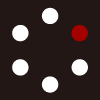
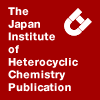
HETEROCYCLES
An International Journal for Reviews and Communications in Heterocyclic ChemistryWeb Edition ISSN: 1881-0942
Published online by The Japan Institute of Heterocyclic Chemistry
e-Journal
Full Text HTML
Received, 1st September, 2010, Accepted, 18th October, 2010, Published online, 1st November, 2010.
DOI: 10.3987/COM-10-S(E)120
■ Kinetic Resolution of the Racemic 1-(Aryloxazol-2-yl)carbinols with Achiral Carboxylic Acids by Asymmetric Esterification: A New Method for the Preparation of Chiral 1,2-Amino Alcohols
Kenya Nakata, Keisuke Ono, and Isamu Shiina*
Department of Applied Chemistry, Faculty of Science, Tokyo University of Science, Kagurazaka 1-3, Shinjuku-ku, Tokyo 162-8601, Japan
Abstract
An efficient kinetic resolution of racemic 1-(aryloxazol-2-yl)carbinols with achiral carboxylic acids using pivalic anhydride in the presence of (R)-benzotetramisole ((R)-BTM) is reported. It was determined that the naphth[1,2-d]oxazole moiety at the C-1 position in the alcohols is a suitable structure to attain a high selectivity. An application to produce optically pure 1,2-amino alcohols was developed by the kinetic resolution of the racemic 1-(aryloxazol-2-yl)carbinols followed by a two-step cleavage of the aryloxazole part to form the corresponding amino group. Transition states that provide the desired (R)-esters from (R)-1-(benzoxazol-2-yl)ethan-1-ol or the undesired (S)-esters from (S)-1-(benzoxazol-2-yl)ethan-1-ol are disclosed by DFT calculations. Another transition state that affords the desired (R)-ester from (R)-1-(naphth[1,2-d]oxazol-2-yl)ethan-1-ol included in the racemic mixture is also estimated, and the structural features of these transition states are discussed.Optically active 1,2-amino alcohol units are widely distributed in natural products, such as alkaloids, azamacrolides, and amino sugar antibiotics. Moreover, several kinds of optically active 1,2-amino alcohols are frequently utilized as the starting materials for the synthesis of pharmaceutical drugs and the production of chiral ligands in the field of asymmetric catalysis. There are currently several methods for preparing chiral 1,2-amino alcohols derived from chiral or achiral epoxides by the oxirane-opening ammonolysis.1 However, in these syntheses, the basic carbon skeletons of the 1,2-amino alcohols must be constructed before the oxirane-opening reaction, so that there are several structural limitations in the preparation of the chiral or achiral epoxides. Thus we decided to attempt the development of an alternative and facile protocol for the synthesis of optically active 1,2-amino alcohols by the kinetic resolution of the racemic compounds.
We have reported the first asymmetric esterification of the racemic carboxylic acids2 and the kinetic resolution of the racemic secondary alcohols with achiral carboxylic acids3,4 via the in situ formation of the mixed-anhydride using carboxylic anhydrides as coupling reagents and the chiral acyl-transfer catalysts, such as (S)-tetramisole and (R)-benzotetramisole ((R)-BTM), which were popularized by Birman et al.5 Furthermore, it was independently reported that the benzoxazole moiety could be transformed into the substituted 2-aminophenol by reductive cleavage with LiAlH4,6 and the oxidative treatment of the resulting secondary 2-aminophenol derivatives using ceric ammonium nitrate (CAN) could provide the corresponding primary amine;7 therefore, we planned to obtain the chiral primary amines from the optically active benzoxazole compounds via the successive transformation of the aryloxazole groups. We now report the novel kinetic resolution of racemic 1-(aryloxazol-2-yl)carbinols, followed by the effective conversion of the naphthoxazole moieties into the desired primary amino groups to provide the chiral 1,2-amino alcohols (Scheme 1).
First, we examined the kinetic resolution of the racemic 1-(aryloxazol-2-yl)ethan-1-ols (±)-1a–d, which include the basic structure of the 1-(benzoxazol-2-yl)carbinols, with 3-phenylpropanoic acid using pivalic anhydride and a catalytic amount of (R)-BTM in order to determine the suitable structure of the aryloxazole moiety (Table 1). When the kinetic resolution of (±)-1-(benzoxazol-2-yl)ethan-1-ol (1a), which was prepared from the racemic lactic acid by the condensation with 2-aminophenol according to the literature method,8 was carried out under the standard reaction conditions established in the previous paper,3b a relatively fair selectivity factor9 (s = 22) was obtained (Entry 1). Other derivatives were also synthesized from the racemic lactic acid, and the resulting 1-(naphthoxazol-2-yl)ethan-1-ols (1b–d) were successively examined under the same reaction conditions. As shown in Entries 2–4, the fused-structure of the naphthalene ring together with the oxazole ring significantly affected the stereoselectivities of the kinetic resolution of the racemic alcohols (±)-1b–d. When the substrates include the naphth[2,1-d]oxazole and naphth[2,3-d]oxazole moieties at the C-1 position (1b and 1c), the selectivity factors were nearly equal to that of the reaction of 1a (Entries 2 and 3, cf. Entry 1). Fortunately, it was found that the kinetic resolution of 1-(naphth[1,2-d]oxazol-2-yl)ethan-1-ol (1d) afforded the corresponding chiral ester (R)-2d in 45% yield with a high enantiomeric excess (87% ee), and 54% of the starting alcohol (S)-1d was recovered with good enantiomeric excess (73% ee) to attain the highest selectivity (s = 31, Entry 4).
The optimized structure of the oxazole part in substrates was successfully determined as shown above, and so we next examined other racemic 1-(naphth[1,2-d]oxazol-2-yl)carbinols bearing several alkyl substituents at the C-1 position in order to assess the generality of the kinetic resolution (Table 2). As shown by Entries 1–5, all of the kinetic resolutions of (±)-3a–e effectively took place to give the desired esters (R)-4a–e in high yields with good s-values irrespective of the steric hindrance of the alkyl groups in the substrates. It was found that the synthetically important substrates, 3d and 3e, which include the useful functionalities, such as the α-siloxy and allyl groups, were also applicable for the same reaction without any loss of the stereoselectivities (s = 41 and 20, Entries 4 and 5).
Second, we examined the transformation of the naphthoxazole moiety in the products into the corresponding amino groups in order to prepare the chiral 1,2-amino alcohols (Scheme 2). Treatment of ester (R)-4a (86% ee; Table 2, Entry 1) with LiAlH4 in THF afforded 1-amino-2-naphthol (R)-5, and the successive deprotection of the 2-naphthol moiety in the resulting (R)-5 by the oxidative cleavage with CAN furnished the desired 1,2-amino alcohol (R)-6 in 42% yield in 2 steps without any loss of the chirality (Scheme 2, upper transformation). Similarly, alcohol (S)-3a (>99% ee; Table 2, Entry 6) was also subjected to the two-step procedure to generate the 1,2-amino alcohol (S)-6 in 44% yield in 2 steps (Scheme 2, lower transformation). Thus, both enantiomers, (R)-6 and (S)-6, were successfully obtained via the kinetic resolution of the racemic alcohol (±)-3a followed by the transformation of the naphthoxazole moieties into the primary amino groups without any loss of chirality.
We further carried out the transformation of the optically pure alcohol (S)-3a into synthetically useful products, such as O-Bn-amino alcohol (S)-9, N-Boc-O-Bn-amino alcohol (S)-10, and N-Boc-amino alcohol (S)-11 (Scheme 3). First, the hydroxyl group in (S)-3a was protected by the benzyl group to provide (S)-7 in quantitative yield, and the resulting benzyl ether (S)-7 was subjected to the above established two-step effective procedure including the conversion of the naphthoxazole moiety into
the 1-amino-2-naphthol functionality to afford the O-Bn-amino alcohol (S)-9. The hydrogenation of (S)-9 provided the corresponding chiral 1,2-amino alcohol (S)-6 in an overall yield of 83% in four steps from the alcohol (S)-3a. Protection of the O-Bn-amino alcohol (S)-9 was attained by treatment with (Boc)2O and triethylamine to produce the N-Boc-O-Bn-amino alcohol (S)-10 in 71% yield, which was then subsequently deprotected to give the N-Boc-amino alcohol (S)-11 in quantitative yield. It is remarkable that the optically purity was not affected through a series of these transformations.
Finally, determination of the transition states forming the optically active esters from (R)-1-(benzoxazol-2-yl)ethan-1-ol ((R)-1a) and (S)-1-(benzoxazol-2-yl)ethan-1-ol ((S)-1a) with the intermediary zwitterionic species (R)-int, which was generated from acetic anhydride and (R)-BTM, was carried out using the density functional theory (DFT) calculations at the B3LYP/6-31G*//B3LYP/6-31G* level according to the former reports on the kinetic resolution of the racemic compounds.2b,4,10,11 We obtained several transition states for the reaction of the (R)- or (S)-1a with the dihydroimidazolium salt (R)-int, and the three stable structures to form the (R)- or (S)-ester are depicted in Scheme 4. All the 3-dimensional structures of the transition states in Schemes 4 and 5 are shown in Figure 1.
It was found that the nitrogen in the oxazole coordinates to the cationic center in the most stable transition state ts-(R)-1a-A1, which was derived from (R)-1a with the zwitterionic species (R)-int, and the nucleophilic oxygen in the hydroxy approaches to the carbonyl carbon in the activated acetyl group included in ts-(R)-1a-A1. The distance of the forming C–O bond (between the carbonyl carbon of the acid component and the oxygen of hydroxy) is 2.220 Å, and the distance of the cleaved O–H bond (between the oxygen and hydrogen in hydroxy) is 1.328 Å. The calculated bond distances of these elements support the fact that the nucleophilic attack of the alcohol on the carbonyl group and the deprotonation of the hydroxyl group with the carboxylate anion simultaneously proceeded with a concerted reaction mechanism to form the desired chiral (R)-ester.
Another transition state (ts-(R)-1a-A2) is a rotamer of ts-(R)-1a-A1, in which the oxygen in the oxazole coordinates to the cation center of the zwitterionic species (R)-int, and the calculation of the transition structure ts-(R)-1a-A2 suggested that ts-(R)-1a-A2 has a more unstable structure compared to ts-(R)-1a-A1 (ΔErel = 1.84 kcal/mol) because coordination of oxygen to the cation center in ts-(R)-1a-A2 is weaker than that of nitrogen to the cation center in ts-(R)-1a-A1.
On the other hand, the complexation of (S)-1a with (R)-int produced an unstable transition structure ts-(S)-1a-B, that has a higher energy derived from the steric repulsion between the methyl group on the carbinol carbon in (S)-1a and another methyl group of the acetic acid moiety of the dihydroimidazolium salt (R)-int to afford the corresponding (S)-ester. Based on the above theoretical studies of the reaction mechanism, it was concluded that the structure of ts-(R)-1a-A1 is more stable than that of ts-(S)-1a-B (ΔErel = 4.84 kcal/mol); therefore, the desired (R)-ester was predominantly obtained via the rapid consumption of (R)-1a by the kinetic resolution of the racemic alcohol (±)-1a through the favorable transition state ts-(R)-1a-A1.
According to the similar theoretical predictions for the reaction of (R)- or (S)-1d with the zwitterionic species (R)-int generated from the acetic anhydride with (R)-BTM, the corresponding transition structures were also determined as shown in Scheme 5 and Figure 1. It was anticipated that ts-(R)-1d-A1 is more stable than ts-(R)-1d-A2, a rotamer of ts-(R)-1d-A1, because of the strong stabilization effect of the coordination of nitrogen to the cation center in ts-(R)-1d-A1 instead of the oxygen to the cation center in ts-(R)-1d-A2. It was further calculated that the difference in the structural energies between ts-(R)-1d-A1 and ts-(R)-1d-B was 5.38 kcal/mol, which is higher than that between ts-(R)-1a-A1 and ts-(R)-1a-B (cf. 4.84 kcal/mol in Scheme 4), so that the reaction of (±)-1d with the zwitterionic species (R)-int should provide a better selectivity than that by the reaction of (±)-1a with (R)-int. This assumption provides good agreement with the experimental results that shows the high s-value in Entry 4 using (±)-1d in Table 1 (s = 31) although the kinetic resolution of (±)-1a provide a lower selectivity factor as shown in Entry 1 (s = 22).
Typical procedure for the asymmetric esterification of racemic 1-(naphth[1,2-d]oxazol-2-yl)-3- phenylpropan-1-ol ((±)-3a) with 3-phenylpropanoic acid using pivalic anhydride in the presence of (R)-BTM was described (Table 2, Entry 1): To a solution of 3-phenylpropanoic acid (15.1 mg, 0.100 mmol) and pivalic anhydride (24.0 µL, 0.118 mmol) in dichloromethane (1.0 mL) at room temperature were successively added diisopropylethylamine (42.0 µL, 0.241 mmol), (R)-BTM (2.5 mg, 99.1 µmol), and racemic 1-(naphth[1,2-d]oxazol-2-yl)-3-phenylpropan-1-ol ((±)-3a) (60.7 mg, 0.200 mmol). The reaction mixture was stirred for 12 h at the same temperature, and then saturated aqueous NaHCO3 was added to the mixture. The organic layer was separated and the aqueous layer was extracted with ethyl acetate. The combined organic layer was dried over Na2SO4. After filtration of the mixture and evaporation of the solvent, the crude product was purified by preparative thin layer chromatography on silica to afford the corresponding optically active ester (R)-4a (38.8 mg, 45% yield, 86% ee) and the recovered optically active alcohol (S)-3a (33.5 mg, 55% yield, 75% ee) [s = 29.8].
In summary, we have found a new method for the preparation of the optically active 1,2-amino alcohols by the kinetic resolution of the racemic 1-(aryloxazol-2-yl)carbinols with achiral carboxylic
acids using pivalic anhydride and the chiral acyl-transfer catalyst followed by the conversion of the aryloxazole moiety into the primary amino group. Further studies using the present method to provide chiral materials and other applications of this novel protocol are now in progress in our laboratory.
ACKNOWLEDGEMENTS
This study was partially supported by a Research Grant from Toray Science Foundation, and Grants-in-Aid for Scientific Research from the Ministry of Education, Science, Sports and Culture, Japan. The author thanks the Shin-Etsu Chemical Co., Ltd. (Japan), for kindly providing t-butylchlorodimethylsilane as a bulk sample.
References
1. a) G. Bartoli, M. Bosco, A. Carlone, M. Locatelli, P. Melchiorre, and L. Sambri, Org. Lett., 2004, 6, 3973; CrossRef b) D.-M. Lee, J.-C. Lee, N. Jeong, and K.-I. Lee, Tetrahedron: Asymmetry, 2007, 18, 2662; CrossRef c) Y. Kaburagi and Y. Kishi, Tetrahedron Lett., 2007, 48, 8967; CrossRef d) F. W. Lewis, M. C. Eichler, and D. H. Grayson, Synlett, 2009, 1923, and references cited therein. CrossRef
2. a) I. Shiina, K. Nakata, and Y. Onda, Eur. J. Org. Chem., 2008, 5887; CrossRef b) I. Shiina, K. Nakata, K. Ono, Y. Onda, and M. Itagaki, J. Am. Chem. Soc., 2010, 132, 11629. CrossRef
3. Kinetic resolution of racemic benzylic alcohols: a) I. Shiina and K. Nakata, Tetrahedron Lett., 2007, 48, 8314; CrossRef b) I. Shiina, K. Nakata, M. Sugimoto, Y. Onda, T. Iizumi, and K. Ono, Heterocycles, 2009, 77, 801; CrossRef c) K. Nakata and I. Shiina, Heterocycles, 2010, 80, 169. CrossRef
4. Kinetic resolution of racemic 2-hydroxyalkanoates: I. Shiina, K. Nakata, K. Ono, M. Sugimoto, and A. Sekiguchi, Chem. Eur. J., 2010, 16, 167. CrossRef
5. a) V. B. Birman and X. Li, Org. Lett., 2006, 8, 1351; CrossRef b) V. B. Birman and L. Guo, Org. Lett., 2006, 8, 4859. CrossRef
6. a) N. G. Gaylord and D. J. Kay, J. Am. Chem. Soc., 1956, 78, 2167; CrossRef b) A. Raadt, H. Griengl, M. Petsch, P. Plachota, N. Schoo, H. Weber, G. Braunegg, I. Kopper, M. Kreinner, and A. Zeiser, Tetrahedron: Asymmetry, 1996, 7, 491. CrossRef
7. a) D. R. Kronenthal, C. Y. Han, and M. K. Taylor, J. Org. Chem., 1982, 47, 2765; CrossRef b) S. Kobayashi, J. Kobayashi, H. Ishitani, and M. Ueno, Chem. Eur. J., 2002, 8, 4185. CrossRef
8. E. Tauer and K.-H. Grellmann, Chem. Ber., 1990, 123, 1149. CrossRef
9. H. B. Kagan and J. C. Fiaud, Top. Stereochem., 1988, 18, 249. CrossRef
10. See also previous mechanistic studies of acyl-transfer reactions: for DMAP, a) S. Xu, I. Held, B. Kempf, H. Mayr, W. Steglich, and H. Zipse, Chem. Eur. J., 2005, 11, 4751; CrossRef for CF3-PIP, b) X. Li, P. Liu, K. N. Houk, and V. B. Birman, J. Am. Chem. Soc., 2008, 130, 13836. CrossRef
11. All calculations were performed with the program package Spartan '08 1.1.1 of Wavefunction Inc. (http://www.wavefun.com).