Yoshida, Y. Akagi, H. Naka, M. Uchiyama, Y. Kondo, N. Kobayashi, Tetrahedron Lett., 2008, 49, 5084. CrossRef
7. T. Kimura, J. Kumasaka, and T. Namauo, Eur J. Org. Chem., 2008, 5079. CrossRef
8. a) T. Kimura, A. Yomogita, T. Matsutani, T. Suzuki, I. Tanaka, Y. Kawai, Y. Takaguchi, T. Wakahara, and T. Akasaka, J. Org. Chem., 2004, 69, 4716; CrossRef b) T. Kimura, N. Kanota, K. Matsui, I. Tanaka, T. Tsuboi, Y. Takaguchi, A. Yomogita, T. Wakahara, S. Kuwahara, F. Nagatsugi, and T. Akasaka, Inorg. Chem., 2008, 47, 3577. CrossRef
9. A. Deronzier and J. M. Latour, Nou. J. Chim., 1984, 8, 393.
10. N. Nagahora, S. Ogawa, S. Yoshimura, Y. Kawai, and R. Sato, Bull. Chem. Soc. Jpn., 2003, 76, 1043. CrossRef